Hemostatic activities of methanol leaf extracts of Croton megalocarpus Hutch and Lantana camara Linn
Abstract
The effects of Croton megalocarpus Hutch and Lantana camara Linn plants leave on blood coagulation has not been scientifically tested despite their common use in bleeding control. The current study investigates the effects of the plants’ leaves extracts on mice plasma coagulation time. Leaves of C. megalocarpus (H.) and L. camara (L.) were harvested, washed, air dried, crushed, extracted in absolute methanol and concentrated to dry powders. Preliminary qualitative phytochemical screen of both extracts was done. A total of 55 mice plasma samples were mixed with solutions of leaves extracts of both plants, before prothrombin time (PT) and activated partial thromboplastin time (aPTT) were determined. Data was analyzed using one – way ANOVA. Phytochemical screen on separate extracts of C. megalocarpus (H.) and L. camara (L.) revealed the presence of tannins, phenols, cardiac glycosides, and terpenoids. Moreover, C. megalocarpus (H.) extract contained steroids and flavonoids while that of L. camara (L.) had saponins. Solutions of the various concentrations of methanol leave extracts of C. megalocarpus (H.). L. camara (L.) and leaves blend extracts from both plants reduced aPTT and PT significantly (p<0.05) when mixed with normal mice plasma. Significant reduction of aPTT and PT of normal treated plasma was associated with the activity of tannins, phenols, flavonoids and saponins detected in the plant extracts.
INTRODUCTION
Vessels in the vascular network are lined with endothelium whose smooth surface resists the adhesion of blood cells on its surface. There exists an innate emergency system called hemostasis, which utilizes proteins and mucopolysaccharides to minimize blood loss when the integrity of blood vessels is breached due to injury or surgery [1]. Hemostasis involves an elaborate interaction between platelets and proteins leading to the formation of a fibrin clot, sealing the injured site [2]. The mechanism involves two distinct phases: the primary and secondary hemostasis which take place simultaneously [3, 4]. Primary hemostasis results in vasoconstriction at the injury site, arresting blood loss following vascular spasm [3]. Following the activity of Von Willebrand factor, platelets are activated and attracted to the site of injury during primary hemostasis [5]. The activated platelets also bind to each other to form a temporary platelet plug over the bleeding site, occluding the injured surface [3]. Secondary hemostasis results in the production of fibrin fibers over the temporary platelet plug formed during primary hemostasis [4]. The secondary phase of hemostasis involves the coagulation cascade, which is made up of three distinct but interrelated branches; the intrinsic, extrinsic and the common pathways [1, 5].
Each pathway is made up of a group of inactive coagulation enzymes called zymogens [6]. Incase of injury, the exposure of the endothelium leads to sequential activation of initial zymogens in the cascade into serine proteins [6]. The serine proteases formed act as catalysts for the activation of the next zymogen chain, converting it into a serine protein, ultimately resulting in the conversion of fibrinogen into fibrin fibers [2]. Major injuries may overwhelm hemostasis leading to massive blood loss and death [3].
Blood loss control is an important factor in the survival following vascular injury [4]. Between 30 and 40% of trauma related deaths occurs within the first few hours after injury with about 10% of them being preventable [7]. In Kenya, road traffic accidents form 61.7% of the total disease burden [8]. This makes bleeding associated with traumatic injury one of the top ten leading causes of mortality in Kenya [9]. Hemostatic agents control bleeding by enhancing coagulation through the stimulation of fibrin formation or inhibition of fibrinolysis [10]. The ideal hemostatic agent needs to be inexpensive, easy to use, and capable of extended storage in a wide range of temperatures, ability to stop bleeding in 2 minutes and void of mixing or pre-application preparation [5, 11,13, 14]. Currently, no conventional hemostat is perfect [10]. Conventional hemostatic agents range from coagulation proteins to complex carbohydrates [11]. These include platelet sealants, oxidized cellulose, thrombin, fibrin sealants, microfibrillar collagen, and gelatin [11].
