Antibacterial potential of synthesized silver nanoparticles from leaf extract of Moringa oleifera
Abstract
Silver nanoparticles (Ag-NPs) are among the most widely used nanoparticles that show a broad spectrum of antibacterial activity. Green synthesis of Ag-NPs from plant extract is the most popular method in vitro. In this study, Ag-NPs were biologically synthesized from the leaf extract of Moringa oleifera and the antimicrobial activity was observed. Furthermore, a comparative assessment of antimicrobial activity of biologically synthesized Ag-NPs and crude plant extract was performed. Initially, 11 pathogenic bacterial strains were used to evaluate the antibacterial potentiality of crude leaf sample. However, the result showed that the crude leaf extract exerted maximum potential against Proteus vulgaris. Additionally, the application of biologically synthesized Ag-NPs was assessed against the same pathogenic strains and observed enhanced antibacterial activities with larger inhibited zones. Thus, our results suggest that the biological synthesis of Ag-NPs significantly enhanced the antibacterial activity of M. oleifera leaf extract against selected pathogenic bacterial strains. M. oleifera could be a potential source of Ag-NPs for successful use as an antibacterial agent in pharmaceutical and cosmetic industries.
INTRODUCTION
Nanoparticles are very commonly used word which represents particles in nano scale size (1–100 nm) with increased surface area. Their structural conformation and organized distribution in solution confer enhancement of their physical and chemical properties which are beneficial for different biological areas. In recent times, silver nanoparticles (Ag-NPs) has gained enormous attention because of its diverse characteristics which are appropriate for various medical and biomedical applications such as catalysis [1], diagnostics [2], antimicrobial development [3] and targeting of drug [4].
Different conventional methods (both physical and chemical) for nanoparticle synthesis have very restricted use due to many limitations, especially for their toxicity, high-energy requirements and an expensive downstream processing. On the contrary, biological synthesis of silver nanoparticles offers several advantages such as fast, high yields and importantly, the cheap downstream processing requirements compared to conventional methods. Thus, different biological methods of nanoparticles synthesis using microorganisms [5, 6], enzymes [7], and plants or plant extracts [8-13] have been suggested as possible ecofriendly alternatives to chemical and physical methods. In the process of nanoparticles synthesis using plant extracts involved chemical reaction with phytocompounds present in the plant extract and silver nitrate (AgNO3) [14].
In the present study, we used leaf of Moringa oleifera Lam. (Moringaceae) plant, locally known as “Drumstick”, for synthesis of green Ag-NPs. The leaves of this plant are prominent for their natural healing properties and are gobbled up in varieties ways. Also, it possess high natural antioxidant properties and antibacterial activity against different gram positive and gram negative pathogenic bacteria [15]. Moreover, it does not require addition of any external stabilizing agents during synthesis of nanoparticles which are really detrimental to the environment [16]. Thus, here we report the biosynthesis of Ag-NPs from M. oleifera leaf extract which are further assessed and compared for antibacterial efficacy.
MATERIALS AND METHODS
Collection of samples, processing and extraction of metabolites
The leaf of M. oleifera were collected from Khulna University campus, Khulna, Bangladesh. All samples were carefully washed and cleaned for surface sterilization. Undesired materials were removed from samples and finally cut into tiny pieces. After proper sun drying, the leaves were firmly grounded and immersed into 50% ethyl acetate (Loba Chemie Pvt Ltd, Mumbai, India). The soaked mixtures were maintained in dark with periodic shaking and stirring for next 7 days. Then the mixtures were filtered and the filtrates were incubated at 45 °C for 30 min in water bath. After that it was transferred to an incubator (at 37 0 C for 72 hours). The crude leaf extract was quantified and stored in 4 0C. This extraction procedure is slightly modified from that of reported by Alinezhad et al. in 2012 [17].
