Characterization of human articular cartilage derived mesenchymal progenitor cells from osteoarthritis patients
Abstract
Osteoarthritis (OA) is a leading pathological condition resulting in the degeneration and destruction of articular cartilage. The presence of inherent mesenchymal progenitor cells (MPCs) within the articular cartilage has led to explore the possible reparative mechanisms to regenerate and restore the functional and mechanical properties of hyaline cartilage. The present in vitro study was aimed to identify and characterize the MPCs derived from OA cartilage. MPCs derived from the explant culture of OA cartilage were analyzed in terms of cellular and biological properties, and multilineage differentiation abilities. Upon cell surface marker analysis, MPCs were CD73+, CD90+, CD166+, CD146-, CD34-, CD45-, and HLA-DR-, whose expression defines stemness and chondroprogenitor status. MPCs exhibited a higher proliferative index and limited or no senescence activity till later passages. Trilineage differentiation towards osteogenesis, adipogenesis, and chondrogenesis was observed with cytochemical staining and also by mRNA expression of lineage-specific markers by RT-qPCR. The results showed that OA cartilage harbors a viable pool of MPCs with greater chondrogenic potential. These cell niches could serve as a superior cell source for cartilage regeneration due to their committed progeny and hence could prevent heterotypic cartilage formation.
INTRODUCTION
Articular cartilage is a highly specialized connective tissue encircling the epiphysis of diarthrodial joints. This subtype of hyaline cartilage is greatly resilient and evenly distributes mechanical shear forces to assist the locomotion [1]. Articular cartilage harbors a divergent subgroup of mesenchymal progenitor cells (MPCs) among the other sparsely distributed terminally differentiated chondrocytes in a dense extracellular matrix [2]. The MPCs are multipotent cells residing in the articular cartilage, owing to the aging-induced MPCs scarcity, avascularity, and disease-induced malformations, the indigenous repair mechanisms are barely functional. To address these, therapies based on exogenous MPCs were evolved [3]. Also, over the years, a large body of literature has confirmed the phenotypic destabilization of highly differentiated chondrocytes during various pathological conditions [1,4].
Osteoarthritis (OA) is a chronic degenerative disease of joints, previously believed to be associated with aging or trauma but recent studies depict the involvement of lifestyle, genetics, and other idiopathic factors [5–8]. OA is one of the leading causes of disability and discomfort around the world affecting different age groups [8]. Primarily, OA is observed as an increase in anabolic and catabolic activity leading towards the destruction of articular cartilage, but the eventual progression of the disease is associated with hypertrophy of subchondral bone, osteophyte formation, synovial inflammation, and changes in the joint capsule, ligaments, and periarticular muscles [5,6]. Present treatment options for OA are mainly non-conservative and rather focus on pain management or lifestyle modifications to slow down the progression of the disease and also the prosthetic joint replacement in severe conditions. Although recent techniques in cell-based therapies, such as autologous articular chondrocyte implantation (ACI) and more advanced matrix-induced autologous chondrocyte implantation (MACI) were slightly successful, the quality of neo-cartilage formed is questionable. The relatable explanation for the compromised results of ACI or MACI could be the dedifferentiation of chondrocytes during ex vivo expansion leading to the formation of fibrocartilage [3,9].
For successful regeneration of diseased articular cartilage either by exogenous strategies, such as ACI or stimulating the intrinsic repair, it is crucial to understand the characteristics of MPCs. Despite the lack of key markers to identify MPCs, the present in vitro study aimed at investigating the cellular and biological properties, and plasticity complying with the International Society for Cellular Therapy criteria [10].
MATERIALS AND METHODS
Chemicals and media
All chemicals were purchased from Sigma-Aldrich (St. Louis, MO, USA) and media from Gibco (Invitrogen, Thermofisher Scientific, Grand Island, NY, USA), unless otherwise specified.
Ethics statement
This study followed the Declaration of Helsinki on medical protocol and ethics. This study design, including the experimental protocols, was reviewed and approved by the Central Ethics Committee (Ref: NU/CEC/2018/0177/A) and Institutional Committee for Stem Cell Research (Ref: NU/ICSCR/2017-2018/014/P2A). All the subjects involved in the study accorded with prior written informed consent.