Conventional agents are associated with various adverse effects. Hemostats prepared from human sources which include thrombin and fibrin sealants carry risks of transmission of blood-borne viruses. [12, 15]. In addition, products derived from bovine sources are known to elicit immunologic reactions to users [11, 15]. Gelatin swells when applied to bleeding sites, making its use in body cavities to be undesirable [11]. Furthermore, oxidized cellulose was found to form fibrous scars after wound healing [11]. Besides unwanted effects, thrombin-based hemostats like fibrin sealants and microfibrillar collages are expensive to manufacture [11,12, 16]. Currently, the world is experiencing a surge in interest, acceptance and use of herbal remedies, in both developed and developing countries [17]. However, herbal remedies need to be evaluated for their effects to avoid relying on traditional beliefs and assumptions [18].
Traditionally, sap from fresh leaves of Croton megalocarpus (Hutch) is used topically to arrest bleeding and wound healing [19]. Paste from fresh leaves of Lantana camara (Linn) is applied topically to stop bleeding from cuts [20, 21, 22]. Therefore, this study was designed to evaluate the effects of methanol extracts of C. megalocarpus (MECM.) and L. camara (MELC) on plasma coagulation. MECM and MELC extracts were both found to reduce coagulation time of mice plasma involving both intrinsic and extrinsic pathways ex vivo.
MATERIALS AND METHODS
Extract preparation
Guided by folk literature, fresh leaves of C. megalocarpus (H.) and L. camara (L.) were collected with help of local herbalists at Mbooni, Makueni County. The leaves were transported to the study site at Biochemistry laboratories, situated at Kenya Methodist University School of Medicine and Health Sciences, Meru campus. The samples were verified and authenticated by a paratoxonomist. Voucher specimens were deposited at the United States International University – Africa’s School of Pharmacy and Health Sciences herbarium (C. megalocarpus (H.) MUINDI.H/014; L. camara (L.) MUINDI.H/013).
Leaves were washed, air dried under shade for 14 days and milled to fine powders using an electric mill (Christy and Norris Model 8). Separate powders of C. megalocarpus (H.) and L. camara (L.) leaves were weighed using an analytical balance (Citizen Scales, Edison, USA) and placed in separate beakers then mixed with absolute methanol (ratio of 150 grams of powder to 1.5 liters of methanol) and allowed to extract for 48 hours with agitation. Leaves from both plants were blended (75g of C. megalocarpus (H.) powder and an equal weight of L. camara (L.) powder) before extraction in 1.5 liters of absolute methanol. Following filtration with Whatman filter paper number 1, the extracts were then concentrated by use of a rotary evaporator (Stuart RE400, Stuart, Germany) at 45oc under pressure to dry powders.
Extracts and control preparation
Powders of MECM and MELC extracts were weighed using an analytical balance (Citizen Scales, Edison, USA) and then dissolved in distilled water to make concentrations of 0.25, 0.5 and 1 mg/ml. A volume of 0.1 ml of dimethyl sulfoxide (DMSO) was added to each extract solution to enhance solubility. An aqueous solution of the reference drug (StopsBleeding™, CoAg Medical, Minnesota, USA) was prepared to a concentration of 0.5 mg/ml. Normal control was prepared by adding 0.1ml of DMSO to 4.90 ml of distilled water.
Plasma sample collection and preparation
The approval for use of laboratory animals for the research was obtained from the National Commission for Science, Technology, and Innovation (Reference number NACOSTI/P/20/4729). Fifty-five adult (4-month-old), laboratory-bred Swiss white albino mice, weighing between 200 and 300 grams were obtained. They were allowed to acclimatize in the laboratory for 1 week with unlimited access to water and rodent pellets. Blood was collected from all mice by cardiac puncture into labelled plastic tubes containing sodium citrate anticoagulant (Vacutainer, BD), making a ratio of 9:1. Samples were immediately centrifuged for 5 minutes at 7000rpm using Eppendorf 5427R refrigerated centrifuge (Eppendorf AG, Germany). Plasma was separated into cryogenic plastic tubes and stored at -25oC awaiting evaluation.
Experimental design
The study used a randomized controlled ex vivo experimental design where plasma of 55 mice were randomly placed into 11 treatment groups with 5 samples each. Prothrombin Time (PT) and activated Partial Thromboplastin Time (aPTT) tests were used to evaluate hemostatic effects of MECM, MELC and MECM – MELC blend leaves extracts and controls on treated mice plasma following standard method [24].
