Lactoferrin: potential functions, pharmacological insights, and therapeutic promises
Abstract
Lactoferrin (LF) is an iron-binding multifunctional glycoprotein, act as a natural protective agent. In general, LF is involved in various physiological activities, including antibacterial, antifungal, antiviral, antiparasitic, anticarcinogenic and iron metabolism. The LF is most frequently found in milk as well as many other exocrine secretions such as saliva, bronchial mucus, seminal fluids, and gastro-intestinal fluids, respectively. Increased expression and secretion of LF may play a significant role in the first line of host defense. One of the primary functions of LF is to scavenge free iron in fluids and inflamed areas to avoid free radical-facilitated damage. LF influences the proliferation, maturation, and activity of immune cells at the cellular level. LF plays a significant protective role in inflammation, oxidative stress, fibrosis, endoplasmic reticulum (ER) stress, autophagy dysfunction, and mitochondrial dysfunction. Also, LF was found protective against various pathologies including anemia, sepsis, and diarrhoea in clinical settings. This article reviews the protective role of LF against different pathophysiological conditions and its therapeutic advances as well as further research prospects.
INTRODUCTION
Lactoferrin (LF) is a glycoprotein and a part of the transferrin family, formerly known as lactotransferrin. These proteins conjugate and transfer Fe3+ ions [1]. LF is one of the essential components of the body’s immune system, primarily a member of mucosal innate defenses [2]. LF is a component of the iron-resistant immune system incorporated in vertebrate species to detoxify and sequester toxic metals [3]. LF was first isolated from bovine milk by Sorensen and Sorensen in 1939. In 1960, it was simultaneously determined by three independent laboratories as the main iron-binding protein in human milk with a higher concentration in colostrum (range 6–7 g/L) than in mature milk (range 2 g/L) [1][4]. LF existing in mammalian milk, tears, saliva, cerebrospinal fluid, and other excretory fluids [4] is a 78-kDa glycoprotein, folded into two globular lobes with a single amino acid chain. Each lobe is connected to a 3-kDa glycan chain via an N-glycosidic linkage. These lobes bind to each of the iron-binding sites firmly with the active residue being two tyrosines, a histidine, and an aspartate [3]. LF is excreted from the exocrine glands and identified in particular granules of neutrophils. At the end of degranulation, neutrophils are the primary source of LF in blood plasma.
LF provides non-specific protection against pathogens and other pathologies due to its antimicrobial, anti-inflammatory, and anticancer properties, and it is an important component of mammalian innate immunity [5]. It has broad spectrum of biological functions, including cell proliferation and differentiation, iron metabolism, antiparasitic, antifungal, antiviral, and antibacterial activities [1]. LF played an important role in defense mechanisms by the chelating of iron. Since bacteria require iron to grow, LF can inhibit them by chelating it [6]. Inflammation is a vital part of the host’s protection against bacterial infection. Seeking new therapeutics is important in the fight against infectious diseases. A milk derivative known as bovine LF (bLF) has recently been discovered to be an effective regulator of iron and inflammatory homeostasis, with a strong effect on reducing inflammatory host responses [7]. Furthermore, several studies have reported the antioxidant effect of LF, and binding affinity of LF to the cells, which limit the membrane lipid peroxidation process because LF is not fully saturated and can scavenge free iron radicals that are cytotoxic activators of the lipid peroxidation and oxidative stress and suppresses free radical-mediated damage [8]. LF, a nutraceutical protein, is implicated in certain immunogenic processes and has been suggested to play a part in neurodevelopment and neuropathy [9]. Recently, LF has been suggested as potential preventative and adjunct treatment for COVID-19 [10]. These observations of LF indicate that it may have significant therapeutic potential, and this review was intended to deliver an insight of scientific information on this biomolecule and its activities.
METHODS
The literature was collected by searching the published research articles from PubMed, Google Scholar, and Scopus. To conduct the searching, we used several keywords such as LF vs “structure, sources, functions, inflammation, oxidative stress, fibrosis, endoplasmic reticulum stress, autophagy dysfunction, and mitochondrial dysfunction”. Also, we used ClinicalTrials.gov to find out recent clinical trials on LF. Figures were generated using BioRender.com.
POTENTIAL FUNCTIONS OF LACTOFERRIN
LF exists in biological liquids such as milk, seminal fluid, and saliva [6]. It also exists on mucosal surfaces as well as in some granules found in polymorphonuclear leukocytes. Human milk, as well as bovine milk, are the most plentiful source of LF. LF concentrations in milk vary significantly from one lactation period to another. LF concentrations in colostrum varies in different species with humans having 5.80 ± 4.30 mg/mL [11], bovine0.82 ± 0.54 mg/mL [12], goat 0.39 ± 0.07 mg/mL [13], camel0.81 ± 0.31 mg/mL [14] of LF, respectively. Additionally LF can also be found in different concentrations in human milk 2.00 – 3.30 mg/mL [11], bovine milk 0.03 – 0.49 mg/mL [6], goat milk0.17 – 0.59 mg/mL [15], camel milk 0.06 – 0.89 mg/ml [14], and human tears1.13 ± 0.29 mg/mL [16], respectively.
Structure
LF is comprised of a glycoprotein-rich polypeptide chain with a weight of approximately 78 kDa. Detailed structural studies have shown that in the human and bovine LF, there are respectively 691 and 696 amino acids [17][18]. LF has an analogous sequence of amino acids from mammalian species. Bovine and human LF have a similarity in structure of about 70 percent, and chimpanzee and human LF share nearly 97 percent [19]. Human and bovine LFs amino acids (AA) composition and secondary structural elements are represented in Table 1.
The protein sequence of LF present in human and bovine was collected from the UniProtKB server (https://www.uniprot.org/) with the accession IDs of P02788, and B9VPZ5, respectively. The ProtParam server (https://web.expasy.org/protparam/) and the NPS-SOPMA server (https://npsa-prabi.ibcp.fr/cgi-bin/npsa_automat.pl?page=/NPSA/npsa_sopma.html) were executed to calculate the amino acid composition and the secondary structural elements, accordingly.
Table 1. The composition of amino acids and the secondary structural elements of Human (Homo sapiens) and Bovine (Bos taurus) LF.
Functions
In this section, we have summarized various function of LF as below and in the Figure 1.
Iron Metabolism
Iron is a cofactor for vital enzymes involved in many fundamental cell functions and metabolic pathways. Iron insufficiency can lead to reduced immunity and fatigue [20][21]. LF has an excellent iron affinity with a constant balance dissociation (KD) of approximately 10-20 and is required to maintain a regular iron harmony and eliminate iron deficiency and iron overload. In the presence of two molecules of carbonate ions (CO32-), two ferric ions (Fe3+) can bind with one LF molecule [22], thus acting as an iron transporter in the iron recycling process, which is why numerous studies demonstrated therapeutic benefits and the nutritional advantages of LF [23][20][24]. Oral delivery of bovine LF is effective in preventing anemia in pregnant women [25]. Therefore, LF can be a significant iron source for people with iron deficiency [26].
