Follow-up of bacterial and physicochemical quality of water during live transportation of Climbing perch (Anabas testudineus) in Bangladesh
Abstract
This study was conducted to evaluate the changes in viable count of bacteria and physicochemical parameters, and their correlations in the changing pattern during live transportation of Climbing perch, (Anabas testudineus). Investigations were conducted in three live fish supply channels of Bangladesh from Mymensingh to Dhaka (channel 1), Mymensingh to Sylhet (channel 2), and from Mymensingh to Rajshahi (channel 3). It took nearly 6 h in channel 1, 8 h in channel 2, and 8 h in channel 3 for the live fish to reach the unloading points of destination. Viable count of bacteria and physicochemical parameters, such as temperature, dissolved oxygen (DO), pH, and ammonia were recorded from 0 hr with 2 h interval during transportation. The viable count of bacteria in water increased several folds from the initial values and showed a regrowth within the system. In all the supply channels, water temperature and pH were more or less stable with the headway of the transportation, but remarkable differences were observed in the concentrations of DO and ammonia. Although the initial (0 hr) DO level was varying among the supply channels, a decreasing trend was observed at the end in every channel. On the other hand, ammonia concentration was gradually increased during the transportation process. Thus, a gradual decline in water quality (decreased DO level, increased ammonia concentration, and higher bacterial regrowth) may negatively affect the survival and quality of live fish during transportation.
INTRODUCTION
Fish transportation is a global necessity, related to human feeding [1] and transportation of live fish is a common practice among aquaculture facilities. The most obvious respect in which the road transport of live fish differs from that of terrestrial livestock is the requirement to provide a life-support system for the duration of the process [2]. Handling and the physical disturbances associated with loading, transport, and discharge have the potential to cause stress in live fish [2]. Climbing perch (Anabas testudineus), commonly known as Koi in Bangladesh, is a freshwater fish found in small rivers, canals, and swamps [3]. It is one of the most delicious fish with high market demand in Bangladesh and thus commercial Koi fish farming in the pond is becoming very popular [4]. Due to good market prices and consumer demands, Koi fish needs to transport from the production site to retail markets in the live condition. The transportation of fishes is influenced by many factors, including the fish species and its physical condition, loading density, physicochemical parameters, the duration of transport, etc. [5]. In Bangladesh, live fish is transported usually by two systems viz., “open truck system” and “tank system” with or without aeration. In the “open truck system,” the truck bed is usually covered with a plastic/ tarpaulin so that it can hold water and here the water volume varies with the carrying capacity of the truck. In the case of the “tank system”, low-cost plastic tanks with a capacity of around 1 m3 are used, which are sometimes reinforced by an iron frame [6]. Typically, in Bangladesh mainly Taki, Pangus, Koi, Shing, Magur fish are transported by truck as live form by plastic drum from the production site. Each drum contains 35-40 kg of fish with water [7].
Water that is chemically and physically suitable for normal fish at the beginning of the transport operation can easily become contaminated with the organic toxin, resulting from the bacterial breakdown of fecal waste, that the carrying medium becomes harmful to the fish
[8]. Water quality is a crucial factor in the transportation of live fish and can be affected by the condition and/or deterioration of the holding systems [9]. The number (or weight) of fish that can be successfully transported depends on water quality, the duration of the transport, water temperature, fish size, and the species [10]. Maintenance of water quality parameters like dissolved oxygen (DO), pH, temperature, carbon dioxide, ammonia at an optimal level is essential in the successful transportation of live fish [9]. The major water quality effects experienced by fish during live transportation were low DO levels due to oxygen consumption by respiration, accumulation of carbon dioxide from respiration, depression of pH caused by carbon dioxide accumulation, and increased ammonia levels resulting from ammonia excretion [11]. Long-transport episodes lead to more extreme values of pH and ammonia in the water [12]. Microorganisms introduced into a live fish distribution system, through the raw water, will change density and diversity due to the selective pressures of the system. The goal here is to better integrate these two approaches, relating physicochemical consequences to water quality deterioration and microbial changes especially considering oxygen deprivation and enrichment of ammonia after transport. Treatment of raw water with different chemicals will result in a decrease in microbial load, with many live fish distribution systems later experiencing an increase in bacterial numbers with a distance away from the point of treatment. This increase has been termed regrowth and is recognized as a major problem within many live fish distribution systems [13].
The regrowth of bacteria within any fish distribution system is of concern to fish traders for a variety of reasons. Many microorganisms found in live fish distribution systems also can behave as opportunistic pathogens [14, 15]. Poor water quality causes burn of the slime coat or stress the fish making it more susceptible to bacterial infection [16]. The slime produced by the fish is another substrate for bacterial growth that consumes O2 and releases toxic metabolites resulting in a decrease in the water oxygen content [17]. During the transportation of fish in live conditions, adverse changes of physicochemical parameters and bacterial loads in water are frequent which influence much in successful transportation. Fish is affected by bacteria and the degradation of fish is accelerated by microorganisms associated with aquatic environments as well as contaminants during post-harvest handling [18]. Changes in physicochemical parameters and viable bacterial count and the correlation of different factors with viable bacterial count during live transportation of A. testudineus are not well understood in Bangladesh. We hypothesized that the bacterial count will be increased with the distance and duration of the transportation, and the DO level will be reduced and reach a critical level; the ammonia concentration will be increased with time which will ultimately result in bacterial regrowth to occur. We also hypothesized that the bacterial viable count will be correlated with the concentration of ammonia and the DO level in the water. Thus, the objective of this study is to verify these hypotheses. Three supply channels of live A. testudineus transportation were selected and sub-samples were collected to follow the regrowth of bacteria and changes of physicochemical parameters from the beginning (at 0 h of loading) of the supply channel to the end/ final destination (at point of unloading).