Group I (normal control) plasma samples were mixed with distilled water (vehicle) and dimethyl sulfoxide. Groups II (positive control) plasma samples were mixed with the reference hemostatic drug (StopsBleeding™), at a concentration of 0.50 mg/ml. Groups III, IV and V samples were mixed with MECM extract solutions at concentrations of 0.25, 0.50 and 1 mg/ml respectively. Groups VI, VII and VIII samples were mixed with MELC extract solutions at concentrations of 0.25, 0.50 and 1 mg/ml respectively. Groups IX, X and XI samples were mixed with blend methanol leaves extracts of MECM-MELC extract at concentrations of 0.25, 0.50 and 1 mg/ml respectively as shown in table 1.
Table 1. Experimental design for the determination of hemostatic effects.
Effects of extracts on prothrombin time
On the Start4 coagulometer user menu, PT test mode was selected, and the sample identification number entered. A set of three test cuvettes were obtained and analysis metal balls added. A volume of 50 µL of the respective extract/ control solution was added into three cuvettes containing analysis metal balls [24]. A similar volume of platelet poor plasma was then added and mixed with the extract/ control. The mixture was incubated for 5 minutes at 37oC in Start 4 (Diagnostica Stage, France) coagulometer incubation channels [24]. Pre-incubated Neoplastine Cl Plus (100 µL) was added using the equipment timer-linked pipette then coagulation detected automatically, and results printed. The average from the three test runs for each sample was calculated and recorded [24].
Effects on activated partial thromboplastin time
aPTT test mode was selected by navigating Start4 coagulometer user menu and sample identification number entered. Analysis metal balls dispensed into three test cuvettes. A solution of respective sample/control solution (50 µL) was added into the test cuvettes [24]. A volume of 50 µL of respective platelet-poor plasma was then added to the extract solution and mixed well and incubated for 5 minutes at 37oC in the incubation channels [24]. Pre-incubated PTT reagent (PTT Automate) was added and incubated for 180 seconds. The equipment’s timer-linked pipette was used to deliver 50 µL of 0.025M calcium chloride into the reaction to the reaction cuvettes [24]. Clot formation was detected automatically by the equipment and results printed. The average for the three test runs for each sample was calculated.
Statistical analysis
Data was transferred to Microsoft Excel then exported to Minitab version 21 (MinitabLLC, Pennsylvania, USA). The data was subjected to descriptive statistics and expressed as Mean ± SEM, analyzed using One-way ANOVA followed by Tukey’s post-hoc test for pairwise comparison of means. The values of p≤ 0.05 were considered significant. Results were presented using bar charts.
RESULTS
Methanol extraction yield
C. megalocarpus (H.) leaves yielded 17.3% of methanol extract. Leaves of L. camara (L.) produced 12% yield after extraction.
Ex vivo effects of leaves extract on prothrombin time in normal mice plasma
MECM and MELC extracts at 0.25, 0.5 and 1 mg/ml concentration reduced PT significantly (p<0.05 compared to the normal controls as shown in Figure 1. At all the levels evaluated, extracts showed a dose dependent activity where higher concentration was found to show a lower PT (p<0.05).
The effects of MECM, MELC extracts and MECM-MELC blend were not significantly different (p=0.112) at 0.25 mg/ml concentration (Figure 2). The same trend was noted in the effects of MECM at the extract concentration of 0.5 mg/ml, compared to the blended leaves extract at a similar concentration, where the activity was not statistically different (p=0.083) a similar concentration. At 0.5 and 1 mg/ml concentration, the activity of MECM and MELC extracts was not significantly different (p=0.104) compared to each other as illustrated in Figure 2.