Antibacterial properties
Numerous widely diversified LF-sensitive pathogens have proved to have bactericidal and/or bacteriostatic impact [27]. One of the factors that underlie LF’s bacteriostatic behavior is its ability to bind vast quantities of iron and inhibit its use for development by microorganisms [28].The bactericidal function of LF occurs predominantly by direct contact with bacterial surfaces by interfering with the lipopolysaccharide (LPS) lipid A and its eventual neutralization by which the Gram-negative bacterial membrane’s permeability may be impaired [28]. LF also has bactericidal and bacteriostatic properties and has a wide range of inhibitions, especially in Bacillus stearothermophilus [29], Staphylococcus aureus [30], Bacillus subtilis [31], Streptococcus parasanguinis [32] of gram-positive bacteria; and Chlamydophila psittaci [33], Haemophillus influenzae [34], Vibrio cholerae [35], Mycobacterium tuberculosis [36][37], Samonella enteritidis [38] of gram-negative bacteria. By interacting with LPS, LF can conflict with bacterial attachment and thus prevent one of the vital virulence effects of these microorganisms [39][40][27].
Antifungal properties
The broad array of LF antifungal activity against mold and yeast is now well documented [41][42].Owing to their powerful iron (Fe3+) scavenging effects, LF and its related peptides can effectively function on a diverse array of fungal organisms. LF has been reported to have antifungal potentials against Candida krusei, Candida albicans, and Aspergillus fumigatus [43][44]. In addition to its iron-depriving activity, LF attaches to the cell’s fungal surface followed by destroying the layer, and enhances the cell membrane’s permeability, resulting in death of the organism. Because of these attributes, LF is included in a variety of antifungal therapies, such as those required to treat oral candidiasis [45].
Antiviral properties
LF is a broad spectrum antiviral agent that effectively prevents naked and coated DNA and/or RNA viruses from infecting animals and humans [46][47]. The LF domain that functions against viral infections is distinct from that of bacteria. LF bacteriostatic peptide was not observed as being selective for viruses [46], therefore LF antiviral activity is well demonstrated against a broad range of viruses, including herpesvirus, human immunodeficiency virus (HIV), friend virus complex (FVC), human hepatitis B virus (HBV), human hepatitis C virus, parainfluenza virus (PIV), respiratory syncytial virus (RSV), alphavirus, hantavirus, human papillomavirus (HPV), rotavirus, adenovirus, and picornavirus [48].
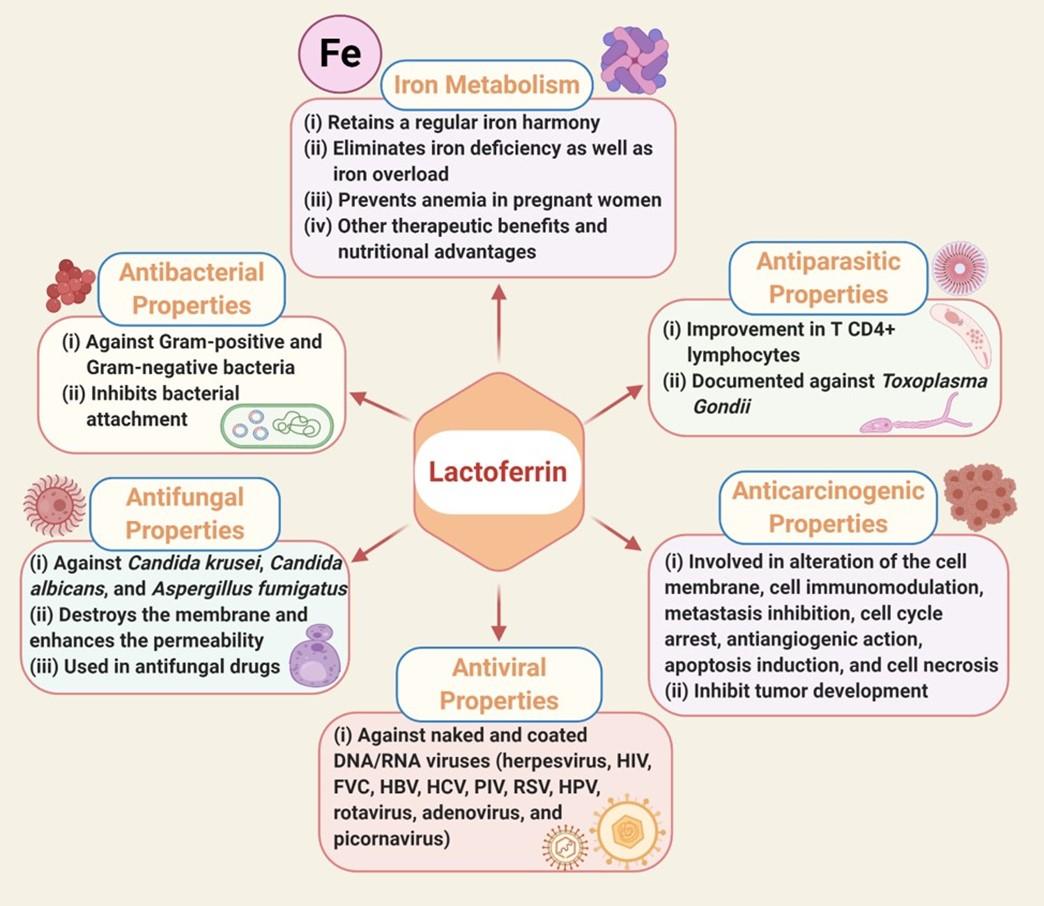
Antiparasitic properties
LF’s antiparasitic commitment is different and varies between species. The noteworthy underlying mechanism in many of these parasites is the sequestration of iron, which plays a prominent part in hosting parasites. Numerous investigations indicated that the T-cell reaction is enhanced as an intervention mechanism [49]. The improvement in T CD4 + lymphocytes after LF is administered to both immunocompetent and immunosuppressed mice before Toxoplasma Gondii infections have been reported. However, on the contrary, many parasites, including Trichomonas vaginalis and Trichomonas fetus use LF for development [28].
Anticarcinogenic properties
LF enforces antineoplastic involvement through various cancer-type response pathways, such as altering the cell membrane, cell immunomodulation, metastasis inhibition, cell cycle arrest, antiangiogenic action, apoptosis induction, and cell necrosis. LF’s chelating involvement is by far the most extensively reported biological mechanism towards tumor development. Oxidative stress (OS) on genetic material is found to increase iron in the tissue, which induces carcinogenesis, and thus, LF prevents this by trapping this ion on the surface of the tissue [50].
PHARMACOLOGICAL POTENTIALS OF LACTOFERRIN AGAINST VARIOUS PATHOLOGICAL CONDITIONS
LF is a cell-secreted mediator that links innate and adaptive immunity in mammals [51]. LF binding affects the target cells in a cellular signaling pathway and activates target genes [52]. The pharmacological potential of LF against various pathological conditions such as oxidative stress, inflammation, fibrosis, endoplasmic reticulum (ER) stress, autophagy dysfunction, and mitochondrial dysfunction (Figure 2).
Inflammation
Inflammation is an adaptive response that involves a wide range of physiological and pathological processes stimulated by noxious stimuli and conditions [53][54]. It is a complex interaction between soluble components and cells in any tissue in reply to traumatic, infectious, post-ischemic, toxic, acute kidney injury or autoimmune injury [55][56][57]. LF is known to be up regulated in inflammatory diseases, including inflammatory bowel disease, allergic skin, lung disorders, neurodegenerative disease, and arthritis [52].