MATERIALS AND METHODS
Supply channels details
The study was conducted in three different A. testudineus supply channels of Bangladesh. The loading/stating point was a major fish producing division, Mymensingh (Muktagacha, 24°44′01″N 90°20′36″E) and unloading point was three important divisions such as Dhaka (Showarighat, 23°42′25″N 90°24′34″E; channel 1), Sylhet (Poschim Kazir Bazar, 24°.88′80″N 91°.86′40″E; channel 2) and Rajshahi (Shaheb Bazar, 24°21’53″N 88°35’66″E; channel 3) respectively (Figure 1). The samples were collected from July to September 2019.
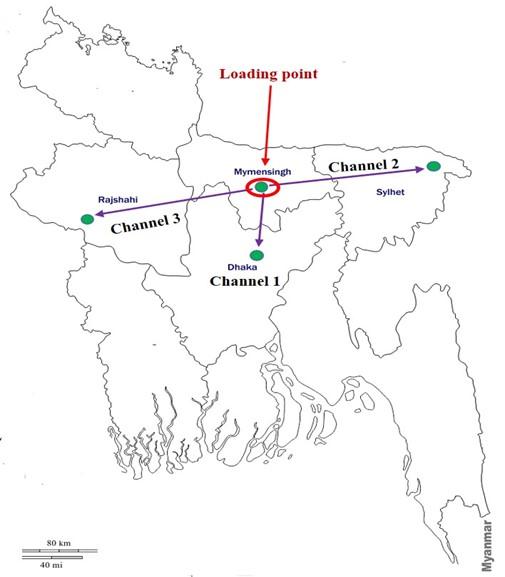
Sampling techniques
Thoroughly washed and sterilized sampling materials like beakers, volumetric flasks, tips, plastic containers, physiological saline, test tube, L-shaped glass rod, micropipette, hand gloves, cotton, etc. were carried within the iceboxes and traveled by the same carrying vehicle
(commercial truck) to carry out works during transportation. Previously prepared agar plates and necessary reagents for physicochemical parameters were also carried. For the viable count of bacteria and physicochemical parameters, water samples were collected randomly in triplicate from different sites of the plastic barrels in previously sterile plastic bottles (250 ml) from 0 hr and at every 2 hrs interval throughout the transportation. In this experiment after the collection of water from the plastic barrels, subsequent analyses and plating for viable counting were carried out immediately.
Determination of physicochemical parameters
Water temperature was recorded using a glass thermometer (Saraan Scientific Industries, Haryana, India) by sticking the bulb end into the water, waiting for a few minutes until the liquid in the glass stops moving. Finally, the temperature was measured as °C.
The DO was measured as mg/L by using Biosol dissolved oxygen kit (Biosol AE DO8 Dissolved Oxygen Kit, A.A. Biotech Private Limited, Chennai, India). The DO test bottle was filled with sample water till it overflew and then stoppered the bottle and ensured that no air bubbles were trapped inside. Then, 10 drops of DO-1 followed by 10 drops of DO-2 were added and waited for a minute. Brown precipitation was formed and started to settling. The bottle was kept in a safe place for a minimum of 20 min. Then 10-20 drops of DO-3 were added and shook the bottle till the precipitate dissolved. In the test jar, 10 ml of sample was taken, 4 drops of DO-4 were added and mixed well, and then DO-5 was added drop-wise until the blue colors disappeared. At last dissolved oxygen was calculated by using the following formulae: Dissolved oxygen (mg/l) = 0.65× (No. of drops of DO-5).
Water pH was measured by using a pH test kit (Charoen Pokphand Feed Company, Thailand). A color cell was washed with the sample water. Then 5 ml of sample was taken in the color cell. Two drops of pH reagent were added and mixed well. Lastly, pH was determined by comparing it with the standard color gradation.
The ammonia concentration (mg/l) was measured by using an ammonia test kit (Charoen Pokphand Feed Company, Thailand). At first, the measuring vessel of the kit was rinsed with the test sample and filled to the 5 ml mark. Two drops of the reagent AMMONIA-1 were added and mixed. Two drops of reagent AMMONIA-2 were added and mixed. Then two drops of reagent AMMONIA-3 were added and mixed thoroughly. At last four drops of the reagent AMMONIA-4 were added and mixed. After 20 minutes, the color on the top view of the measuring vessel was compared with the color band.
Determination of viable count of bacteria
After the collection of the water sample, 1 ml was transferred with a micropipette to a test tube containing 9 ml of physiological saline (0.85% w/v NaCl). The test tube was shaken thoroughly by a vortex mixture to get 10-1 dilution of the original sample solution. Using a similar process several dilutions of 10-2, 10-3 were made. Aliquots of 0.1 ml of the above dilutions were pipetted out and transferred aseptically to the previously prepared plate count agar plates (HiMedia Laboratories Private Limited, Kolkata, West Bengal, India) with maintaining replication of each, by raising the upper lids sufficient enough to admit the tip of the pipette. The samples pipetted were spread by L-shaped glass rods throughout the surface of the media until the samples were dried out. The plates were then wrapped by aluminum foil and kept in the zipper bag, where the temperature was around 28°C. All these activities were carried out aseptically in the cabinet of the truck and then plates were brought to the Fisheries Microbiology Laboratory, Dept. of Fisheries Technology, Bangladesh Agricultural University and put in the incubator at 30°C. After 48 h of incubation, the developed colonies were counted using the Stuart Scientific colony counter (SC6PLUS-UK). The plates having a number of colonies in between 30 to 300 were considered for calculation as colony forming unit (cfu) per ml of samples using the following formulae: cfu/ml = no. of colonies on petridish × 10 × dilution factor
After calculation, the mean logarithmic values (log10 cfu/ml) were obtained for every subsample of water.
Statistical analysis
All the collected data for both physicochemical parameters and bacterial viable count were incorporated and analyzed using Microsoft Excel 2010. Statistical analysis was performed by SPSS software (SPSS-24.0) and Pearson’s correlation was done to evaluate the significant relationship between physicochemical parameters and bacterial viable count at 5% level (p<0.05).