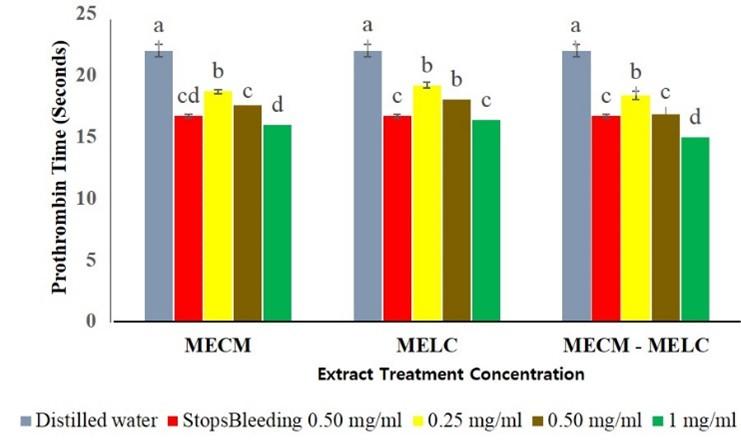
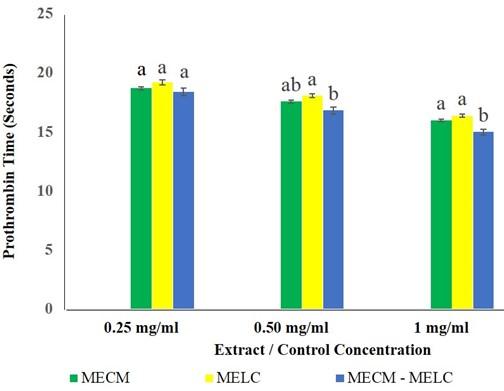
Ex vivo effects of leaves extract on activated partial thromboplastin time in normal mice plasma
MECM, MELC extracts and the MECM – MELC blend caused a significant shortening of activated Partial Thromboplastin Time ex vivo compared to the negative control, as illustrated in Figure 3. The effects of the extract on aPTT were dose dependent, where the highest concentration had a higher reduction in coagulation time (p<0.05; Figure 3).
The effect of the blend extract on aPTT was significantly higher (p<0.05) compared to separate extracts of the same plants across all concentrations studied as illustrated in Figure 4. At lower extract concentrations (0.25 and 0.5 mg/ml) the effects of MECM and MELC extracts on aPTT were, however, not significantly different (p>0.092). In contrast, at 1 mg/ml concentrations, the MELC extract had more activity than that of MECM (p<0.05; Figure 4).
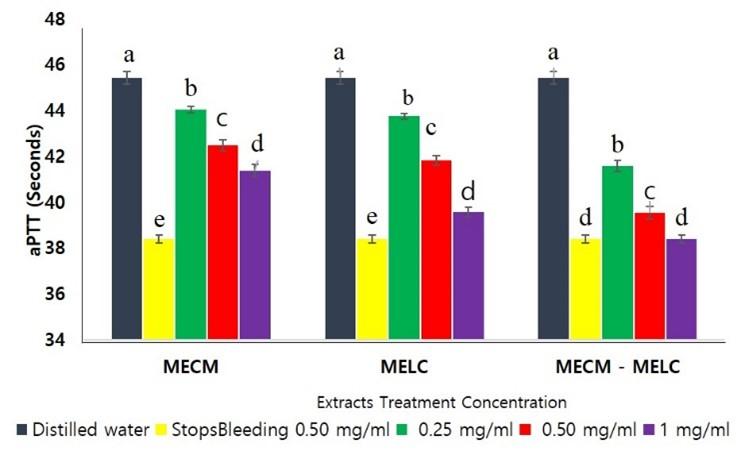
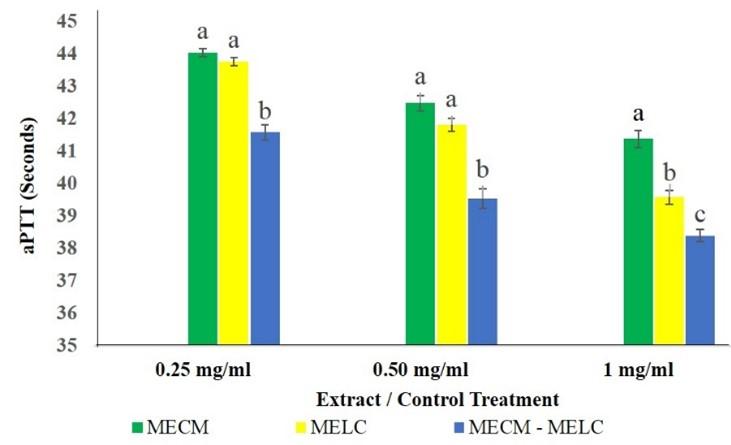
Phytochemical analysis of leaves extract
Qualitative phytochemical analysis on MECM extract revealed the presence of tannins, phenols, steroids, flavonoids, cardiac glycosides, terpenoids, reducing sugars and carbohydrates. MELC extract was found to have saponins, tannins, phenols cardiac glycosides, terpenoids, reducing sugars and carbohydrates as listed in table 2.