Green synthesis of Ag-NPs
Silver nitrate salt (AgNO3, 1 mM) (Unichem Laboratories Ltd., Mumbai, India) was used for the synthesis of silver nanoparticles. This synthesis procedure was previously reported by Moodley et al., in 2018 [18]. In brief, 5 ml of Silver nitrate solution was mixed with 50 ml of plant extract and incubated into direct sunlight for 30 min. Subsequent change in color to dark brown indicated the formation of nanoparticles in the reaction tubes. To prevent agglomeration of the synthesized nanoparticles the respected tubes were removed from sunlight and kept in dark at room temperature. The synthesized Ag-NPs were further confirmed and quantified using a UV-vis spectrometer (Prolific Instruments, Mumbai, India) at 300-700 nm.
Purification of synthesized Ag-NPs and spectral analysis
The mixture of Ag-NPs was centrifuged at 10,000 rpm for 1 hour at 4 °C using a centrifuge machine (Thermo Fisher Scientific, Massachusetts, USA). The supernatant was discarded and the pellet was washed with distilled water to remove the adulterated plant material. The pellets were again centrifuged at 10,000 rpm for 30 minutes at 4 °C. This wash step was repeated twice to remove water soluble biomolecules such as proteins and cellular metabolites. Finally, the pellets containing the silver nanoparticles were kept at 37 °C for 24 hours to obtain a concentrated mixture of nanoparticles. For further confirmation of Ag-NPs UV-Vis spectral analysis was performed after sunlight exposure of 0, 30 and 90 min. The absorbance bands of the samples were fixed in 300 to 700 nm.
Agar disc diffusion assay
Agar disc diffusion assay was performed to evaluate the antibacterial potential of extracted samples. The assay procedure was previously reported by Biemer in 1973 [19]. Briefly, overnight incubated bacterial subcultures were inoculated in nutrient broth (Himedia Laboratories Pvt. Ltd., Bengaluru, India) and mix properly. Then the broth was flooded into petri dishes containing Muller-Hinton agar media (Himedia Laboratories Pvt. Ltd., Bengaluru, India) and was spread throughout the media gently with a sterile glass spreader. The 5 mm sterile filter paper disc were impregnated with 50 μg of the test stuffs and dried under restrained condition to vaporize residual solvent. Standard ciprofloxacin (30μg/disc) were used as positive control and blank discs were used as negative control. The sample discs, antibiotic discs, negative control discs were gently placed on to inoculated agar plates. Then the plates were transferred to the incubator (at 37⁰C for 24 hours) and kept inversely. After incubation the antibacterial activities were quantified by measuring the diameters of zone of inhibitions. An overall representation of the methodology is plotted in Figure 1 for better understanding.
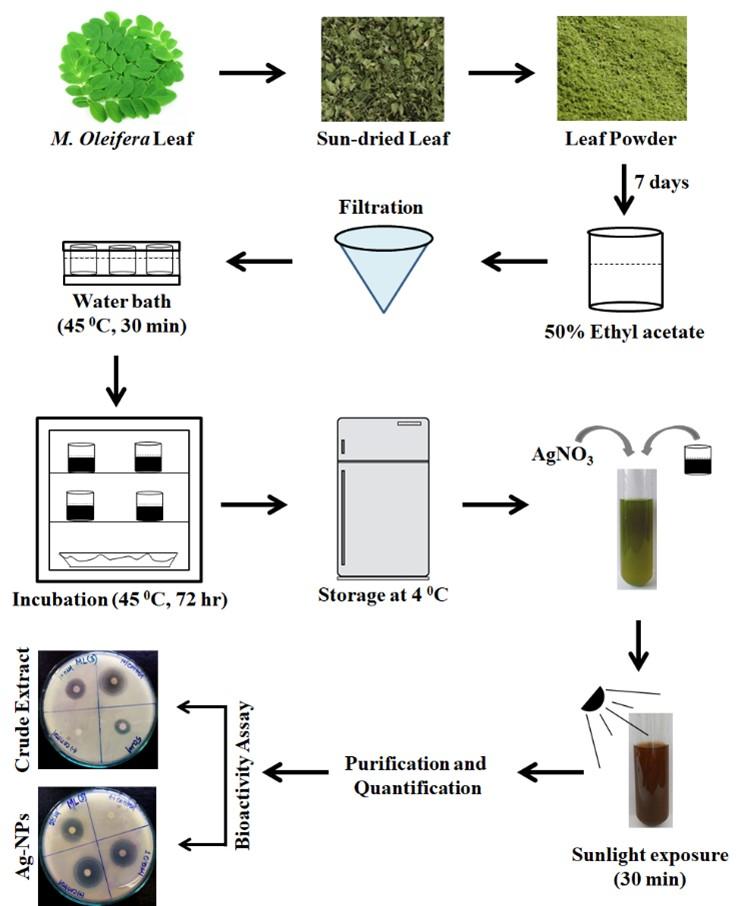
Statistical analysis
All data were analytically scrutinized using SPSS version 21. In the case of inhibited zone measurement ANOVA test was performed at 95% confidence level. Differences were considered significant at the 0.05 level and where possible, means were separated by a Tukey post-hoc test.