RESULTS
Length and duration of the supply channels
The distances between starting to the end of the supply channels are about 140 km, 305 km, and 230 km, respectively in the case of channel 1,
channel 2, and channel 3. But the time duration was not completely relevant to the distance that was about 6 h, 8 h, and 8 h for channel 1, channel 2, and channel 3, respectively due to traffic jams on the road.
Fish harvesting and preparation for transportation
At the fish loading point (commercial climbing perch farm), the capturing of fish was undertaken during the late afternoon for transportation the following night. The fishes are usually harvested using a large seine net. After catching, the fishes were kept in “hapas” (net made rectangular barriers) setting at the corner of the fishponds for acclamation usually for about 2 h before transportation.
For transportation of fish in the live condition, a commercial truck Tata LPT-709 (5029.2 mm × 2286 mm × 2341 mm) maximum carrying capacity of 5.17 ton from (Tata Motors Limited, Mumbai, India) and to provide a life-support system (water) for the duration of transport plastic barrel (1000 L) was used. Plastic barrels were filled with 500 L of groundwater from the commercial water supplier before 3-4 h of transportation and carried on the truck bed to the targeted loading point. For road transportation, each barrel was filled with 35 kg of fish approximately (on average 3 fishes per kg weight) and a maximum of 40 barrels was placed on the truck in every channel. Any kind of water additives, aeration facilities, and water exchange not practiced throughout the transportation in all supply channels.
Physicochemical parameters
Physicochemical parameters as water temperature (°C), pH, ammonia (mg/l), and dissolved oxygen (mg/l) were measured from 0 h just before loading of the fish up to reach the desired unloading point from all the three supply channels every 2 h interval. It was found that the water temperature changed from 29-31 °C in channel 1, while 30-29 °C in channel 2 and 31-30 °C in channel 3. Dissolved oxygen (DO) decreased from 6.25 to 2.05 mg/l in channel 1, while 7.35 to 1.95 mg/l in channel 2, and 6.75 to 1.65 mg/l in channel 3. The pH values of the water in all the supply channels were found to decrease slightly, reduced from 8.0 to 7.0 in channel 1, from 8.2 to 6.7 in channel 2, and from 7.6 to 7.0 in channel 3. The ammonia level increased from 0.1 to 5.0 mg/l in channel 1, while 0.5 to 5.0 mg/l in channel 2, and 0.2 to 3.0 mg/l in channel 3, respectively (Figure 2).
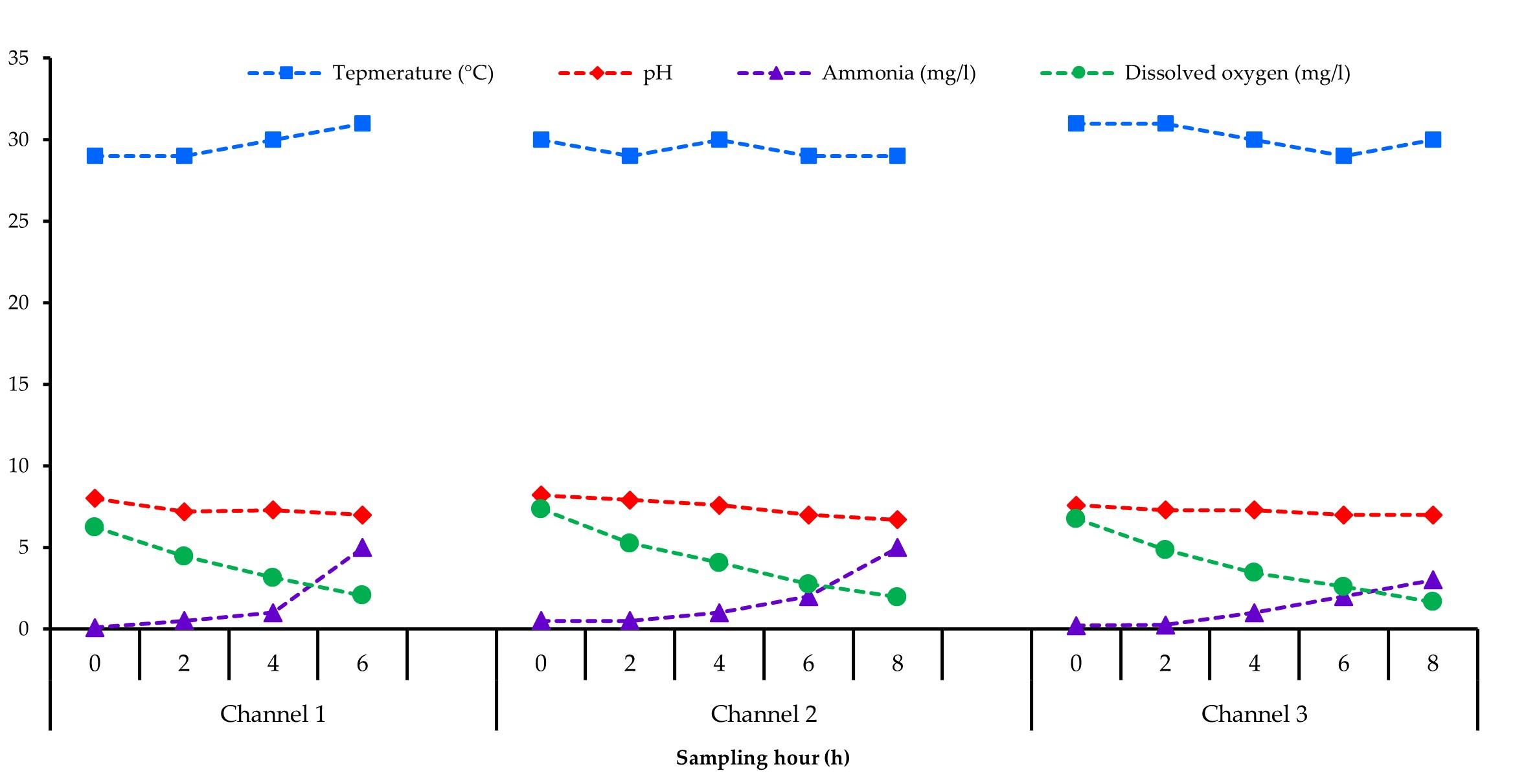
Viable count of bacteria
In this study, the averages of the viable count of bacterial in water changed from about 0.64×104 cfu/ml at 0 h to 16.32×104 cfu/ml at 2 h, 28.85×104 cfu/ml at 4 h, and to 31.01×104 cfu/ml at 6 h (before unloading) of transportation in channel 1. While in channel 2, the averages of the viable count were about 0.56×104 cfu/ml at 0 h, about 18.27×104 cfu/ml at 2 h, about 24.55×104 cfu/ml at 4 h to about 38.52×104 cfu/ml at 6 h, and 47.52×104 cfu/ml at 8 h of transportation. In the case of channel 3, the averages of the viable counts of bacteria changed from about 0.69×104 cfu/ml at 0 h to about 16.98×104 cfu/ml at 2 hrs, about 23.07×104 cfu/ml at 4 h to about 29.52×104 cfu/ml at 6 hrs, and finally about 41.01×104 cfu/ml at 8 h of transportation (Figure 3).