LF acts as a natural regulator of host defense [51] with significant anti-inflammatory activities related to the scavenging of free iron that accumulates in inflamed tissue and catalyzes the production of tissue-toxic hydroxyl radicals, making LF a potent therapeutic candidate in the treatment of common inflammatory diseases [58]. LF is one of the first factors expressed by neutrophils after exposure to pathogens and contributes to innate activation of adaptive responses [51] by releasing active neutrophils into the site of inflammation. The concentration of LF in the blood is usually low(0.2–0.6 g/ml), and may increase significantly due to exposure to neutrophils at the inflammation site and exceed 200 g/ml [59]. It has been demonstrated that LF may inhibit inflammation related to microbial challenge [52] and also been reported to bind to bacterial endotoxin lipopolysaccharides (LPS), a key mediator of the inflammatory response to bacterial infections.
Consequently, the interaction of LPS with receptors is disrupted, and events such as the upregulation of inflammatory cytokines are reduced [60]. In vitro studies have shown LF administration to protect the gut mucosal integrity induced by LPS challenge, gastritis from Helicobacter pylori, and endotoxemia and lethality in response to systemic challenge with E. coli or LPS. Studies on monocytes suggest that LF’s anti-inflammatory activity in response to the challenge of LPS may inhibit pro-inflammatory cytokine synthesis after LF translocation into the nucleus by inhibiting NF-kB activation [52].
Another possible role of LF is removing free iron in inflammatory centers e.g. rheumatoid joints, by preventing the catalysis of harmful free radicals [60]. Rheumatoid arthritis patients often have iron deposits in their synovia which when bound with LF, cannot react with superoxide to produce injurious hydroxyl radicals that cause vital tissue damage in rheumatoid arthritis. LF has been injected intra-articularly into animal models of inflammation and has been reported to inhibit inflammation. Therefore, LF’s local administration and consequence of increased LF in the synovium is initially thought to be anti-inflammatory and may be effective in the treatment of rheumatoid arthritis [59].
Fascinatingly, in neurodegenerative diseases where iron accumulation contributes to oxidative stress and neuronal death, excessive expression of LF in some regions of the brain has been reported [61]. This phenomenon may play a role in limiting oxidative stress in the brain with transcytosis of plasma LF by the blood-brain barrier during inflammation [58][62].
In vivo studies have shown that LF can protect against skin and lung allergies [63][64]. LF is highly expressed in allergic patients [65], a mechanism that contains the activation of mast cells and basophils and IL-1β and TNF-α-triggered translocation of antigen-presenting cells. In skin allergies, a mechanism has been proposed that LF binds to keratinocytes and prevents the release of TNF-α from those cells [66]. LF can reduce cutaneous inflammation by inhibiting the migration of Langerhans cells [60]. In humans and mice, it is shown that LF protects from IL-1β induced cutaneous inflammation and inflammatory bowel disease that chemically influenced. In many cases, this protection was significantly associated with an increase in anti-inflammatory cytokines, including IL-10, and a decrease in pro-inflammatory cytokines, including TNF-α and IL-1β. The capability of LF to interact with specific receptors in several immune cells, including monocytes, macrophages, neutrophils, and in addition to epithelial cells, indicates that the anti-inflammatory activity of LF may be an outcome of a direct effect on modulating cytokine production by these cells via receptor-facilitated signaling pathways [52].
LF is called first-line defense glycoprotein due to its protection system against the progression of systemic inflammatory response syndrome (SIRS) and sepsis. The clinical significance of LF in controlling these processes has been demonstrated through studies based on neonates, where dietary supplementation with LF has reduced the incidence of late-onset sepsis. LF has proven to be a primary innate immune modulator that plays a crucial role in controlling acute septic inflammation [60]. In the case of infection, the systemic monocyte or macrophage reacts with the generation of inflammatory mediators, which then persuades bone marrow to form new immune cells and trigger the degranulation of mature neutrophils. Consequently, a vast amount of LF is released from neutrophil’s secondary granules to fight infection [60]. Thus, the anti-inflammatory effects of LF signify a systematic sequence of events during the development of acute inflammation and could be seen as a further manifestation of the role of neutrophils in inflammation [60][67].
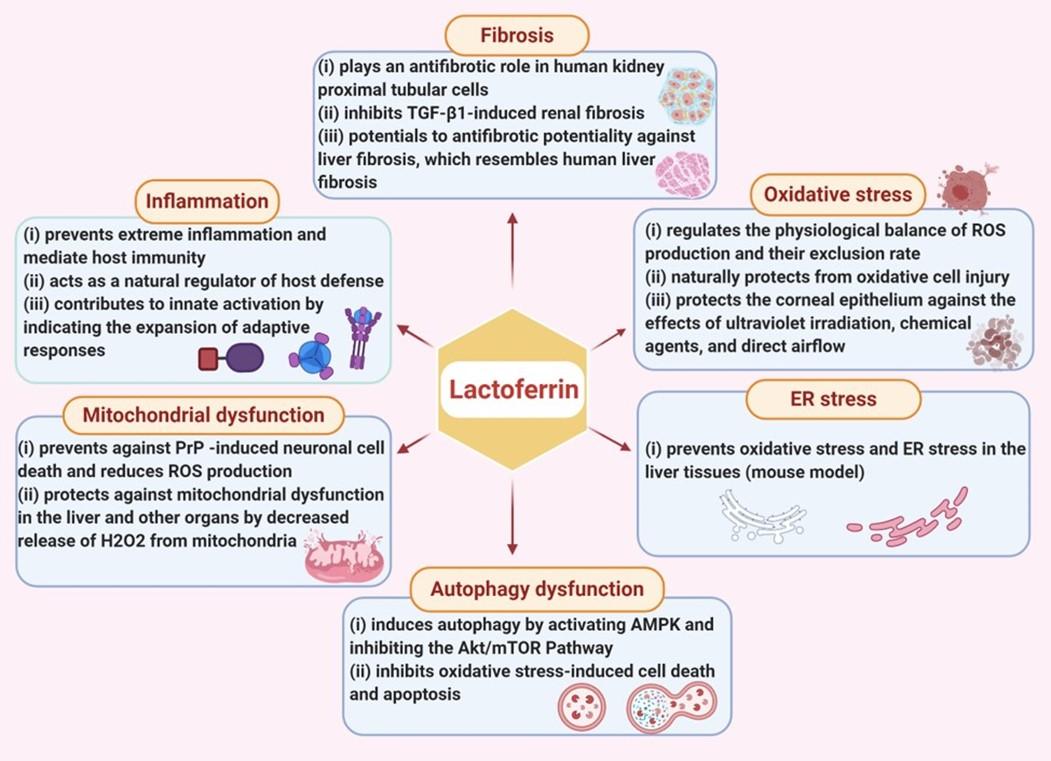
Oxidative stress
An imbalance of pro-oxidants and antioxidants is called oxidative stress, which is portrayed as a disturbance of redox signaling and control mechanisms [68]. Oxidative stress arises when free radical levels exceed the cell’s ability to fight them causing an imbalance between free radical production and the antioxidant defense system [69]. It can occur due to exposure to reactive oxygen mediators, which can damage proteins, nucleic acids, and cell membranes [70]. Free iron radicals are toxic, damaging cellular components, or producing reactive oxygen species (ROS) that are cytotoxic [71]. Antioxidants can reduce oxidative damage and improve life quality by preventing or delaying the onset of degenerative diseases.
bLF, with its iron-binding capability, appeared as antioxidant activity [72] and was considered an antioxidant [73][74][75], and LFs from other animal sources, such as humans [76] and camels [77], showed antioxidant activity too [78]. According to iron sequestration, LF regulates the physiological balance of ROS production and their exclusion rate that naturally protects from oxidative cell injury.