Sample collection and isolation of OA cartilage-derived MPCs
OA articular cartilage (n=3, mean age: 63 years) from distal femoral condyles were collected from the patients presented with stiffness or tenderness in the knee joint due to grade 3 or grade 4 osteoarthritis (OA) (Kellgren and Lawrence system for classification of OA knee) and also were voluntarily undergoing total knee replacement surgery (TKR). The articular cartilage tissue fragments were stochastically chosen from the areas adjacent to osteoarthritic lesions. To isolate MPCs, cartilage tissue pieces were washed twice with Dulbecco’s phosphate-buffered saline (DPBS) supplemented with 100 U/ml penicillin, and 100 μg/ml streptomycin (1X Pen-Strep) and were minced into small pieces using a sterile scalpel and enzymatically digested with 0.1% collagenase type I for 2-3 hours at 37° C in a humidified 5% CO2 incubator. Following this, the semi-digested tissue pieces were seeded for explant culture onto 60 mm or 100 mm culture dish pre-coated with 0.1X attachment factor overnight at 37° C in a humidified 5% CO2 incubator with DMEM/F12 (1: 1) supplemented with 20% fetal bovine serum (FBS), 1X glutamax, 0.1 mM mercaptoethanol, 1X non-essential amino acids (MEM-NEA) and 1X Pen-Strep. The medium was replenished on every third day and followed by achieving confluency MPCs were subpassaged and further cultured with DMEM/F12 consisting of 10% FBS, 1% glutamax, 0.1 mM mercaptoethanol, 1X MEM-NEA, and 1X Pen-Strep.
Morphology, viability, and growth kinetics
MPCs were assessed for morphology at different time points of culture using a phase-contrast microscope (Olympus, Tokyo, Japan) and images were acquired. To assess viability and growth kinetics, cells at 1×105 at passage 2 were seeded per well of a 12-well culture dish in triplicates specifically for different time intervals such as 24 h, 72 h, 144 h, 216 h, and 288 h. Cells were harvested at the mentioned time intervals and counted using a hemocytometer under phase-contrast microscope. The initial seeding density and the recorded cell counts on different time points were used to draw a growth curve. Population doubling time (PDT) was calculated as, PDT = t (log2) / (log Nt-log No), where “t” was culture time, and “No” and “Nt” were the cell numbers before and after seeding, respectively. The Viability of harvested cells at various time points of a particular passage and between different passages was recorded using a 0.4% trypan blue exclusion test, according to which the blue-stained dead cells were excluded from the total cell count and unstained viable cells were expressed as a percentage viability.
Senescence activity
Replicative senescence of MPCs was measured qualitatively by using Senescence associated β-Galactosidase Staining Kit (SA-β-GAL) (Cell signaling technology, USA). For the assay, MPCs at passage 5 were seeded at a density of 1×104 cells per 35 mm dish and cultured until they reached 70-80% confluence. Then, MPCs were stained according to the manufacturer’s instructions. Briefly, cells were washed with DPBS and incubated for 15 min with 3.7% formaldehyde, and again washed twice with DPBS. Colour development was observed by incubation overnight at 37°C with the staining solution provided. Plates were then observed for the development of blue colour under phase-contrast microscope.
Colony-forming ability
To assess the colony-forming ability, MPCs in the passage 3 were seeded at a density of 5×102 cells in a 100 mm culture dish and cultured for 14 days followed by staining with crystal violet solution. The colonies with >50 cells were considered positive and were scored both microscopically as well as macroscopically.
Alkaline phosphatase activity
Culture expanded MPCs at passage 3 were plated at a density of 1×105 cells onto 35 mm cell culture dishes in duplicates and one of the replicate dishes was fed with basal media, the other with osteogenic induction media (described under phenotypic plasticity) for 11 days. Then cultures were stained with BCIP/NBT color development substrate kit (Promega, Madison, WI, USA) according to the manufacturer protocol. After the incubation period, the images were acquired using a phase-contrast microscope. Another set of 35 mm cell culture dishes with the same seeding density were maintained under similar culture conditions. After 11 days of incubation instead of staining with BCIP/NBT, they were subjected to the quantitative analysis of alkaline phosphatase (ALP) activity through mRNA expression by RT-qPCR.
Immunophenotyping analysis
MPCs at passage 3 were harvested and post washing with DPBS fixed in 3.7% paraformaldehyde for 15-20 min at room temperature. Followed by washing with cell staining buffer, cells were incubated with monoclonal mouse anti-human CD73 (Biolegend, USA), anti-human CD90 (e-Bioscience, Thermo Fisher Scientific, USA), anti-human CD105 (Biolegend), anti-human CD146 (e-Bioscience), anti-human CD166 (Biolegend), anti-human CD34 (Biolegend), anti-human CD45 (e-Bioscience), anti-HLA-DR (Biolegend), for 2 hrs in a water bath maintained at 37°C. After which the primary antibody labeled cells were incubated in dark with FITC-conjugated secondary antibody (e-Bioscience) for 2 hrs at room temperature. The standard was established by isotope-matched control (e-Bioscience). A total of 10,000 FITC-labeled cells were acquired and analyzed by flow cytometry (BD FACSCalibur, Becton Dickinson, NJ, USA).