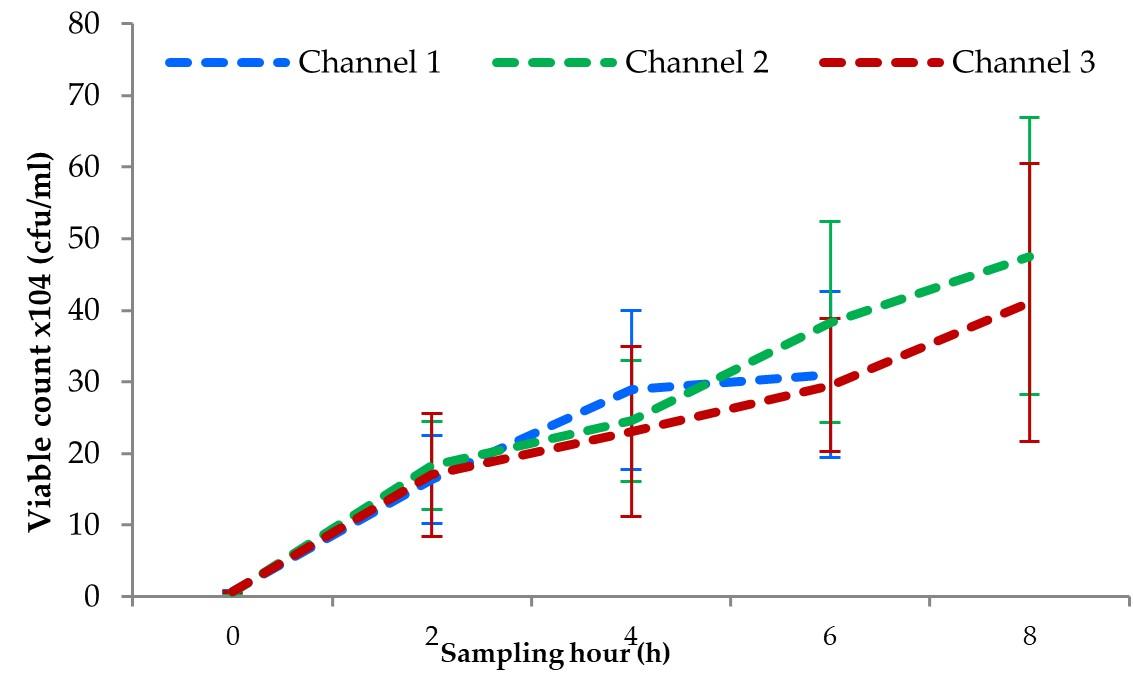
Relationship between viable count and physicochemical parameters
Throughout the study, it was observed that the DO level decreased while NH3 concentration increased gradually. The viable count also increased gradually from the loading to the unloading points in all the three supply channels (Figure 4, 5, and 6). Pearson correlation analysis between the viable count of bacteria and physicochemical parameters was carried out and the relationship was considered for significance at p < 0.05. In the case of channel 1, between viable count and ammonia, a moderate positive correlation (r= 0.689) was observed, while between viable count and DO level, a strong negative correlation (r= -0.981) was assessed. In the case of channel 2 and channel 3, Pearson correlation analyses between viable count and ammonia showed a significant positive correlation (r= 0.879 and r= 0.916, respectively for channel 2 and channel 3). Similar to channel 1, a strong negative correlation (r= -0.994, and r= -0.984, respectively for channel 2 and channel 3) was assessed between viable count and dissolved oxygen level in channel 2 and channel 3. Moderate to strong negative correlations (r= -0.814, r= -0.838, and r= -0.909 in channel 1, channel 2, and channel 3, respectively) were observed between ammonia concentration and DO level in all the studied channels (Table 1).