On the other hand, the superoxide radical can undergo a two-step, non-enzymatic degradation process in the presence of free ferric ions (Fe3+) [67]. In the first step, a superoxide molecule responds with ferric ion (Fe3+), generates ferrous salt (Fe2+) and the ground state oxygen. In the second step, ferrous (Fe2+) ions react with hydrogen peroxide generates ferric salt (Fe3+), a hydroxyl radical, and alcohol, which is known as the Fenton reaction [67]. Microbicidal activity is strongly involved in phagocytic and lipid peroxidation events, especially in polyunsaturated fatty acids, to form hydroxyl radicals through an iron-dependent reaction. The reaction of the hydroxyl radical with polyunsaturated fatty acids results in the notion of a hydrogen atom. It begins the lipid peroxidation and the formation of intermediates like hydroxyalkenals. These new radicals can induce functional alterations in many biologically essential macromolecules, including DNA, proteins, and lipids. LF aids in the sequestering of ferric (Fe3+) ions to protect from oxidative stress’s harmful effects [67].
The tissues of the anterior part of the eye are mainly susceptible to oxidative stress, and they have developed numerous antioxidant defense mechanisms to avoid injury. Deficiencies in the antioxidative apparatus and immune systems are involved in various ocular pathologies, for example, keratoconus (KC), dry eye, and Sjögren syndrome [79]. Tear fluid contains antioxidative compounds such as LF, vitamin C, glutathione, and superoxide dismutase (SOD), which protect the corneal epithelium against the effects of ultraviolet irradiation, chemical agents, and direct airflow [79]. The main antioxidant molecules in the cornea are enzymes superoxide dismutase catalase, and glutathione peroxidase. Besides, the synthesis of reactive oxygen and nitrogen species can be noticed in KC tissues. Significantly, the decline of antioxidant molecules was observed in the KC cornea compared to healthy corneas. Besides, LF is a protein with vital antioxidant activity, is present in the tears of high concentration (1.1 ± 0.3 mg/mL vs. 7.0 ± 1.6 mg/mL, total tear protein content). This natural defense against oxidative stress can play a crucial role in preventing such eye disorders progression [79].
Mainly in human medicine, bLF is used as a food additive due to its availability that designated by the U.S. Food and Drug Administration, which is generally recognized as safe [71]. The bLF can regulate the physiological balance of ROS generation and their elimination rate by iron sequestration. Many researchers have proven that bLF is capable of modulating the adaptive immune response and that it has crucial regulatory activity in cellular redox through the uptake of vital antioxidant enzymes [80][81][82][83]. It is shown that oral supplementation of bLF enhances total, helper, and cytotoxic T-cell activation and hydrophilic antioxidant status. The bLF may otherwise be a useful nutritional supplement to support immunity and antioxidant status in healthy individuals [73].
LF can protect human mesenchymal stem cells against oxidative stress-induced senescence and apoptosis [84]. Mesenchymal stem cells (MSCs) have been proposed as a crucial candidate for cell therapy due to their ability to self-regulate and differentiate. MSCs poorly live after exposure to environmental factors such as oxidative stress and endure senescence or apoptosis when transplanted. The clinical application of these cells is still limited due to its survival issue. Therefore, the effectiveness of MSC therapy can be improved by reducing oxidative stress through LF administration. The study revealed that pretreatment of LF is effective against oxidative stress-induced senescence and apoptosis of human MSCs (hMSCs). ROS measurement discovered that LF inhibited hydrogen peroxide-induced senescence of hMSCs by inhibiting caspase-3 and Akt activation [84].
Oxidative stress has also been associated with pregnancy complications. Administration of LF to the vagina reduced the oxidative stress of amniotic fluid (AF) in pregnant women with mid-trimester genetic amniocentesis. So, the stable generation of ROS is crucial for various reproductive processes, from oocyte maturation to fetal development to delivery stimulation [85][86]. Oxidative stress may lead to a variety of pregnancy-related complications directly or indirectly, for example, spontaneous abortion, recurrent miscarriage, preterm labor, and preterm pre-labor rupture of membranes (PPROM) [87]. Vaginal LF administration can control the expression of inflammatory markers and matrix metalloproteinases (MMPs), intensely associated with preterm labor, pre-labor rupture of membranes, and PPROM [88][89][90]. It reduces oxidative stress assessed in pregnant women’s amniotic fluid enduring mid-trimester genetic amniocentesis [91]. As the LF enters into the amniotic sac, it can reduce lipoperoxides production by directly mediating intermediate ROS scavenging or eliminating Fe3+ from the Fenton reaction [92][84]. Transvaginal LF can directly exert an immunomodulatory effect through modulating the in vivo and in vitro ROS generation. LF can limit oxidative stress by interfering with lipid peroxidation products formation. LF can be used as a therapeutic to treat pregnancy-related complications [91].
Fibrosis
Fibrosis is defined as excessive growth, stiffness, or scarring of several tissues and is identified as causing excessive deposition of extracellular matrix components, including collagen. Fibrosis is the final result of chronic inflammatory reactions stimulated by various stimuli, including persistent infections, autoimmune reactions, allergic reactions, chemical insults, radiation, and tissue injuries [93]. LF plays an antifibrotic role in human kidney proximal tubular cells. Excess matrix proteins, fibroblasts accumulation, and nephrons functional damage are the leading pathological features of progressive CKD and the cause of renal fibrosis. Transforming growth factor-β1 (TGF-β1) is an essential mediator in renal fibrosis [94]. Connective tissue growth factor (CTGF) and plasminogen activator inhibitor-1 (PAI-1) are known to be effective inducers of tissue fibrosis. It is documented that TGF-β1 amplifies CTGF, PAI-1, and collagen 1 in a concentration-dependent manner.
To scrutinize the capabilities of LF against fibrosis, stimulated cultured renal epithelial cells (HK-2) with TGF-β1 were observed in the presence or absence of LF to examine whether LF prevents the TGF-β1-induced fibrosis signaling pathway. The investigation exhibited that LF decreased the profibrogenic TGF-β1 target genes PAI-1, CTGF, and collagen I thus indicating that LF inhibits TGF-β1-induced renal fibrosis [94].
Liver fibrosis is the most crucial pathological concern of all chronic liver diseases. Certain drugs, autoimmune disorders, and genetic diseases are the significant causes of liver fibrosis. Antifibrotic therapy can restore the normal functioning condition of the liver. LF has an antiviral effect against a wide variety of viruses, including hepatitis C. LF prohibited hepatocellular necrosis and showed a direct cytoprotective function in the liver. In a recent study on rats using thioacetamide (TAA), a model that revealed LF antifibrotic potentiality against liver fibrosis resembles human liver fibrosis [95].