RESULTS
Visible color change of crude extract indicating the formation of nanoparticles
The formation of nanoparticles in the crude extract is usually detected by observing the color change from clear (transparent) to dark brown in the presence of sunlight. During this study, the leaf extract changed its color to dark brown after 30 min of exposure in sunlight. Whereas, the control (distilled water and silver nitrate solution) did not show any color difference after sunlight exposure. The color change was easily noticeable and satisfactory to confirm the presence of Ag-NPs in a significant amount as presented in Figure 2A-C.
The UV-vis spectral analysis showed that the absorbance of samples was increased gradually following sunlight exposure. At the beginning, the absorbance of the reaction mixture was found nil/zero as there were no particles formed at that time. The increased absorbance indicates the formation of Ag-NPs with an increased concentration. The surface plasmon resonance of Ag-NPs showed a peak near 440 nm to 450 nm for leaf extract (Figure 2D). The symmetrical shape of the plasmon bands suggesting well-dispersed and uniform-sized nanoparticle synthesis.
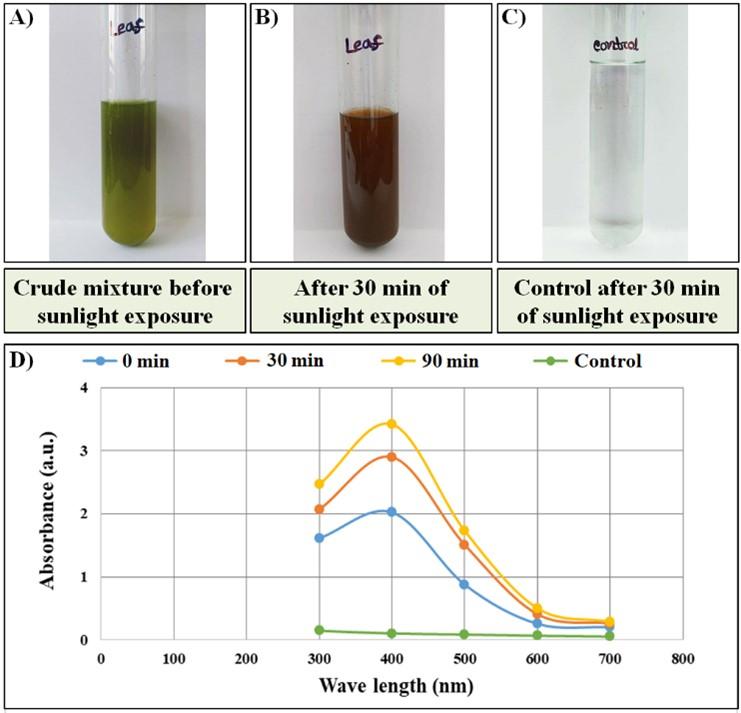
Antibacterial activities of crude extract of M. oleifera
The crude extract from leaves was applied to observe its antibacterial potentials on 11 different pathogenic bacterial strains. Among the 11 strains 3 were gram positive (Staphylococcus aureus, Micrococcus and Mycobacterium) and 8 were gram negative (Escherichia coli, Vibrio cholera, Salmonella typhi, Salmonella paratyphi, Proteus vulgaris, Shigella dysenteriae, Shigella flexneri and Campylobacter) bacteria. However, only 6 strains showed countable susceptibility against crude extract (50 μg/disc). The maximum zone of inhibition (7.93±1.68 mm) was recorded for P. vulgaris against M. oleifera leaf extract. The detailed information of the 6 susceptible strains with their inhibited zone diameters is presented in Table 1.