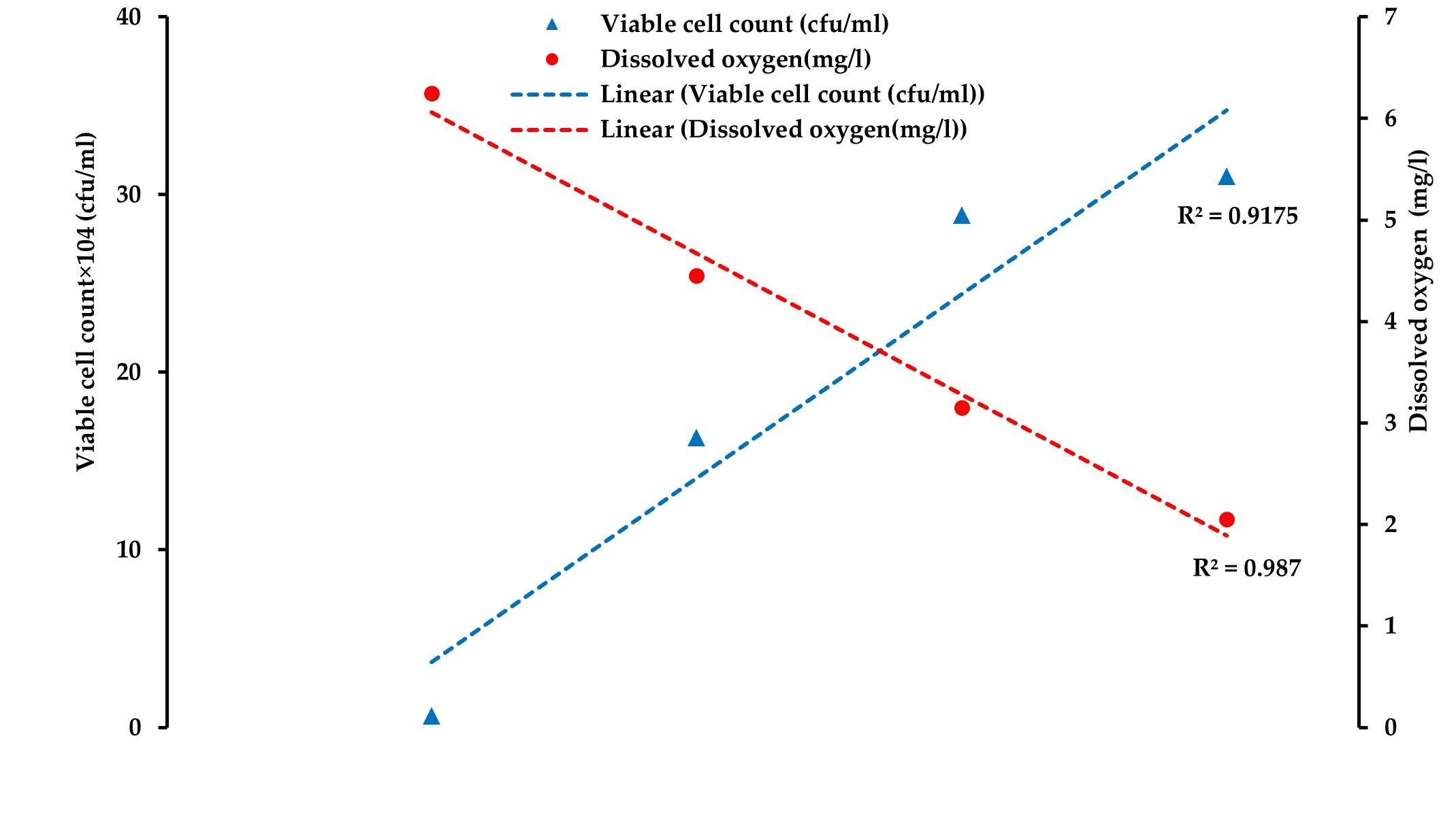
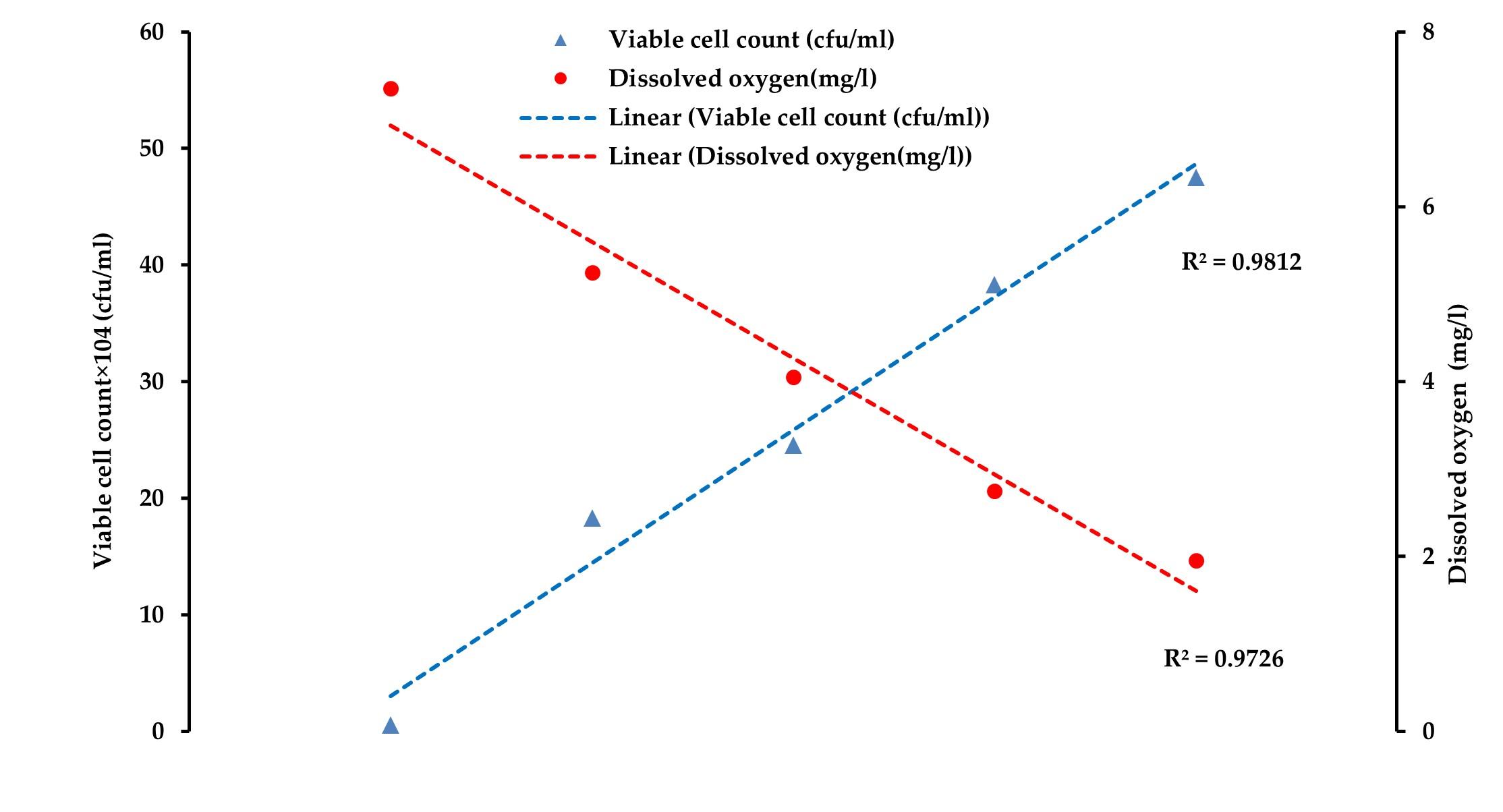
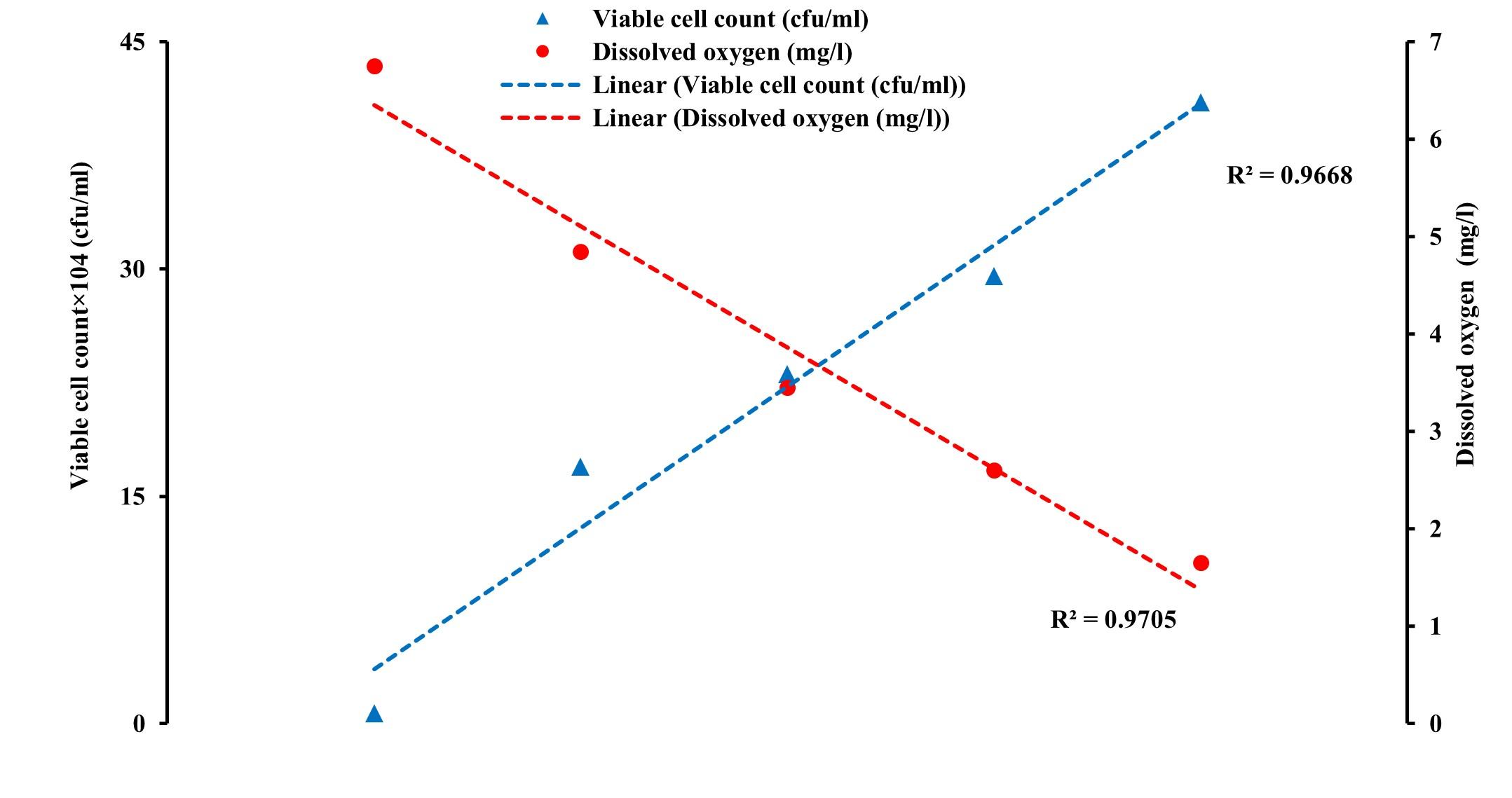
Table 1. Correlations between viable count of bacteria and physicochemical parameters (DO level and ammonia concentration) in different supply channels during live transportation of Climbing perch (Anabas testudineus) in Bangladesh.
DISCUSSION
This study was conducted to assess the changing pattern of bacterial count and water quality parameters during live transportation of climbing perch, A. testudineus. We hypothesized that the viable count of bacteria will be increased with the distance and duration of the transportation, and the DO level will be reduced and reach a critical level; the ammonia concentration would be increased with time which will ultimately result in bacterial regrowth. We also hypothesized that the bacterial viable count will be correlated with the concentration of ammonia and the DO level in the water.
Results of this study indicated the occurrence of bacterial regrowth within the live A. testudineus transportation system with relation to the changes of physicochemical parameters and they are significantly correlated. The greatest challenge with any live fish transportation is to keep the water quality parameter near the optimum range to minimize stress and mortality since a remarkable percentage of fish die before reaching the desired market. Among the physicochemical parameters assessed in this study, temperature and pH are the most important that control behavioral characteristics of organisms, the solubility of gases and salts in water as well as chemical and physical characteristics of water [19]. The results of water temperature were almost stable at around 30 °C in all the three supply channels (Figure 2) which is comparable with the findings of [20, 21] as they were observed temperature fluctuation during transportation of fish in live conditions was negligible.