Aerosolized bovine LF diminishes infection, inflammation, and iron imbalance in a cystic fibrosis mouse model of Pseudomonas aeruginosa mediated chronic lung infection [96]. Chronic airway infections are often sustained by Pseudomonas aeruginosa [97][98]. P. aeruginosa mediated chronic infection associated with decreased lung function as well as increased morbidity and mortality [99]. Along with infection, inflammation, and instability of iron homeostasis in CF airways and high levels of iron (up to >100 µM) in airway secretions has been observed [100][101][102]. Above all, increased expression of ferroportin (Fpn), ferritin (Ftn), and transferrin (Tf) were observed in the lung tissue of CF patients, which together with accumulated high levels of iron in the lower respiratory tract of CF subjects [102][103][104]. Excessive iron in CF airways increases growth and the biofilm lifestyle of P. aeruginosa, thus exacerbates inflammatory conditions and host injury [105][101][106]. LF can chelate two Fe3+ ions per molecule with high affinity, which is synthesized by exocrine glands and neutrophils at infection and inflammation sites. bLF is a milk derivative, with which it shares a high sequence homology of human proteins that looks identical with characteristics [107]. The bLF prevents host cell invasion by specific intracellular attachments or obligate bacterial
pathogens [108][109][110]. It employs a strong anti-inflammatory activity that contributes to protecting mucus from inflammatory damage [108][109][111][112]. Treatment with aerosolized bLF or saline after infection proved that aerosolized bLF effectively reduced bacterial lung load and infiltrated leukocytes in infected CF mice. By reducing pulmonary iron overload in both wild-type (WT) and CF mice, bLF acts as a powerful multi-targeting agent capable of breaking down the cycle induced by P. aeruginosa, inflammation, and iron imbalance, thereby reducing the severity of CF-related pathology [96].
ER stress
The ER is a protein-folding apparatus which is made up of protein chaperones. ER catalyzes protein folding and senses the existence of misfolded or unfolded proteins [113]. Pathological evidences exhibited that ER stress is a common cause of many diseases where the stress is so severe or chronic that cells may die or damage [114]. Cellular dysfunction and cell death often occur when ER stress is prolonged, and a load of proteins in the ER exceeds its folding capacity [115]. Disruption of the ER’s normal functions lead to an evolutionary preserved cell stress response, an unfolded protein response, which is primarily intended to compensate for damage but can ultimately lead to cell death due to severe or chronic ER dysfunction [116].
LF prevents oxidative stress and ER stress in the liver tissues of ob/ob mice. In that experiment, leptin-deficient (ob/ob) mice were used as rodent models of NAFLD (non-alcoholic fatty liver disease). Remarkably, ER stress has been currently recognized as a reason for controlled iron homeostasis via the contribution of hepcidin induced obesity and hepatic lipid accumulation. To evaluate LF’s hepatoprotective effects, recombinant human LF is administered by intraperitoneal injection to alleviate or delay the pathological process of NAFLD. It is shown that the activation of ERK1/2 and eIF2α, like NF-κB activation and oxidative stress, was seemingly blocked in the liver tissues of LF-treated ob/ob mice compared with vehicle-treated ob/ob mice. As a result, it recommends that LF’s cytoprotective role may be related to ER stress prevention. It is indicated that hepatosteatosis-induced ER stress can be prevented by LF administration because the expression levels of hepatic p-eIF2α and p-NF-κB were considerably higher in ob/ob mice than in wild-type (WT) or LF-treated ob/ob mice. It has been shown that LF may be responsible for inhibition of ER stress and progression of autophagy of damaged hepatocytes and induction of up-regulation of hypoxia-inducible factor-1α/vascular endothelial growth factor (HIF-lα/VEGF) to assist liver function recovery due to its cytoprotective role [117].
Autophagy dysfunction
Autophagy is an intracellular degradation and energy recycling system, plays crucial roles in the immune responses and abnormal pathways that have been related to numerous disease conditions [118]. Typically, it is activated in nutritional deprivation conditions through the mTOR and AMPK signaling pathways to improve cell survival [119][120][121]. Autophagy dysfunction is defined as extreme autophagy initiation or block of autophagy flux, which cause possible cell death process, resulting in apoptosis or autophagic cell death [122].
LF induces autophagy by activating AMPK and inhibiting the Akt/mTOR Pathway. Cells were treated with different concentrations of LF and determined that LF augmenting autophagy in HK-2 cells (a human kidney proximal tubular epithelial cell line). It was observed that LF did not cause apparent cell function changes, but high concentrations of LF (200 µg/mL and 400 µg/mL) slightly improved HK-2 cells’ viability. Besides, western blot analysis of autophagy-related proteins predicts that LF induces autophagy [94]. It is reported that levels of beclin-1 and LC3-II were significantly raised in cells treated with LF. Furthermore, determining the percentage of punctate LC3-stained cells by fluorescence microscopy showed that LF treatment caused a concentration-dependent increase in LC3 dots in HK-2 cells. Another previous study also reported that autophagy induction could be regulated by activating AMPK and blocking the Akt/mTOR pathway. LF enhances AMPK phosphorylation and inhibits Akt and mTOR phosphorylation. Moreover, increased beclin-1 and LC3-II expression are caused by forced expression of the exogenous LF gene. These investigations specify that LF induces autophagy by activating AMPK and inhibiting the Akt /mTOR pathway. Augmenting autophagy in HK-2 cells, LF inhibits oxidative stress-induced cell death and apoptosis [94].
Mitochondrial dysfunction
Mitochondria are vital organelles of different cell types that play a significant role in cell survival and apoptotic cell death [123]. The definition of mitochondrial dysfunction is defined as the inability of mitochondria to produce and maintain adequate ATP levels through oxidative phosphorylation in response to energy needs [124][125]. Mitochondrial dysfunction is related to ROS-mediated damage [126][127]. A variety of degenerative diseases occurred due to mitochondrial oxidative injury. In neurodegenerative disorders, oxidative stress-induced neurodegeneration is occurred by ROS generation [128]. Oxidative stress is the leading cause of mitochondrial-mediated apoptotic cell death. Abnormal regulation of mitochondrial dynamic proteins can lead to neuropathological changes in prion disorders due to mitochondrial dysfunction. Neurodegenerative diseases like Prion disorder are caused by the accumulation of prion protein (PrP) scrapie isoform (PrPsc) in the central nervous system. PrPsc mediates neuronal cell death by raising the intracellular production of ROS [126].
LF has antioxidant capability due to the scavenging of ROS. LF treatment prevents against PrP -induced neuronal cell death and reduces ROS production. Decreased ROS production stopped PrP -induced mitochondrial dysfunction. Furthermore, PrP -induced protein activation and c-Jun N-terminal kinase and caspase-3 were inhibited by LF treatment [123]. Significantly, LF protects against mitochondrial dysfunction in the liver and other organs by decreased release of H2O2 from mitochondria. Also, LF protects against the development of insult-induced SIRS and its progression into septic conditions in vivo [129]. LF can diminish oxidative insult at the cellular and tissue levels after lipopolysaccharide exposure through the possible mechanism. Acute inflammatory responses mediated by cell injury and cell death are responsible for SIRS progression into sepsis. Both apoptotic and necrotic cell death is firmly related to mitochondrial dysfunction. It frequently happened due to increased ROS generation, increased membrane permeability, loss of mitochondrion integrity, and cellular ATP levels [129]. LF pretreatment in cultured cells and animal (mice) model of endotoxemia showed decline in LPS-induced elevation of ROS levels, decreased damage to nuclear and mtDNA. Early LF administration may provide comprehensive protection from mitochondrial dysfunction.