Table 2. Phytochemical Screen of methanol extracts of C. megalocarpus (H.) and L. camara (L.).
DISCUSSION
Overall, leaves extract of MECM and MELC and those of MECM – MELC blend demonstrated a significant reduction of the PT and aPTT of treated plasma. This is an indication of hemostatic activity involving extrinsic, intrinsic and common pathways of blood coagulation. The activity of the individual extracts of both plants on PT was noted to be the same at similar concentrations. However, MELC extract demonstrated higher activity on aPTT compared to MECM at 1 mg/ml concentration.
The effects of the plant extracts evaluated in this study could be attributed to phytochemicals detected in the qualitative screen. Literature identifies tannins, phenols, flavonoids, and serine proteases as some of the phytochemicals linked to a reduction of PT and aPTT in treated plasma [25]. Additionally, saponins are associated with the shortening of plasma coagulation time involving aPTT [25]. Saponins isolated from Paris forgesii plant were associated with a reduction of prothrombin time in normal plasma [26]. The presence of saponins and phenols in plant extracts is associated with their reduction of PT in normal plasma [27, 28]. In study involving Chromolaena ordata, it was noted that flavonoids and tannins had blood clotting properties, leading to acceleration of plasma coagulation [29].
Astragalin, a flavonoid isolated from an extract of Rosa chinesis (J.) flowers, caused significant shortening of PT compared to the model group [30]. A similar finding was made in evaluation of kaempferol and phloridzin, (both flavonoids) isolated from Malus pumila Mill flower extract, where the compounds shortened normal plasma PT [31]. Gallic acid and Vanillin acid, (both phenols) isolated from Sedum aizoon (L.) leaves extracts, were associated with a reduction in plasma Prothrombin Time [27]. Phloretin (a phenol) isolated from Malus pumila Mill flowers extract reduced PT of treated plasma [31]. Phenols and flavonoids are also associated with the reduced coagulation time affecting the extrinsic pathway [32, 33].
Gallic acid and Vanillin acid (both phenols), isolated Sedum aizoon (L.) leaves extract were linked with reduction of plasma aPTT and Thrombin time [27]. On the other hand, phloretin, (a phenol) isolated, from Malus pumila M. flower extract was found to reduce aPTT of treated plasma significantly [31]. Serine glycoprotease isolated from Cucumis savitus L. fruit extract reduced aPTT of treated plasma significantly [36]. Furthermore, saponins are generally associated with reduced blood clotting time when applied topically [32]. In a study involving Paris polyphylla V., the reduction in aPTT in normal plasma was attributed to saponins present in the extract [37].
Likewise, tannins have been found to reduce aPTT as demonstrated by activities of Mirabilis jalapa and Paris polyphylla V. extracts, in normal rabbit plasma [37, 38]. Strong complexes formed when tannins are mixed with proteins are associated with the phytochemical’s ability to shorten coagulation time [39]. In a study conducted by Yin et al. (2018), kaempferol and phloridzin, (both flavonoids) isolated from Malus pumila M. flower extract decreased aPTT of treated plasma significantly [31]. In addition, Chalcone (X), a flavonoid, separated from Oxytropis falcate extract reduced plasma re-calcification time significantly [40].