Table 1. The antibacterial activity of crude extract from M. oleifera leaves.
Antibacterial activities of the synthesized Ag-NPS
To observe the antibacterial activities of the synthesized Ag-NPs agar disc diffusion assay was performed again. The same amount of synthesized Ag-NP (50 μg/disc) was used against all of the six pathogenic strains. The Ag-NPs from leaf extract showed highest antibacterial potential against V. cholera and S. aureus with the zone diameter of 21.67±4.72 mm and 21.67±1.53 mm, respectively. The detailed information is presented in Table 2.
Table 2. The antibacterial activity of synthesized Ag-NPs from M. oleifera leaf extract.
Synthesized Ag-NPs significantly enhanced the antibacterial potential than crude extract
It was observed that the similar dose of either crude extract or synthesized Ag-NP produced different zone diameters. In the all cases the antibacterial activity of Ag-NPs were enhanced compared to that of the crude extract. The highest fold enhancement of antibacterial activity with 50 μg/disc was observed against V. cholera (4.98 fold) when compared with leaf extract. The detailed result is plotted in Figure 3.
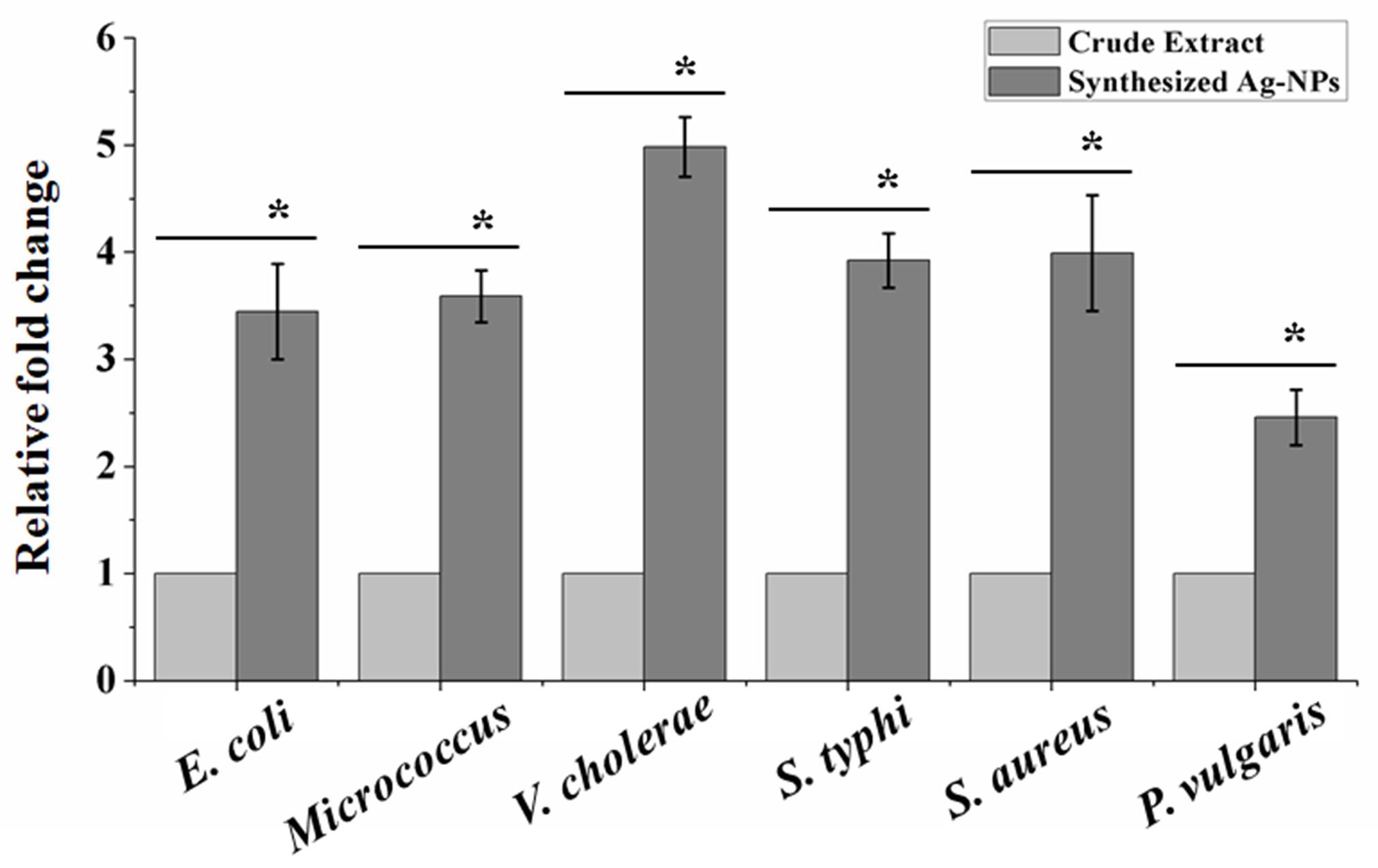
Higher dose of the synthesized Ag-NPs showed higher antibacterial activity
From the above results it was observed that synthesized Ag-NPs were more potent than crude extract. However, it was not clear that whether the increased dose of synthesized Ag-NPs also show increased activity. To conclude this point we used a higher dose of 100 μg/disc to evaluate the antibacterial capacity against V. cholera, and S. aureus. The result showed that this increased dose was more potent as expected. The detail result is presented in Table 3.
Table 3. The antibacterial activity of synthesized Ag-NPs with a higher dose (100 μg/disc).
DISCUSSION
In recent years, scientists are focusing on the invention of different synthesis procedures of Ag-NPs. Among the strategies biological or green synthesis of Ag-NPs are considering safe and simple as the use of conventional methods are associated with toxic environmental substances [20]. In the current manuscript the antibacterial potential of synthesized Ag-NPs from the leaf extract of M. oleifera were evaluated. The synthesis of Ag-NPs reported here is a green and cost effective synthetic approach. The green synthesis was performed under sunlight irradiation for nanoparticle formation