It was currently revealed that LF could stop the progression of SIRS into sepsis in endotoxemic mice. LF pretreatment of cells in a dose-dependent manner reduces LPS-mediated oxidative insults. Pretreatment of LF in experimental animals considerably (P<0.05) depressed LPS-induced mitochondrial dysfunction in vivo. Notably, LF can prevent mitochondrial ROS generation and the accumulation of oxidative damage in the DNA. It has been shown that LF pretreatment reduced the release of H2O2 and DNA damage in the mitochondria. Thus, LF can be a potential option for the prevention and treatment of SIRS [129].
CURRENT UPDATE ON CLINICAL TRIALS
Beside preclinical studies, clinical trial with LF is also undergoing (Table 2). A preliminary research indicates that LF may be a potential new oral iron replacement approach [130]. Some trials were performed based on the principal theory that oral bLF may decrease death risks or significant morbidities of preterm infants through its antioxidant, antimicrobial, and anti-inflammatory effects [131][132].
Table 2. Current update on clinical trials of LF.
FUTURE PERSPECTIVES AND CONCLUSIONS
LF is a multifunctional natural iron-binding protein. LF is widely active in different physiological processes, include metabolism of iron, antibacterial, antifungal, antiviral, antiparasitic, and anticarcinogenic functions. One of the primary features of LF is to scavenge free iron in biological fluids and ingrown regions in order to avoid free, easy-to-use, damage and reduce the involvement of metals to assault microbial and neoplastic cells. In other defensive mechanisms, LF plays a vital function, such as antioxidant prevention and protection against different kidney injuries. Also, LF is a cell-secreted mediator that links innate and adaptive immunity in mammals. LF binding affects the target cells in a cellular signaling pathway and activates the target genes. The pharmacological potential of LF against various pathological conditions, including oxidative stress, inflammation, fibrosis, ER stress, autophagy dysfunction, and mitochondrial dysfunction. Also, LF was found potential against various pathologies in clinical settings.
More research is eventually needed to better understand the role of Lf in metabolism, especially in adipose tissues, lipolysis, and hepatic and intestinal lipid metabolism [133]. Study is also required to better understand the molecular features of Lf in cancer localization [134]. This study provides insights into LF’s protective role against various pathological mechanisms or conditions and outlines the updates on its clinical uses.
ACKNOWLEDGEMENT
This work acknowledges National Research Foundation (No. 2020R1I1A1A01072879), and Brain Pool program funded by the Ministry of Science and ICT through the National Research Foundation (No. 2020H1D3A2A02110924), Republic of Korea.
AUTHOR CONTRIBUTIONS
This work is a collaboration among all the authors. MJU and AM designed outlines. KAA and ASMS wrote the initial draft of the manuscript. ASMS prepared the tables and illustrated the figures. MJU, SAMK, AM, KAA, and MRI edited and reviewed the scientific contents described in the manuscript. All authors read and approved the final submitted version of the manuscript.
CONFLICTS OF INTEREST
There is no conflict of interest among the authors.
References
- [1]Adlerova L, Bartoskova A, Faldyna M. Lactoferrin: A review. Vet Med (Praha) 2008;53:457–68.
- [2]Német K, Simonovits I. The biological role of lactoferrin. Haematologia (Budap) 1985;18:3–12.
- [3]Weinberg ED. Human lactoferrin: a novel therapeutic with broad spectrum potential. J Pharm Pharmacol 2010;53:1303–10.
- [4]Liu J, Li B, Lee C, Zhu H, Zheng S, Pierro A. Protective effects of lactoferrin on injured intestinal epithelial cells. J Pediatr Surg 2019;54:2509–13.
- [5]Giansanti F, Panella G, Arienzo A, Leboffe L, Antonini G. Nutraceutical Peptides from Lactoferrin. Adv Dairy Res 2018;6:1–4.
- [6]Cheng JB, Wang JQ, Bu DP, Liu GL, Zhang CG, Wei HY, et al. Factors affecting the lactoferrin concentration in bovine milk. J Dairy Sci 2008;91:970–6.
- [7]Berlutti F, Pilloni A, Pietropaoli M, Polimeni A, Valenti P. Lactoferrin and oral diseases: current status and perspective in periodontitis. Ann Stomatol (Roma) 2011;2:10–8.
- [8]Hegazy R, Salama A, Mansour D, Hassan A. Renoprotective effect of lactoferrin against chromium-induced acute kidney injury in rats: Involvement of IL-18 and IGF-1 inhibition. PLoS One 2016;11:e0151486.
- [9]Iglesias-Figueroa BF, Espinoza-Sánchez EA, Siqueiros-Cendón TS, Rascón-Cruz Q. Lactoferrin as a nutraceutical protein from milk, an overview. Int Dairy J 2019;89:37–41.
- [10]Chang R, Ng TB, Sun WZ. Lactoferrin as potential preventative and adjunct treatment for COVID-19. Int J Antimicrob Agents 2020;56:106118.
- [11]Montagne P, Cuillière ML, Molé C, Béné MC, Faure G. Changes in lactoferrin and lysozyme levels in human milk during the first twelve weeks of lactation. Adv. Exp. Med. Biol., vol. 501, Kluwer Academic/Plenum Publishers; 2001, p. 241–7.
- [12]Kehoe SI, Jayarao BM, Heinrichs AJ. A survey of bovine colostrum composition and colostrum management practices on Pennsylvania dairy farms. J Dairy Sci 2007;90:4108–16.
- [13]Hiss S, Meyer T, Sauerwein H. Lactoferrin concentrations in goat milk throughout lactation. Small Rumin Res 2008;80:87–90.
- [14]Konuspayeva G, Faye B, Loiseau G, Levieux D. Lactoferrin and immunoglobulin contents in camel’s milk (Camelus bactrianus, Campus dromedarius, and Hybrids) from Kazakhstan. J Dairy Sci 2007;90:38–46.
- [15]Chen PW, Chen WC, Mao FC. Increase of lactoferrin concentration in mastitic goat milk. J Vet Med Sci 2004;66:345–50.
- [16]Balasubramanian SA, Pye DC, Willcox MDP. Levels of lactoferrin, secretory IgA and serum albumin in the tear film of people with keratoconus. Exp Eye Res 2012;96:132–7.
- [17]Baker HM, Baker CJ, Smith CA, Baker EN. Metal substitution in transferrins: Specific binding of cerium(IV) revealed by the crystal structure of cerium-substituted human lactoferrin. J Biol Inorg Chem 2000;5:692–8.
- [18]SA M, BF A, CR G, M H, EN B. Three-dimensional structure of diferric bovine lactoferrin at 2.8 Å resolution. J Mol Biol 1997;274:222–36.
- [19]Yount NY, Andrés MT, Fierro JF, Yeaman MR. The γ-core motif correlates with antimicrobial activity in cysteine-containing kaliocin-1 originating from transferrins. Biochim Biophys Acta – Biomembr 2007;1768:2862–72.