Saponins, detected in MELC extract are postulated to mediate the aggregation of thrombin and fibrinogen during hemostasis, a mechanism that leads to shorter PT and aPTT coagulation time [25]. Flavonoids (found in MECM extract) shorten PT and aPTT coagulation time by participating in the direct conversion of factor X to Xa, bypassing the time-consuming cascade within the intrinsic and extrinsic pathways [43]. Tannins (revealed in both extracts) are postulated to reduce PT and aPTT of treated plasma by enhancing the function of fibrinogen in hemostasis by increasing its potency [42]. Phenols (found in both extracts) believed to shorten plasma coagulation time by facilitating the conversion of factor X to Xa in the common pathway, thereby reducing PT and aPTT [43].
The MECM-MELC blend extract had an enhanced activity lowering the PT and aPTT of mice plasma significantly at the highest evaluated concentration compared to individual extracts at similar concentrations. This is an indication of an additive synergistic effect, which could be linked to an increasing pool of active compounds and a possible interaction among hemostatic phytochemicals. In a study involving ethanolic flower extracts of Bauhinia acuminate, the presence of flavonoids, saponins, and phenols created synergistic effects on hemostasis [41]. In a similar study, blend leaves extract of Vernonia amygdalina and Chromolea ordata caused a significant reduction of PT in Wistar rat plasma [34]. In a study on synergistic effects of honey and Nigella sativa extract, there was enhanced activity when blended compared to the control. This is attributed to the combined pool of flavonoids, phenolic acids, enzymes and vitamins which are known to improve wound healing [35].
CONCLUSION
The findings of this study indicate that leaf extracts of C. megalocarpus (H.) and L. camara (L) can be used to control peripheral bleeding. This study recommends that further study be conducted on the effects of fractions of methanol extracts of both plants on plasma coagulation, bleeding time and wound healing properties. L. camara (L.) and C. megalocarpus (H.) plants have also been identified as candidates for future drug development studies.
ACKNOWLEDGEMENT
This research received no external funding. We acknowledge Kenya Methodist University, School of Medicine and Health Sciences for allowing us to use their laboratories and equipment for this study. We also acknowledge United States International University – Africa, School of Pharmacy and Health Sciences herbarium for assistance with plants samples indexing and archiving.
AUTHOR CONTRIBUTIONS
HM and MN were involved in the conception and design of the experiments. HM, CK, and MN contributed to performing the experiments. CK and MN analyzed data. HM contributed to drafting the article. CK and MN contributed to revising it critically for important intellectual content. HM made the final approval of the version to be published.
CONFLICTS OF INTEREST
There is no conflict of interest among the authors.
References
- [1]Austin, (2017). Hemostasis. Medicine, 45 (4), 204 -208.
- [2]Conway, E. (2018). Compliment – coagulation connections. International Journal of Hemostasis and Thrombosis, 29(3), 243 – 251.
- [3]Bain, J., Bates, I., Laffan, M. and Lewis, M. (2011). Practical haematology (11th ed.). London: Elsevier Churchill Livingstone.
- [4]Versteeg, H., Heemskerk, M., Levi, M. and Reitsma, P. (2013). New fundamentals in hemostasis. Physiological Reviews, 93(1), 327–358.
- [5]Camp, M., (2014). Hemostatic agents: A guide to safe practice for perioperative nurses. Association of Perioperative Registered Nurses Journal, 100(2), 131-144.
- [6]Chaudhry, R., Usama, S. and Babiker, H. (2020). Physiology, coagulation pathways. Treasure Island, Florida: StatPearls Publishing.
- [7]Kushimoto, S., Kudo,D. and Kawazae, Y. (2017). Acute traumatic coagulopathy and trauma induced coagulopathy: an overview. Journal of Intensive Care, 5 (1), 1-7.
- [8]Saidi, H., Mutiso, B. K., and Ogengo, J. (2014). Mortality after road traffic crashes in a system with limited trauma data capability. Journal of Trauma Management and Outcomes, 8(4), 4–9.
- [9]Janeway, H., O’reilly. G. Schmachtenberg, F., Khaeva, N. and Wachira, B. (2019). Characterstics of tertiary referral hospital in Kenya. PLoSONE, 14 (7), e0220 – 179.
- [10]Ebrahim, F. Torbati, M., Mohmoudi, J, and Valizadeh, H. (2020). Medicinal plants as potential hemostatic agents. Journal of Pharmacy and Pharmaceutical Sciences, 23(1), 10 – 23.