The water pH is an important water quality parameter for aquatic organisms to survive since most of the chemical reactions in the aquatic environment are controlled by any change in its value. A decreasing trend of water pH during fish transportation was reported by [12, 22]. In the current study, pH values were decreased from the initial values with time intervals in all of the three studied supply channels, and the averages were from 8.0 to 7.0 (Figure 2). However, the pH range was within the optimum level as it was reported that pH levels 6.90 to 7.43 are tolerable to climbing perch [23].
In this study, the DO was found to decline sharply from the initial loading to unloading period and reached a critical in all three supply channels (Figure 2). The fish transporters usually fill the plastic barrels with groundwater several hours before loading the fish and carried to the loading point by truck. In the meantime on the road, due to the interaction of air and water on the truck, the DO levels may increase slightly. As a result, we found an average DO level of about 6.70 mg/l initially in all three supply channels. In live fish transportation, the initial 30–60 min in the transport container is critical [10] and a drop from 20 to <5 mg/l DO during this time was noticed by [24]. A similar condition was also observed here may be due to the increased activity by the fish during the initial tank loading process. At the later stage of transportation, the DO level continued to decrease and reached a critical level (2.05 mg/l, 1.95 mg/l, and 1.65 mg/l in channel 1, channel 2, and channel 3, respectively).
DO is a limiting factor at very high loading densities over an extended period. The decomposition and oxidation of organic matter reduce the solubility of oxygen in the water [25].In this study, although the DO level reduced to the critical limit, most of the transported fish was survived may be due to the following reasons. Firstly, the interaction between water and air added oxygen to the water during the running of the transport, especially at the surface. However, at the same time the DO was utilized by the fishes. Secondly, A. testudineus possesses accessory air-breathing organs and can withstand harsh environmental conditions such as low DO, a wide range of temperature, and other poor water conditions.
There was a noticeable increase in concentrations of ammonia (almost 5 mg/l) was found in all the studied channels with the progress of the transportation period (Figure 2). Ammonia accumulates in water during live fish transportation, mostly due to the catabolism of protein and excretion by the fishes. Besides, bacterial decomposition of organic matters such as uneaten feed, fecal materials, dead algae, and aquatic plants also enhances ammonia accumulation [19, 26]. Higher levels of NH4+ concentration are associated with low dissolved oxygen levels may lead to the death of fish [27]. Also, high NH4+ concentrations can strongly influence dissolved oxygen levels because around 4.3 mg oxygen is necessary to oxidize 1.0 mg NH4+ [27, 28]. Both in nature and under typical culture conditions, excreted CO2 and NH3 tend not to accumulate because of their high solubility and the potential for extensive dissolution. In contrast, during live transportation of fish, significant accumulation of these potentially toxic waste products in the fixed volume of water available is possible. Fortunately, during dissolution, the majority of NH3 is converted to the nontoxic ammonium ion (NH4+) [2].
Ammonia is toxic, and therefore, air-breathing fishes have special adaptations to defend against ammonia toxicity [29, 30]. Although the ammonia level was higher, the fishes were survived throughout the transportation period in all the supply channels.
In the present study, bacterial viable count in water increased several folds from the initial values and found positively related to the duration and distance of transport (Figure 3). Viable counts were also negatively correlated with the DO level and positively correlated with the ammonia concentration of the water during live transportation of climbing perch in all the supply channels (Table 1 and Figure 4, 5, 6).
Although groundwater is used for the transportation of the fishes, bacterial numbers may be increased in the water as fish itself is a source of bacteria (it is well known that fish can harbor around 105 cfu/inch2 bacteria in the skin, and 105 cfu/g in gill and intestine) that transferred a wide range of bacteria in water [31]. Further increase in bacterial number is due to their regrowth by utilization excretory organic matters of the transport water. The temperature of the water was almost around 30 °C in all the supply channels, which facilitated the metabolic activities of microbes and hence increased growth [32]. It was reported that there were usually negative correlations between viable bacterial count and dissolved oxygen [33, 34] and positive correlations between viable bacterial count and ammonia [34, 35] in water. So in this study, the higher the temperature, the higher was the growth rate of bacteria, and the decomposition of fecal organic matters resulted in the critical declined in DO level in the water during transportation. Thus, the viable count of bacteria was negatively correlated with the DO level and positively correlated with the ammonia concentration in the water during live transportation of climbing perch. The findings showed a gradual decline in water quality (decreased DO level, increased ammonia concentration, and higher bacterial growth) that may negatively affect the survival and quality of live fish during transportation.
ACKNOWLEDGMENTS
The authors are grateful to the Bangladesh Agricultural University Research System (BAURES) for funding the research and also to the Department of Fisheries Technology, Bangladesh Agricultural University, for their technical supports.
AUTHOR CONTRIBUTIONS
The work was designed by MNH, MNU, and MIH. MMH, ANMRKB conducted the experiments and collected data. MAH also aided in data collection. MNH, MNU, and MIH supervised the whole work. The first draft of this manuscript was prepared by MMH. MNH critically checked, improved the manuscript and finally approved it for publication.
CONFLICTS OF INTEREST
There is no conflict of interest among the authors.
References
- [1]Marcalo A, Pousao P, Mateus L, Duarte JH, Stratoudakis Y. Sardine early survival, physical condition and stress after introduction to captivity. J Fish Biol 2008;72:103–20.
- [2]King HR. Fish transport in the aquaculture sector: An overview of the road transport of Atlantic salmon in Tasmania. J Vet Behav Clin Appl Res 2009;4:163–8.
- [3]Mijkherjee M, Praharaj A, Das S. Conservation of endangered fish stocks through artificial propagation and larval rearing technique in West Bengal, India. Aquac Asia 2002;7:8–11.
- [4]Hossain MA, Sultana Z, Kibria ASM, Azimuddin KM. Optimum dietary protein requirement of a Thai strain of climbing perch (Anabas testudineus) (Bloch, 1792) fry. Turk J Fish Aquat Sci 2012a, 12: 217-224.