within minimal time duration. It was reported that reduction of Ag+ by the leaf extract occurred in the presence of direct sunlight within 1 hour [18]. The completion of reduction process was indicated by the change of color due to the surface plasmon phenomenon from green to dark brown [21-23]. In this study the color change was observed after 30 min of direct sunlight exposure. Thus, it indicates the suitability and accuracy of used synthesis procedure for Ag-NP formation. Furthermore, the use of renewable energy sources would be a promising alternative for silver nanoparticle production.
From the results it was observed that the green synthesis of Ag-NPs enhanced the bioactivity against different pathogenic bacteria. Enhancement of antimicrobial activity by addition of Ag-NPs was also reported before with other plant extracts [24-26]. From the different published reports it is observed that the antibacterial activity of Ag-NPs depend on the particle size of the synthesized Ag-NPs. The small-sized Ag-NPs exerting stronger bioactivities than large-sized Ag-NPs [27, 28]. In this study the size of the synthesized Ag-NPs were not measured. However, it was previously published that the use of direct sunlight favored the formation of small sized Ag-NPs. Moodley et al., showed that Ag-NPs synthesized from M. oleifera leaf extract using direct sunlight produced particles with an average diameter range of 9-11 nm [18]. Previous reports showed that Ag-NPs with oxidized surfaces (presence of silver, oxygen, carbon and nitrogen on the surface of Ag-NPs) enhanced their bioactivity by inducing holes on the bacterial surface [29-31]. Usually, biologically synthesized Ag-NPs carry silver, oxygen, carbon and nitrogen on their surfaces [18]. A major limitation of this study is that we did not perform any characterization of our synthesized Ag-NPs. However, we believe that the enhancement of antibacterial activity of the synthesized Ag-NPs was due to the smaller particle size and the oxidized particle surfaces as mentioned by previous reports.
CONCLUSION
In this study the antibacterial activity of synthesized Ag-NPs from M. oleifera leaf extract were evaluated against several pathogenic bacterial strains. The result showed a broad-spectrum of antibacterial susceptibility of the synthesized Ag-NPs. Thus, the green synthesis of Ag-NPs has promising antibacterial potential and could be used as a potent biomedicine.