Phenotypic plasticity
MPCs were induced to differentiate into osteocytes, adipocytes, and chondrocytes to determine phenotypic plasticity. Osteogenic, adipogenic, and chondrogenic differentiation was performed in monolayer cultures. Briefly, 2×105 cells were seeded per well of a 12-well culture dish and cultured in DMEMF12 medium supplemented with 10% FBS until they reached 70-80% confluency. Thereby media was regularly changed every 3 days with appropriate differentiation media for 28 days. Osteogenic differentiation media consisted of DMEM/F12 media supplemented with 10% FBS, 1% glutamax, 0.1 mM mercaptoethanol, 1X MEM NEA, 1X Pen-Strep 10 nM dexamethasone, 10mM β-glycerophosphate disodium and 0.1mM L-ascorbic-acid-2-phosphate. Adipogenic differentiation media contained DMEM/F12, 10% FBS, 1% glutamax, 0.1 mM mercaptoethanol, 1X MEM-NEA, 1X Pen-Strep, 1 µM dexamethasone, 100 µM indomethacin, 500 µM 3-isobutyl-1-methylxanthine, and 10 µg/ml human recombinant insulin. Chondrogenic media comprised of DMEM/F12, 10% FBS, 1% glutamax, 0.1 mM mercaptoethanol, 1X MEM-NEA, 1X Pen-Strep, 0.1 µM dexamethasone, 1% insulin-transferring-selenium (ITS), 0.1 mM L-ascorbic-acid-2-phosphate and 10 ng/ml transforming growth factor –β3 (MP Biomedicals, NJ, USA).
Gene expression by real-time PCR (RT-qPCR)
The mRNA expression level of osteogenic markers: Runt-related transcription factor 2 (RUNX2), osteopontin (OPN), and osteonectin (ON), adipogenic markers: lipoprotein lipase (LPL), adipocyte protein 2 (AP2), and peroxisome proliferator activated receptor-gamma (PPAR-γ) and chondrogenic markers: aggrecan (ACAN), collagen 2 alpha 1 (COL2-α1), SOX9, transforming growth factor-beta 3 (TGF-β3), and vimentin (VIM) were assessed in MPCs cultured for 28 days in specific induction media. MPCs cultured in basal media for 28 days were considered as control. Total RNA was extracted using RNAiso Plus kit (Takara, Japan) and was quantified using a nanodrop 1000 spectrophotometer (Thermo Fisher Scientific). Then, cDNA was synthesized using Prime script TM (Perfect real-time) RT Reagent kit (Takara, Japan) according to manufacturers’ protocol. The mRNA expression level of target genes was quantified by real-time PCR (Applied Biosystems, StepOnePlusTM) using SYBR® Premix Ex Taq TM II (Takara, Japan). ΔΔCT method was used to evaluate the relative fold expression of target genes, which was normalized against the expression level of β-actin for every sample. The primer sequences of the studied genes are presented in Table 1.
Table 1. Primer sequences used for RT-qPCR.
Statistical analysis
The data are expressed in terms of the mean ± standard deviation (SD) of each experiment performed in triplicates. The data were analyzed by GraphPad Prism 8 software. One-way or two-way ANOVA followed by Tukey’s multiple comparison tests were used. P < 0.05 was considered statistically significant.
RESULTS
Morphology and viability
A total of three cell lines, designated as MPCs-1, MPCs-2 and MPCs-3 were successfully established in the present study. MPCs were plastic adherent cells with small spindle-shaped fibroblast-like morphology since their release in the primary culture (P0) (Figure 1A) up to late passages (P5) (Figure 1B). When the viability was assessed at different passages, the mean percentage viability varied between 98% and 83% from early to later passages. However, a statistically significant difference was observed at passage 3 between MPCs-1 Vs MPCs-2 (P=0.0182) and passage 5, MPCs-2 Vs MPCs-3 (P=0.0275) (Figure 2A). Viability was also assessed at different time points of passage 2, but there was no statistically significant (P>0.05) difference in the mean percentage viability (>95%) (Figure 2B).