- [11]Achneck, H., Sileshi, B., Jamiolkowski, R., Shapiro, M., and Lawson, H. (2010). A comprehensive review of topical hemostatic agents efficacy and recommendations for use. Annals of Surgery, 251(2), 217–228.
- [12]Schreiber, M. and Neveleff, D. (2011). Achieving hemostasis with topical hemostats: making clinically and economically appropriate decisions in the surgical and trauma settings. Association of PeriOperative Registered Nurses, 94(5), s1 – s20.
- [13]Wang, J., Lian, P., Yu, O., Wei, J. and Kang, W.(2017). Purification, characterization and procoagulant activity of polysaccharides from Angelica dahuricae roots. Chemistry Central Journal, 11(1), 17.
- [14]Mani, M., Ebenezer, V., and Balakrishnan, R. (2014). Impact of hemostatic agents in oral surgery. Biomedical & Pharmacology Journal, 7(1), 215–219.
- [15]Tompek, A., Gajdhar, A., Dowling, M., Johnson, S., Barie, P., Winchell,R., King, D., Scala,T., Britt,L. and Nelyaran, M.(2020). A comprehensive review of topical hemostatic agents: the good, the bad, and the novel. Journal of Trauma and Acute Care Surgery, 88 (1), pe2 – pe20.
- [16]Behrens, A., Sikorshi, M. and Kofinas (2014). Hemostatic strategies for traumatic and surgical bleeding, Journal of Biomedical Materials Research, 102A, 4182 – 4194.
- [17]Ekor, M. (2014). The growing use of herbal medicines: issues relating to adverse reactions and challenges in monitoring safety. Front Pharmacol, 4, 177.
- [18]Firenzuoli, F., and Gori, L. (2007). Herbal medicine today : clinical and research issues. Evidence-Based Complementary and Alternative Medicine, 4, 37–40.
- [19]Maroyi, A. (2017).Croton megalocarpus Hutch in tropical Africa: phytochemistry, Pharmacology and Medicinal Potential, 11(4), 124 – 133.
- [20]Badola, H., and Pradhan, K. (2013). Plants used in healthcare practices by Limboo tribe in South -West of Khangchendzonga Biosphere Reserve, Sikkim, India. Indian Journal of Traditional Knowledge, 12(3), 355–369.
- [21]Lozano, A., Araújo, E., Medeiros, M. and Albuquerque, U. (2014). The apparency hypothesis applied to a local pharmacopoeia in the Brazilian northeast. Journal Ethnobiology and Ethnomedicine, 10(1), 2 – 18.
- [22]Uprety, Y., Poudel, R., Gurung,J., Chettri, N. and Chaudhary, R. (2016). Traditional use and management of NTFPs in Kangchenjunga Landscape: implications for conservation and livelihoods. Journal of Ethnobiology and Ethnomedicine, 12(1),19.
- [23]Algelaagbe and Osamudiamen (2009). Phytochemical screening for active compounds in Mangifera indica leaves from Ibadan, Oyo State. Plant Sciences Research, 2(1), 11-13.
- [24]Cordier, W., Cromarty, A., Botha, E. and Steenkamp, V. (2012). Effects of selected South African plant extracts on haemolysis and coagulation. Human & Experimental Toxicology, 31 (3), 250–257.
- [25]Wen, F., Chen, T., Yin, H., Lin, J. and Zhang, H. (2019]. In vitro effects on Thrombin of paris saponins and in vitro hemostatic activity evaluation of paris fargesii Van brevipetala. Molecules 24(7), 1420
- [26]Liu, Z., Li, N.,Gao, W., Man, S., Yin, S., and Liu, C. (2012). Comparative study of hemostatic, cytotoxic and hemolytic activities of different speccies of Paris L.Journal of ethnopharmacology 142(1) 789-794.
- [27]Lin, Z., Fang, V., Huang, A., Chen, L., Guo, S. and Cheng, L. (2014). Chemical constrituents from Sedum aizoon and their hemostatic activity. Pharmaceutical Biology, 52(11), 1429 – 1434.