- [5]Carneiro PC, Kaiseler PH, Swarofsky EA, Baldisserotto B. Transport of jundia Rhamdia quelen juveniles at different loading densities: Water quality and blood parameters. Neotrop Ichthyol 2009;7:283–8.
- [6]Rajts F, Shelley CC. Guide to improving live fish transportation with special attention to the COVID-19 pandemic in Bangladesh and other tropical developing countries 2020.
- [7]Alam MJ, Yasmin R, Rahman A, Nahar N, Pinky NI, Hasan M. A study on fish marketing system in Swarighat, Dhaka, Bangladesh. Nature and science 2010; 8(12):96-103.
- [8]Washburn GN. Device for the transportation and storage of live fish. United States patent US 2,652,807. 1953 Sep 22.
- [9]Metar S, Chogale N, Shinde K, Satam SB. Transportation of live marine ornamental fish. Adv Agric Res Technol 2018.
- [10]Conte FS. Stress and the welfare of cultured fish. Appl Anim Behav Sci 2004;86:205–23.
- [11]Paterson BD, Rimmer MA, Meikle GM, Semmens GL. Physiological responses of the Asian sea bass, (Lates calcarifer) to water quality deterioration during simulated live transport: Acidosis, red-cell swelling, and levels of ions and ammonia in the plasma. Aquaculture 2003;218:717–28.
- [12]Treasurer JW. Changes in pH during transport of juvenile cod (Gadus morhua) L. and stabilisation using buffering agents. Aquaculture 2012;330–333:92–9.
- [13]Edge JC, Finch PE. Observations on bacterial aftergrowth in water supply distribution systems: implications for disinfection strategies. Water Environ J 1987;1:104–10.
- [14]Burke V, Robinson J, Cooper M, Beaman J, Partridge K, Peterson D, Gracey M. Biotyping and virulence factors in clinical and environmental isolates of Aeromonas species. Appl Environ Microbiol 1984;47:1146–9.
- [15]Burke V, Robinson J, Gracey M, Peterson D, Meyer N, Haley V. Isolation of Aeromonas spp. from an unchlorinated domestic water supply. Appl Environ Microbiol 1984;48:367–70.
- [16]Wendelaar SE. The stress response in fish. Physiol Rev 1997;77:591–625.
- [17]Berka R. The transport of live fish. A review. EIFAC Technical Paper 1986; 48, 52 pp.
- [18]Getu A, Misganaw K. Post-harvesting and major related problems of fish production. Fish Aquac J 2015;06.
- [19]Engdaw F. Physioco-chemical parameters and bacteriological qualities of water samples from wastewater treatment pond, University of Gonder, Ethiopia. Inter J Pharma Res 2014; pp.192-197.
- [20]Gomes LC, Araujo CA, Chippari AR, Roubach R. Transportation of juvenile tambaqui (Colossoma macropomum) in a closed system. Brazilian J Biol 2006;66:493–502.
- [21]Cerreta AJ, Lewbart GA, Harrison TM. A novel approach to ornamental fish transportation for the aquarium hobbyist. J Surv Fish Sci 2020;7:105–12.
- [22]Wang W, Zhang Y, Liu Y, Adanyi N, Zhang X. Effects of waterless live transportation on survivability, physiological responses and flesh quality in Chinese farmed sturgeon (Acipenser schrenckii). Aquacultur 2020; 518:734834.
- [23]Ahmed GU, Rahman MM, Alam MN, Alam MB, Sarker B. Impact of stocking density on growth and production performance of Vietnamese koi (Anabas testudineus) in semi-intensive culture system at Muktaghasa region of Mymensingh district. Res Agric Livest Fish 2015;2:335–41.
- [24]Fries JN, Berkhouse CS, Morrow JC, Carmichae GJ. Evaluation of an aeration system in a loaded fish-hauling tank. Progress Fish-Culturist 1993;55:187–90.
- [25]Khalil MA, Beltagy EA, Fattah El, El-Naga EHA, Elshenawy MA, Kelany MS. Seasonal bacteriological and physico-chemical analysis of Lake Timsah, Ismailia, Egypt. Life Sci J 2014;11:9–17.
- [26]Durborow RM, David MC, Martin WB. Ammonia in fish ponds. J Fish Res Board of Canada 1997: 2379-2383.
- [27]Golombieski JI, Silva LF, Baldisserotto B, Silva JS. Transport of silver catfish (Rhamdia quelen) fingerlings at different times, load densities, and temperatures. Aquaculture 2003; 216:95–102.
- [28]Esteves FA. Fundamentos de limnologia. Interciencia/FINEP. Rio de Janeiro, 1988: 575 pp.
- [29]Tay YL, Loong AM, Hiong KC, Lee SJ, Tng YYM, Wee NLJ. Active ammonia transport and excretory nitrogen metabolism in the climbing perch (Anabas testudineus) during 4 days of emersion or 10 minutes of forced exercise on land. J Exp Biol 2006;209:4475–89.
- [30]Randall DJ, Ip YK. Ammonia as a respiratory gas in water and air-breathing fishes. Respir Physiol Neurobiol 2006;154:216–25.
- [31]Mayer BK, Ward DR. Microbiology of finfish and finfish processing. Microbiol Mar Food Prod 1991:3–17.
- [32]LeChevallier MW. Coliform regrowth in drinking water. A review. J / Am water work assoc 1990;82:74–86.
- [33]Garcia OF, Gomez E, Santos VE, Merchuk JC. Oxygen uptake rate in microbial processes: An overview. Biochem Eng J 2010;49:289–307.
- [34]Power KN, Nagy LA. Relationship between bacterial regrowth and some physical and chemical parameters within Sydney’s drinking water distribution system. Water Res 1999;33:741–50.
- [35]Tsushima I, Kindaichi T, Okabe S. Quantification of anaerobic ammonium-oxidizing bacteria in enrichment cultures by real-time PCR. Water Res 2007;41:785–94.