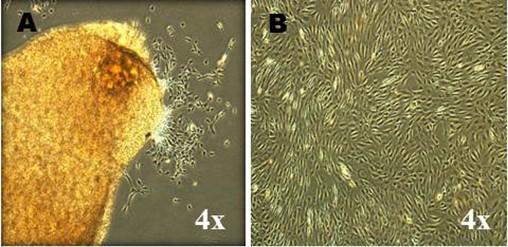
Proliferative index
Growth kinetics analysis depicted a linear proliferation rate of MPCs with an ideal growth phase consisted of a transient lag phase followed by a logarithmic phase and lastly, the stationary phase began at the onset of day 9. Despite the increase in the proportion of live cells over time upon statistical testing, there was a significant difference in the proliferation rate of MPCs-1 Vs MPCs-2 (P=0.0171) and MPCs-2 Vs MPCs-3 (P=0.0439) on day 6 (Figure 2C). Also, the mean PDT of MPCs was 49.72±7.94 hrs without any significant (P>0.05) difference between the cell lines (Figure 2D).
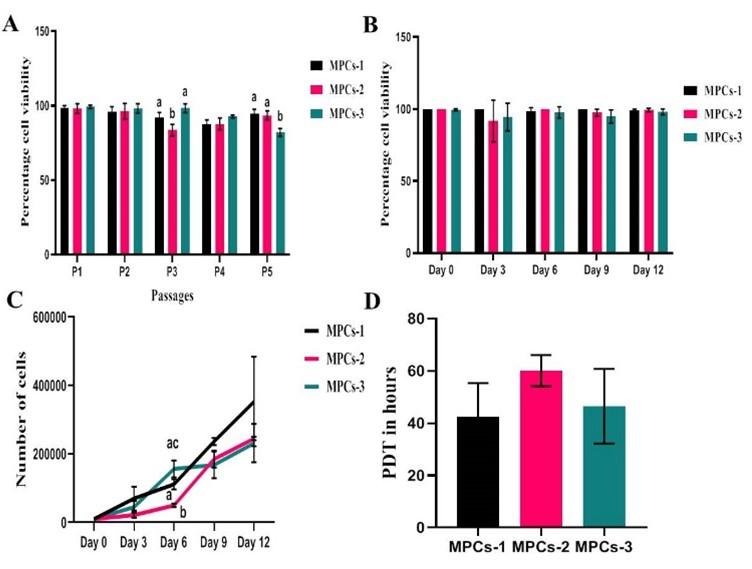
SA-β-GAL assay
At the end of the incubation period of SA-β-GAL staining, the characteristic blue-stained cells generally observed due to the catalytic action of SA β-Galactosidase in the hydrolysis of X-gal were not apparent under standard culture conditions. The results indicated the absence of viable quiescent enlarged cells undergoing replicative senescence (Figure 3A).
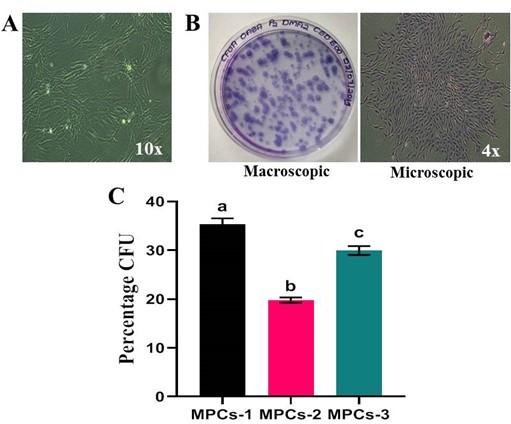
Colony-forming ability
The OA-derived MPCs were capable of forming colonies, each consisted of >50 cells and were stained positive for crystal violet solution (Figure 3B). When the colonies were quantified from two replicates of an individual experiment, the mean percentage colony-forming unit ability was 28.35%. Statistical analysis implied a remarkable difference in colony-formation ability amongst cell lines MPCs-1 Vs MPCs-2 (P=0.0015), MPCs-1 Vs MPCs-3 (P=0.0336) and MPCs-2 Vs MPCs-3 (P=0.0053) (Figure 3C).
Alkaline phosphatase activity
MPCS showed clear positive staining for ALP activity on the 11th day further manifesting inherent stemness and differentiation potential (Figure 4A and 4B). Further, the ALP staining of MPCs was also evident in the osteogenesis-induced cells than the non-induced with an altered morphology. These observations were validated by the mRNA expression of ALP using RT-qPCR (Figure 4C).