- [28]Kedari, A. and Gupta, R (2017) Evaluation of hemostatic and antimicrobial ability of different parts of plant Tridax procumbens with phytochemical screening. International Journal of Plant, Animal and Environmental Sciences, 7(1), 128- 136.
- [29]Mathew, J. Vazha, P., Sajeshkumar, N. and Jaim, J. (2016). Phytochemical analysis and in vitro hemostatic activity of Mimosa pudica, Hemigraphs colorata and Chromolaena odorata leaf extracts. CIB Tech Journal of Pharmaceutical Sciences, 5 (3) 16 – 34.
- [30]Li, C., Hu, M., Jiang, S., Liang, Z., Wang, J., Liu, Z., Wang, H. and Kang, W. (2020). Evaluation of procoagulant activity and mechanism of astragalin. Molecules, 25(1), 177.
- [31]Yin, Z., Zhang, Y., Zhang, J., Wang, J. and Kang, W.(2018). Coagulatory active constituents of Malus pumila Mill. Flowers. Chemistry Central Journal, 12(1), 1- 7.
- [32]Sinulingga, S., Subandrate, Kesumaputri, B. and Anggraini, G. (2017). Hemostatic effect of ethanol extract of Piper betle, Linn leaves to male mice. Molekul, 12(1), 23 – 29.
- [33]Hasan, H., Raharja, W. (2016). Differences in effectiveness of water steeping from green tea leaf and black tea leaf as hemostasis effect on wound cut-tail mice (musmusculus). Journal of Dentomaxillofacial Science, 1(3), 163-165.
- [34]Matthew, L. and Osime, E. (2019). Haemostatic properties of Vernonia amygdalina and Chromolea ordata leaf exracts using wister rat model. Universa medica, 38(2), 132-138.
- [35]Javadi, S., Hashemi, M., Mohammadi, Y., Mammohammadi, A., Sharifi,A., Makarchian,H. (2018). Synergistic effect of honey and Nigella Sativa on wound healing in rats. Acta Cirurgica Brasileira, 33(6), 518 -523.
- [36]Nafeesa, Z., Shivalingu, B., Neema, K., Achar, R., Venkatesh, B., Hanchinal, V., Priya, B. and Swamy, S. (2017). Procoagulant serine glycoprotease from Cucumis sativas L. action on human fibrinogen and fibrin clot. 3Biotech, 7(2), 96.
- [37]Liu, Z., Li, N., Gao, W., Man, S., Yin, S. and Liu, C. (2012). Comparative study of hemostatic, cytotoxic activities of different species of Paris, L. Journal of Ethnopharmacology, 142(1), 789 – 794.
- [38]Alam, M., Shamim, S., Ali, S., and Masood, S. (2012). Studies to evaluate the effect of seeds of Mirabilis Jalapa on blood clotting and bleeding time in rabbit. Pakistan Journal of Medicl and Health Sciences, 6(3), 637- 639.
- [39]Chamara, A. and Thiripuranathar, G. (2020). Assesment of hemostatic activity of medicinal plants using in vitro methods: a concise review. Journal of Pharmacy and Biological Sciences, 15(1), 26-34.
- [40]Zhang, M., Jiang, K., Yang, J and Shi, Y. (2019). Flavonoids as the key bioactive components of Oxytropsis falcata Bubge, a Traditional anti-inflammatory and analgesic tibetan medicine. Natural Product Research, 34(23), 3335-3352.
- [41]Biwas, P. Panda, S. and Pani, S. (2019). Phytochemical Screening and evaluation of wound healing potential of ethanolic leaf extracts of Bauhinia acuminata L. in wistar rats. International Journal of Pharmacognosy. 6(6), 224- 227.
- [42]Deng, L., Qi, Y., Liu, Z., Xi, Y.and Xue, W. (2019).Effect of tannic acid on blood components and function, Colloides and surfaces B. biointerfaces 184 110505
- [43]Omar G., Abdallah, L., Barakat, A., Othman, R., and Bourinee, H. (2020). In vitro hemostatic efficiency of aqueous, methanol and ethanol plant extracts of three medicinal plant species in Palestine. Brazillian Journal of Biology 80(4) 763 – 768.