- [20]Phaniendra A, Jestadi DB, Periyasamy L. Free Radicals: Properties, Sources, Targets, and Their Implication in Various Diseases. Indian J Clin Biochem 2015;30:11–26.
- [21]Bokare AD, Choi W. Review of iron-free Fenton-like systems for activating H2O2 in advanced oxidation processes. J Hazard Mater 2014;275:121–35.
- [22]Baker HM, Baker EN. A structural perspective on lactoferrin function. Biochem Cell Biol 2012;90:320–8.
- [23]Lopez A, Cacoub P, Macdougall IC, Peyrin-Biroulet L. Iron deficiency anaemia. Lancet 2016;387:907–16.
- [24]Gupta PM, Perrine CG, Mei Z, Scanlon KS. Iron, anemia, and Iron deficiency anemia among young children in the United States. Nutrients 2016;8:10–3.
- [25]Rezk M, Kandil M, Dawood R, Shaheen A, Allam A. Oral lactoferrin versus ferrous sulphate and ferrous fumerate for the treatment of iron deficiency anemia during pregnancy. J Adv Nutr Hum Metab 2015:1–5.
- [26]Paesano R, Berlutti F, Pietropaoli M, Pantanella F, Pacifici E, Goolsbee W, et al. Lactoferrin efficacy versus ferrous sulfate in curing iron deficiency and iron deficiency anemia in pregnant women. BioMetals 2010;23:411–7.
- [27]García-Montoya IA, Cendón TS, Arévalo-Gallegos S, Rascón-Cruz Q. Lactoferrin a multiple bioactive protein: An overview. Biochim Biophys Acta – Gen Subj 2012;1820:226–36.
- [28]Jenssen H, Hancock REW. Antimicrobial properties of lactoferrin. Biochimie 2009;91:19–29.
- [29]J O, FF B. Effect of food components on growth of Bacillus stearothermophilus. J Food Sci n.d.;39:1157–60.
- [30]Oliver SP, Duby RT, Prange RW, Tritschler JP. Residues in Colostrum Following Antibiotic Dry Cow Therapy. J Dairy Sci 1984;67:3081–4.
- [31]Oram JD, Reiter B. Inhibition of bacteria by lactoferrin and other iron-chelating agents. BBA – Gen Subj 1968;170:351–65.
- [32]Flores-Villaseñor H, Canizalez-Román A, Reyes-Lopez M, Nazmi K, De La Garza M, Zazueta-Beltrán J, et al. Bactericidal effect of bovine lactoferrin, LFcin, LFampin and LFchimera on antibiotic-resistant Staphylococcus aureus and Escherichia coli. BioMetals 2010;23:569–78.
- [33]Beeckman DSA, Van Droogenbroeck CMAD, De Cock BJA, Van Oostveldt P, Vanrompay DCG. Effect of ovotransferrin and lactoferrins on Chlamydophila psittaci adhesion and invasion in HD11 chicken macrophages. Vet Res 2007;38:729–39.
- [34]Qiu J, Hendrixson DR, Baker EN, Murphy TF, St. Geme JW, Plaut AG. Human milk lactoferrin inactivates two putative colonization factors expressed by Haemophilus influenzae. Proc Natl Acad Sci U S A 1998;95:12641–6.
- [35]Hendrixson DR, Qiu J, Shewry SC, Fink DL, Petty S, Baker EN, et al. Human milk lactoferrin is a serine protease that cleaves Haemophilus surface proteins at arginine-rich sites. Mol Microbiol 2003;47:607–17.
- [36]Schaible UE, Collins HL, Priem F, Kaufmann SHE. Correction of the iron overload defect in β-2-microglobulin knockout mice by lactoferrin abolishes their increased susceptibility to tuberculosis. J Exp Med 2002;196:1507–13.
- [37]Hwang SA, Kruzel ML, Actor JK. Lactoferrin augments BCG vaccine efficacy to generate T helper response and subsequent protection against challenge with virulent Mycobacterium tuberculosis. Int Immunopharmacol 2005;5:591–9.
- [38]Wu J, Hu Y, Du C, Piao J, Yang L, Yang X. The effect of recombinant human lactoferrin from the milk of transgenic cows on Salmonella enterica serovar typhimurium infection in mice. Food Funct 2016;7:308–14.
- [39]Legrand D, Pierce A, Elass E, Carpentier M, Mariller C, Mazurier J. Lactoferrin structure and functions. Adv Exp Med Biol 2008;606:163–94.
- [40]Reyes-cortes R, Acosta-smith E, Mondragón-flores R. Antibacterial and cell penetrating-effects of LFcin17-30 , LFampin265-284 and LFchimera on enteroaggregative Escherichia coli. Cell Biol 2017;95:76–81.
- [41]Van Der Does AM, Joosten SA, Vroomans E, Bogaards SJP, Van Meijgaarden KE, Ottenhoff THM, et al. The antimicrobial peptide hLF1-11 drives monocyte-dendritic cell differentiation toward dendritic cells that promote antifungal responses and enhance Th17 polarization. J Innate Immun 2012;4:284–92.
- [42]Silva T, Adão R, Nazmi K, Bolscher JGM, Funari SS, Uhríková D, et al. Structural diversity and mode of action on lipid membranes of three lactoferrin candidacidal peptides. Biochim Biophys Acta – Biomembr 2013;1828:1329–39.
- [43]Al-Sheikh H. Effect of lactoferrin and iron on the growth of human pathogenic Candida species. Pakistan J Biol Sci 2009;12:91–4.
- [44]Zarember KA, Sugui JA, Chang YC, Kwon-Chung KJ, Gallin JI. Human Polymorphonuclear Leukocytes Inhibit Aspergillus fumigatus Conidial Growth by Lactoferrin-Mediated Iron Depletion . J Immunol 2007;178:6367–73.
- [45]Takakura N, Wakabayashi H, Ishibashi H, Teraguchi S, Tamura Y, Yamaguchi H, et al. Oral lactoferrin treatment of experimental oral candidiasis in mice. Antimicrob Agents Chemother 2003;47:2619–23.
- [46]Van der Strate BWA, Beljaars L, Molema G, Harmsen MC, Meijer DKF. Antiviral activities of lactoferrin. Antiviral Res 2001;52:225–39.
- [47]Redwan EM, Uversky VN, El-Fakharany EM, Al-Mehdar H. Potential lactoferrin activity against pathogenic viruses. Comptes Rendus – Biol 2014;337:581–95.
- [48]Berlutti F, Pantanella F, Natalizi T, Frioni A, Paesano R, Polimeni A, et al. Antiviral properties of lactoferrin\-a natural immunity molecule. Molecules 2011;16:6992–7012.
- [49]SF M. Prophylactic effect of bovine lactoferrin against acute toxoplasmosis in immunocompetent and immunosuppressed mice. J Egypt Soc Parasitol 2009;39:1033–47.
- [50]Rodrigues L, Teixeira J, Schmitt F, Paulsson M, Månsson HL. Lactoferrin and cancer disease prevention. Crit Rev Food Sci Nutr 2009;49:203–17.
- [51]JK A, SA H, M K. Lactoferrin as a natural immune modulator. Curr Pharm Des 2009;15:1956–73.