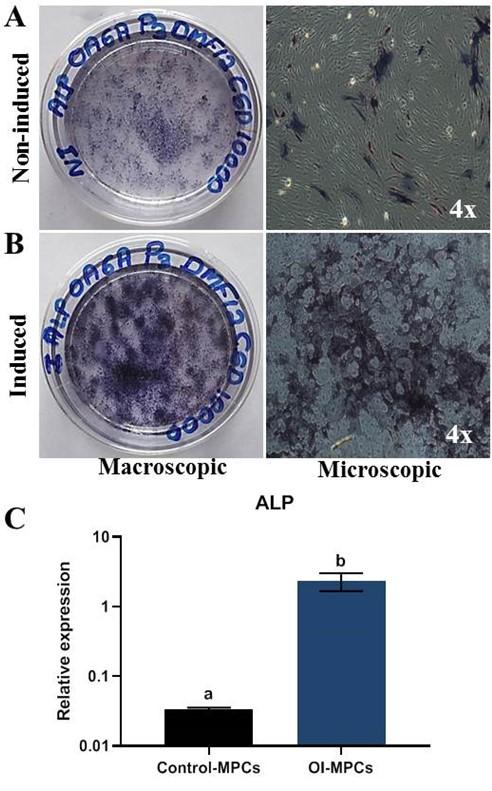
Phenotypic characterization
Flow cytometry results revealed a clear positive expression of cluster of differentiation markers, such as CD73, CD90, and CD166. Negative expression of hematopoietic cell markers, such as CD34, CD45, and HLA-DR affirmed MPCs existence (Figure 5). CD146, a stem/progenitor cell marker, was found negative in MPCs.
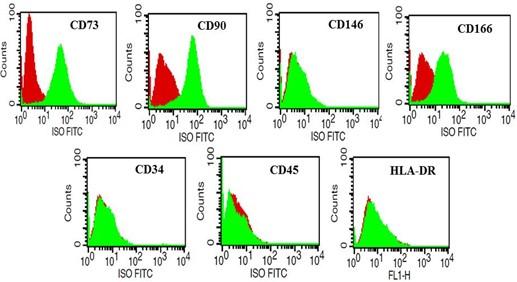
Phenotypic plasticity
At the end of the differentiation, the osteogenesis induced cell cultures displayed positivity to Alizarin red staining of mineral nodules (Figure 6A) and adipogenesis induced MPCs were able to form lipid-filled cytoplasmic vacuoles as stained with Oil red O (Figure 7A). Chondrogenesis-induced MPCs were also able to form Alcian blue-stained spheroids which were distinguishable both microscopically and macroscopically (Figure 8A). All the specific staining images were assessed against non-induced MPCs.
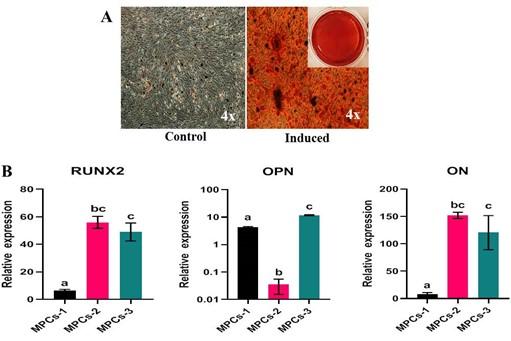
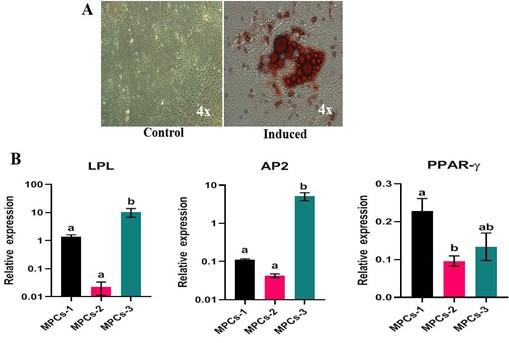
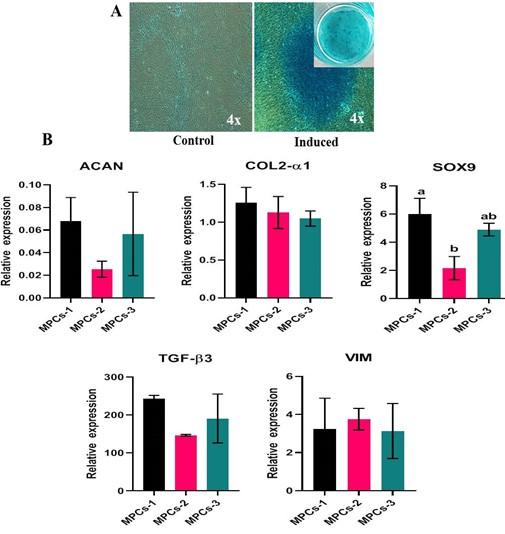
Gene expression analysis
Osteogenesis, adipogenesis, and chondrogenesis induced MPCs showed positive mRNA expression for the selected panel of genes by RT-qPCR. Also, there was a statistically significant (P<0.05) difference in the osteogenic and adipogenic specific relative mRNA expression among the cell lines. RUNX2: MPCS-1 Vs MPCs-2 (P=0.0034) and MPCS-1 Vs MPCs-3 (P=0.0053). OPN: MPCs-1 Vs MPCs-2 (P=0.0021), MPCs-1 Vs MPCs-3 (P=0.0004) and MPCs-2 Vs MPCs-3 (P<0.0001). ON: MPCs-1 VS MPCs-2 (P=0.0089) and MPCs-1 Vs MPCs-3 (P=0.0178). LPL: MPCs-1 Vs MPCs-3 (P=0.0439) and MPCs-2 Vs MPCs-3 (P=0.0303). AP2: MPCs-1 Vs MPCs-3 (P=0.0114) and MPCs-2 Vs MPCs-3 (P=0.0110). PPAR-γ: MPCs-1 Vs MPCs-2 (P=0.0414) (Figure 6B and 7B). Chondrogenesis specific mRNA expression revealed no statistically notable difference in the relative expression of a panel of genes between the cell lines except for SOX9: MPCs-1 Vs MPCs-2 (P=0.0399) (Figure 8B).
DISCUSSION
The MPCs are a readily available source of cells with self-renewal and multilineage differentiation ability. Although the physiology of OA disease progression and repair mechanisms involving MPCs remain unknown, the discovery of OA articular cartilage-derived MPCs with higher chondrogenic potential has led the researchers to attempt MPCs mediated cartilage repair in humans [11]. MPCs could be a heterogeneous population of cells with the varied phenotype and differentiation abilities attributed to different stages of OA, hence it is crucial to identify and enrich the cell source with appropriate chondrogenic lineage-committed cells to prevent irrelevant heterotopic cartilage formation [7,12]. This study was successful in isolating and characterizing the MPCs derived from OA cartilage and it further substantially strengthened the notion of the existence of MPCs in the later stages of OA cartilage.