ACKNOWLEDGEMENTS
This research was partially funded by Khulna University Research Cell; grant number KURC-RGP-15/2019. The authors are grateful to Laxmon Chandra Roy for his invaluable technical assistance. The founding sponsors had no role in the design of the study; in the collection, analyses, or interpretation of data; in the writing of the manuscript, and in the decision to publish the results.
AUTHOR CONTRIBUTIONS
AH was involved in conception and design of the experiments. AI contributed to perform the experiments and also analyzed data. CM contributed to manage entire manuscript drafting including preparation of figures and tables, critical revising of the manuscript. Finally, AH approved the current version of manuscript for publishing.
CONFLICTS OF INTEREST
There is no conflict of interest among the authors.
References
- [1]Crooks RMLBI, Sun L, Yeung LK, Zhao M. Dendrimer-Encapsulated Metals and Semiconductors: Synthesis, Characterization, and Applications. Topics in Current Chemistry. Springer: Berlin, Heidelberg, Germany, 2001.
- [2]Cohen-Karni T, Langer R, Kohane DS. The Smartest Materials: The Future of Nanoelectronics in Medicine. ACS Nano. 2012; 6: 6541-6545.
- [3]Shakeel Ahmed MAaSI. Chitosan: A Natural Antimicrobial Agent – A Review. J Appl Chem. 2014; 3: 493-503.
- [4]Sengupta S, Eavarone D, Capila I, Zhao G, Watson N, Kiziltepe T, et al. Temporal targeting of tumour cells and neovasculature with a nanoscale delivery system. Nature. 2005; 436: 568-72.
- [5]Konishi Y, Ohno K, Saitoh N, Nomura T, Nagamine S, Hishida H, et al. Bioreductive deposition of platinum nanoparticles on the bacterium Shewanella algae. J Biotechnol. 2007; 128: 648-53.
- [6]Nair B, Pradeep T. Coalescence of Nanoclusters and Formation of Submicron Crystallites Assisted by Lactobacillus Strains. Cryst Growth Des. 2002; 2: 293-298.
- [7]Willner I, Baron R, Willner B. Growing Metal Nanoparticles by Enzymes. Adv Mater. 2006; 18: 1109-1120.
- [8]Niraimathi KL, Sudha V, Lavanya R, Brindha P. Biosynthesis of silver nanoparticles using Alternanthera sessilis (Linn.) extract and their antimicrobial, antioxidant activities. Colloids Surf B. 2013; 102: 288-91.
- [9]Vankar PS, Shukla D. Biosynthesis of silver nanoparticles using lemon leaves extract and its application for antimicrobial finish on fabric. Appl Nanosci. 2012; 2: 163-168.
- [10]Narayanan KB, Sakthivel N. Green synthesis of biogenic metal nanoparticles by terrestrial and aquatic phototrophic and heterotrophic eukaryotes and biocompatible agents. Adv Colloid Interface Sci. 2011; 169: 59-79.
- [11]Singh S, Saikia JP, Buragohain AK. A novel ‘green’ synthesis of colloidal silver nanoparticles (SNP) using Dillenia indica fruit extract. Colloids Surf B. 2013; 102: 83-5.
- [12]Das S, Das J, Samadder A, Bhattacharyya SS, Das D, Khuda-Bukhsh AR. Biosynthesized silver nanoparticles by ethanolic extracts of Phytolacca decandra, Gelsemium sempervirens, Hydrastis canadensis and Thuja occidentalis induce differential cytotoxicity through G2/M arrest in A375 cells. Colloids Surf B. 2013; 101: 325-36.
- [13]Noruzi M, Zare D, Davoodi D. A rapid biosynthesis route for the preparation of gold nanoparticles by aqueous extract of cypress leaves at room temperature. Spectrochim Acta A Mol Biomol Spectrosc. 2012; 94: 84-8.
- [14]Prasad TN, Elumalai EK. Biofabrication of Ag nanoparticles using Moringa oleifera leaf extract and their antimicrobial activity. Asian Pac J Trop Biomed. 2011; 1: 439-42.