The in vitro cultured cells were plastic adherent since their release in the primary culture, and exhibited a well-defined short spindle-shaped morphology resembling any other sources of MSCs derived from bone marrow, umbilical cord, placenta, and adipose tissue [13]. The viability and proliferation rate of MPCs play a key role in tissue engineering. It has been reported that, the chondrocytes are terminally differentiated cells and cannot thus proliferate or differentiate [14]. The current study observed a linear proliferation rate and higher viability which was maintained until later passages.
Colony-forming ability is an exceptional trait of stem/progenitor cells and can be very useful in the identification of MPCs. In the deficit of MPCs specific biomarkers, the colony-forming assay can be used extensively to distinguish clonal populations of progenitor cells [5]. Senescence is an irreversible cell cycle arrest instigated either by the shortening of telomeres or by stress-induced epigenetic alterations. Given that stem cells are known for their ability of self-renewal, plasticity, and also lack of replicative senescence, there is increasing evidence of cellular senescence impeding stem cell properties during advanced passages by barely understood mechanisms. Contrarily, extensive in vitro propagation of MPCs is crucial to obtain adequate cells for therapeutic applications [15,16]. Senescence-associated beta-galactosidase, along with p16Ink4A, is regarded to be a biomarker of cellular senescence and the most commonly used for the detection of cellular senescence [17,18]. In the current study, owing to the later stages and age of OA cartilage, the derived MPCs at passage 5 were qualitatively subjected to SA-β-Gal activity, which inferred least or no SA-β-Gal activity. Although the presence of divergent populations of senescent progenitor cells have been identified in many tissues, such as the epidermis of the skin, where the clonogenic cells decrease in aging. This does not seem to be observed in normal cartilage or OA cartilage-derived MPCs, probably due to the more quiescent state, unlike the epidermal cells [5].
Alkaline phosphatase (ALP) is a ubiquitous membrane-bound glycoprotein expressed in a wide variety of tissues and cells. High ALP activity, besides being considered as a traditional marker of pluripotent stem cells, is also attributed to the presence of undifferentiated cell phenotypes. Contrary to the prior notion in the current study high ALP activity was observed in osteogenesis induced MPCs than the non-induced MPCs, which suggests it can also be correlated with the status of differentiation rather than stemness. Unfortunately, the significance of ALP activity in pluripotent cells and MPCs is vaguely understood [19]. We hypothesize that the ALP expression in MPCs could be an indicator of progenitor cells rather than stemness, which can be supported by similar studies that documented high ALP activity in differentiated cells [19,20].
As specific surface markers representing the MPCs population are not known to date, the present study examined the phenotypic characteristics of MPCs using a set of surface antigen markers that represented both MSCs and MPCs. In addition to plastic adherence and plasticity, the cultured MPCs demonstrated positive expression for CD73, CD90, and CD166 and negative expression for CD146, CD34, CD45, and HLA-DR. These results established the presence of MSCs by confirming the ISCT position statement [10]. However, interestingly the MPCs were CD166+ and CD146-, both of which are amongst the others scarcely known markers that might represent progenitor cell niches. Only a few studies have reported the presence of MPCs in both normal and osteoarthritic cartilage and also have demonstrated significantly higher prevalence in OA cartilage [2,5,21,22]. Two studies have proven the superior chondrogenic ability of MPCs by using fluorescence-activated cell sorting for CD105 and CD166 [2,23]. CD166 has also been reported to be expressed in the progenitor cells of the perichondrium [24]. Few studies have considered CD146 as a marker of chondroprogenitor cell subpopulations with higher chondrogenesis and clonogenic potential [25,26]. Contrary to the previous observations, in the present study, MPCs were CD146-, but still were capable of undergoing chondrogenesis along with enhanced colony-forming ability.
Plasticity is an exceptional trait of MPCs to undergo trilineage differentiation into osteocytes, adipocytes, and chondrogenesis. In this study, MPCs in monolayer cultures were capable of undergoing osteogenesis, adipogenesis, and chondrogenesis when cultured in the appropriate medium, as reported previously [2,6,9,12]. CD105+/CD166+ cells do not express markers of differentiated chondrocytes, such as ACAN, COL2-α1 [2]. Vimentin, although a marker of the cytoskeleton is a regulator of chondrogenesis, which has been confirmed by few studies where siRNA-mediated knockdown of vimentin inhibited cartilage-specific ECM production [27]. However, MPCs upon chondrogenic induction in monolayer cultures for 28 days expressed chondrocyte markers (ACAN, COL2-α1, SOX9, TGF-β3, and VIM) and also formed typical Alcian blue stained spheroids. The higher relative expression of these markers further confirmed the chondrogenic lineage differentiation of MPCs in a monolayer culture [9,12].
CONCLUSIONS
The results of the present in vitro study indicated that MPCs exist in the articular cartilage of OA patients possessing stem cell-like characteristics with enhanced ability towards chondrogenesis. Stem cell-based therapy might be a promising approach for treating the articular cartilage defects caused due to noncurable diseases, such as OA. However, studies are warranted to further establish the potency and plasticity of MPCs under in vivo conditions using suitable animal models prior to their applications in cartilage regeneration therapy.
ACKNOWLEDGEMENT
This research was funded by Nitte (Deemed to be University) Mangaluru, India.
AUTHOR CONTRIBUTIONS
ABM and SS were involved in conception and design of the experiments. ABM and SR contributed to perform the experiments. ABM and VS analyzed data. ABM and MKB contributed to drafting the article. SSM and ASA contributed to revising it critically for important intellectual content. SSM and MKB made the final approval of the version to be published.
CONFLICTS OF INTEREST
There is no conflict of interest among the authors.
References
- [1]Sophia Fox AJ, Bedi A, Rodeo SA. The basic science of articular cartilage: structure, composition, and function. Sports Health. 2009;1(6):461-468.
- [2]Alsalameh S, Amin R, Gemba T, Lotz M. Identification of mesenchymal progenitor cells in normal and osteoarthritic human articular cartilage. Arthritis Rheum. 2004;50(5):1522-1532.
- [3]Zhang S, Hu B, Liu W, et al. Articular cartilage regeneration: The role of endogenous mesenchymal stem/progenitor cell recruitment and migration. Semin Arthritis Rheum. 2020;50(2):198-208.
- [4]Chagin AS, Medvedeva EV. Regenerative medicine: Cartilage stem cells identified, but can they heal?. Nat Rev Rheumatol. 2017;13(9):522-524.
- [5]Fellows CR, Williams R, Davies IR, et al. Characterisation of a divergent progenitor cell sub-populations in human osteoarthritic cartilage: the role of telomere erosion and replicative senescence. Sci Rep. 2017;7:41421.
- [6]Man GS, Mologhianu G. Osteoarthritis pathogenesis – a complex process that involves the entire joint. J Med Life. 2014;7(1):37-41.