- [15]Zaffer M, Ahmad S, Sharma R, Mahajan S, Gupta A, Agnihotri RK. Antibacterial activity of bark extracts of Moringa oleifera Lam. against some selected bacteria. Pak J Pharm Sci. 2014; 27: 1857-62.
- [16]Tripathi A, Chandrasekaran N, Raichur AM, Mukherjee A. Antibacterial applications of silver nanoparticles synthesized by aqueous extract of Azadirachta indica (Neem) leaves. J Biomed Nanotechnol. 2009; 5: 93-8.
- [17]Alinezhad H, Baharfar R, Zare M, Azimi R, Nabavi SF, Nabavi SM. Biological activities of ethyl acetate extract of different parts of Hyssopus angustifolius. Pharm Biol. 2012; 50: 1062-6.
- [18]Moodley JS, Krishna SBN, Pillay K, Sershen, Govender P. Green synthesis of silver nanoparticles from Moringa oleifera leaf extracts and its antimicrobial potential. ADV NAT SCI-NANOSC. 2018; 9: 015011.
- [19]Biemer JJ. Antimicrobial susceptibility testing by the Kirby-Bauer disc diffusion method. Ann Clin Lab Sci. 1973; 3: 135-40.
- [20]Patra JK, Baek K-H. Green Nanobiotechnology: Factors Affecting Synthesis and Characterization Techniques. J Nanomater. 2014; 2014: 417305.
- [21]Chandrappa CP, Govindappa M, Chandrasekar N, Sarkar S, Ooha S, Channabasava R. Endophytic synthesis of silver chloride nanoparticles from Penicillium sp. of Calophyllum apetalum. ADV NAT SCI-NANOSC. 2016; 7: 025016.
- [22]Veerasamy R, Xin TZ, Gunasagaran S, Xiang TFW, Yang EFC, Jeyakumar N, et al. Biosynthesis of silver nanoparticles using mangosteen leaf extract and evaluation of their antimicrobial activities. J Saudi Chem Soc. 2011; 15: 113-120.
- [23]Govindappa M, Farheen H, Chandrappa CP, Channabasava, Rai RV, Raghavendra VB. Mycosynthesis of silver nanoparticles using extract of endophytic fungi, Penicillium species of Glycosmis mauritiana , and its antioxidant, antimicrobial, anti-inflammatory and tyrokinase inhibitory activity. ADV NAT SCI-NANOSC. 2016; 7: 035014.
- [24]Mohammad TGM, El-Rahman AFA. Environmentally friendly synthesis of silver nanoparticles using Moringa oleifera (Lam) leaf extract and their antibacterial activity against some important pathogenic bacteria. Mycopath. 2015; 13: 1-6.
- [25]Moyo M, Gomba M, Nharingo T. Afzelia quanzensis bark extract for green synthesis of silver nanoparticles and study of their antibacterial activity. Int J Ind Chem. 2015; 6: 329-338.
- [26]Dobrucka R, Długaszewska J. Antimicrobial Activities of Silver Nanoparticles Synthesized by Using Water Extract of Arnicae anthodium. Indian J Microbiol. 2015; 55: 168-74.
- [27]Martínez-Castañón GA, Niño-Martínez N, Martínez-Gutierrez F, Martínez-Mendoza JR, Ruiz F. Synthesis and antibacterial activity of silver nanoparticles with different sizes. J Nanopart Res. 2008; 10: 1343-1348.
- [28]Jeong Y, Lim DW, Choi J. Assessment of Size-Dependent Antimicrobial and Cytotoxic Properties of Silver Nanoparticles. ADV MATER SCI ENG. 2014; 2014: 763807.
- [29]Song KC, Lee SM, Park TS, Lee BS. Preparation of colloidal silver nanoparticles by chemical reduction method. Korean J Chem Eng. 2009; 26: 153-155.
- [30]Rao B, Tang R-C. Green synthesis of silver nanoparticles with antibacterial activities using aqueous Eriobotrya japonica leaf extract. ADV NAT SCI-NANOSC. 2017; 8: 015014.
- [31]Smetana AB, Klabunde KJ, Marchin GR, Sorensen CM. Biocidal Activity of Nanocrystalline Silver Powders and Particles. Langmuir. 2008; 24: 7457-7464.