- [7]Baghaban Eslaminejad M, Malakooty Poor E. Mesenchymal stem cells as a potent cell source for articular cartilage regeneration. World J Stem Cells. 2014;6(3):344-354.
- [8]Neogi T. The epidemiology and impact of pain in osteoarthritis. Osteoarthritis Cartilage. 2013;21(9):1145-1153.
- [9]Lee WY, Wang B. Cartilage repair by mesenchymal stem cells: Clinical trial update and perspectives. J Orthop Translat. 2017;9:76-88.
- [10]Dominici M, Le Blanc K, Mueller I, et al. Minimal criteria for defining multipotent mesenchymal stromal cells. The International Society for Cellular Therapy position statement. Cytotherapy. 2006;8(4):315-317.
- [11]Koelling S, Kruegel J, Irmer M, et al. Migratory chondrogenic progenitor cells from repair tissue during the later stages of human osteoarthritis. Cell Stem Cell. 2009;4(4):324-335.
- [12]Jiang Y, Tuan RS. Origin and function of cartilage stem/progenitor cells in osteoarthritis. Nat Rev Rheumatol. 2015;11(4):206-212.
- [13]Sandrasaigaran P, Algraittee SJR, Ahmad AR, Vidyadaran S, Ramasamy R. Characterisation and immunosuppressive activity of human cartilage-derived mesenchymal stem cells. Cytotechnology. 2018;70(3):1037-1050.
- [14]Xue K, Zhang X, Gao Z, Xia W, Qi L, Liu K. Cartilage progenitor cells combined with PHBV in cartilage tissue engineering. J Transl Med. 2019;17(1):104. Published 2019 Mar 29.
- [15]Schultz MB, Sinclair DA. When stem cells grow old: phenotypes and mechanisms of stem cell aging. Development. 2016;143(1):3-14.
- [16]Liu J, Ding Y, Liu Z, Liang X. Senescence in Mesenchymal Stem Cells: Functional Alterations, Molecular Mechanisms, and Rejuvenation Strategies. Front Cell Dev Biol. 2020;8:258.
- [17]Bertolo A, Baur M, Guerrero J, Pötzel T, Stoyanov J. Autofluorescence is a Reliable in vitro Marker of Cellular Senescence in Human Mesenchymal Stromal Cells. Sci Rep. 2019;9(1):2074.
- [18]Bellotti C, Capanni C, Lattanzi G, Donati D, Lucarelli E, Duchi S. Detection of mesenchymal stem cells senescence by prelamin A accumulation at the nuclear level. Springerplus. 2016;5(1):1427.
- [19]Štefková K, Procházková J, Pacherník J. Alkaline phosphatase in stem cells. Stem Cells Int. 2015;2015:628368.
- [20]Kim YH, Yoon DS, Kim HO, Lee JW. Characterization of different subpopulations from bone marrow-derived mesenchymal stromal cells by alkaline phosphatase expression. Stem Cells Dev. 2012;21(16):2958-2968.
- [21]Jayasuriya CT, Hu N, Li J, et al. Molecular characterization of mesenchymal stem cells in human osteoarthritis cartilage reveals contribution to the OA phenotype. Sci Rep. 2018;8(1):7044.
- [22]Mazor M, Cesaro A, Ali M, Best TM, Lespessaille E, Toumi H. Progenitor Cells from Cartilage: Grade Specific Differences in Stem Cell Marker Expression. Int J Mol Sci. 2017;18(8):1759.
- [23]Pretzel D, Linss S, Rochler S, et al. Relative percentage and zonal distribution of mesenchymal progenitor cells in human osteoarthritic and normal cartilage. Arthritis Res Ther. 2011;13(2):R64.
- [24]Arai F, Ohneda O, Miyamoto T, Zhang XQ, Suda T. Mesenchymal stem cells in perichondrium express activated leukocyte cell adhesion molecule and participate in bone marrow formation. J Exp Med. 2002;195(12):1549-1563.
- [25]Su X, Zuo W, Wu Z, et al. CD146 as a new marker for an increased chondroprogenitor cell sub-population in the later stages of osteoarthritis. J Orthop Res. 2015;33(1):84-91.
- [26]Jiang Y, Cai Y, Zhang W, et al. Human Cartilage-Derived Progenitor Cells From Committed Chondrocytes for Efficient Cartilage Repair and Regeneration. Stem Cells Transl Med. 2016;5(6):733-744.
- [27]Steward AJ, Wagner DR, Kelly DJ. The pericellular environment regulates cytoskeletal development and the differentiation of mesenchymal stem cells and determines their response to hydrostatic pressure. Eur Cell Mater. 2013;25:167-178.