- [52]Ward PP, Paz E, Conneely OM. Multifunctional roles of lactoferrin: A critical overview. Cell Mol Life Sci 2005;62:2540–8.
- [53]Medzhitov R. Origin and physiological roles of inflammation. Nature 2008;454:428–35.
- [54]Majno G, Joris I. Cells, tissues, and disease: principles of general pathology. Oxford University Press; 2004.
- [55]Nathan C. Points of control in inflammation. Nature 2002;420:846–52.
- [56]Uddin MJ, Dorotea D, Pak ES, Ha H. Fyn kinase: A potential therapeutic target in acute kidney injury. Biomol Ther 2020;28:213–21.
- [57]Moni A, Iqbal A, Uddin MJ. Resveratrol attenuates inflammation through tristetraprolin expression in human hepatocytes. J Adv Biotechnol Exp Ther 2018;1:78–82.
- [58]Legrand D, Elass E, Pierce A, Mazurier J. Lactoferrin and host defence: An overview of its immuno-modulating and anti-inflammatory properties. BioMetals 2004;17:225–9.
- [59]Wong SH, Francis N, Chahal H, Raza K, Salmon M, Scheel-toellner D, et al. Lactoferrin is a survival factor for neutrophils in rheumatoid synovial fluid. Rheumatology 2009;48:39–44.
- [60]Brock JH. The physiology of lactoferrin. Biochem. Cell Biol., vol. 80, Biochem Cell Biol; 2002, p. 1–6.
- [61]Fillebeen C, Ruchoux MM, Mitchell V, Vincent S, Benassa M, Pierce A. Lactoferrin is synthesized by activated microglia in the human substantia nigra and its synthesis by the human microglial CHME cell line is upregulated by tumor necrosis factor α or 1-methyl-4-phenylpyridinium treatment. Mol Brain Res 2001;96:103–13.
- [62]Fillebeen C, Dehouck B, Benaïssa M, Dhennin-Duthille I, Cecchelli R, Pierce A. Tumor necrosis factor-α increases lactoferrin transcytosis through the blood-brain barrier. J Neurochem 1999;73:2491–500.
- [63]Elrod KC, Moore WR, Abraham WM, Tanaka RD. Lactoferrin, a potent tryptase inhibitor, abolishes late-phase airway responses in allergic sheep. Am J Respir Crit Care Med 1997;156:375–81.
- [64]Griffiths CEM, Cumberbatch M, Tucker SC, Dearman RJ, Andrew S, Headon DR, et al. Exogenous topical lactoferrin inhibits allergen-induced Langerhans cell migration and cutaneous inflammation in humans. Br J Dermatol 2001;144:715–25.
- [65]Zweiman B, Kucich U, Shalit M, Von Allmen C, Moskovitz A, Weinbaum G, et al. Release of lactoferrin and elastase in human allergic skin reactions. J Immunol 1990;144:3953–60.
- [66]Kimber I, Cumberbatch M, Dearman RJ, Headon DR, Bhushan M, Griffiths CEM. Lactoferrin: Influences on Langerhans cells, epidermal cytokines, and cutaneous inflammation. Biochem. Cell Biol., vol. 80, Biochem Cell Biol; 2002, p. 103–7.
- [67]Kruzel ML, Zimecki M, Actor JK. Lactoferrin in a context of inflammation-induced pathology. Front Immunol 2017;8.
- [68]Jones DP. Redefining oxidative stress. Antioxidants Redox Signal 2006;8:1865–79.
- [69]Finaud J, Lac G, Filaire E. Oxidative stress: Relationship with exercise and training. Sport Med 2006;36:327–58.
- [70]Storz G, Imlay JA. Oxidative stress. Curr Opin Microbiol 1999;2:188–94.
- [71]Superti F. Lactoferrin from bovine milk: A protective companion for life. Nutrients 2020;12:1–26.
- [72]Shinmoto H, Dosako SI, Nakajima I. Anti-oxidant Activity of Bovine Lactoferrin on Iron/Ascorbate Induced Lipid Peroxidation. Biosci Biotechnol Biochem 1992;56:2079–80.
- [73]Mulder AM, Connellan PA, Oliver CJ, Morris CA, Stevenson LM. Bovine lactoferrin supplementation supports immune and antioxidant status in healthy human males. Nutr Res 2008;28:583–9.
- [74]Okazaki Y, Kono I, Kuriki T, Funahashi S, Fushimi S, Iqbal M, et al. Bovine lactoferrin ameliorates ferric nitrilotriacetate-induced renal oxidative damage in rats. J Clin Biochem Nutr 2012;51:84–90.
- [75]Wang YZ, Xu CL, An ZH, Liu JX, Feng J. Effect of dietary bovine lactoferrin on performance and antioxidant status of piglets. Anim Feed Sci Technol 2008;140:326–36.
- [76]Shoji H, Oguchi S, Shinohara K, Shimizu T, Yamashiro Y. Effects of iron-unsaturated human lactoferrin on hydrogen peroxide-induced oxidative damage in intestinal epithelial cells. Pediatr Res 2007;61:89–92.
- [77]Habib HM, Ibrahim WH, Schneider-Stock R, Hassan HM. Camel milk lactoferrin reduces the proliferation of colorectal cancer cells and exerts antioxidant and DNA damage inhibitory activities. Food Chem 2013;141:148–52.
- [78]Lee K-C, Hsieh K-T, Chen R-B, Lin W-C, Wang C-S, Lee T-T, et al. Expression and Characterization of Rice-produced Recombinant Porcine Lactoferrin and its Antioxidant Activities. Open Biotechnol J 2020;14:94–106.
- [79]Pastori V, Tavazzi S, Lecchi M. Lactoferrin-loaded contact lenses: Eye protection against oxidative stress. Cornea 2015;34:693–7.
- [80]Zimecki M, Mazurier J, Spik G, Kapp JA. Human lactoferrin induces phenotypic and functional changes in murine splenic B cells. Immunology 1995;86:122–7.
- [81]Zimecki M, Kociȩba M, Kruzel M. Immunoregulatory activities of lactoferrin in the delayed type hypersensitivity in mice are mediated by a receptor with affinity to mannose. Immunobiology 2002;205:120–31.
- [82]Legrand D. Overview of Lactoferrin as a Natural Immune Modulator. J Pediatr 2016;173:S10–5.
- [83]Kruzel ML, Actor JK, Zimecki M, Wise J, Płoszaj P, Mirza S, et al. Novel recombinant human lactoferrin: Differential activation of oxidative stress related gene expression. J Biotechnol 2013;168:666–75.
- [84]Park SY, Jeong A-J, Kim G-Y, Jo A, Lee JE, Leem S-H, et al. Lactoferrin Protects Human Mesenchymal Stem Cells from Oxidative Stress-Induced Senescence and Apoptosis. J Microbiol Biotechnol 2017;27:1877–84.
- [85]Agarwal A, Aponte-Mellado A, Premkumar BJ, Shaman A, Gupta S. The effects of oxidative stress on female reproduction: A review. Reprod Biol Endocrinol 2012;10:1–31.
- [86]Agarwal A, Gupta S, Sharma RK. Role of oxidative stress in female reproduction. Reprod Biol Endocrinol 2005;3:1–21.
- [87]Duhig K, Chappell LC, Shennan AH. Oxidative stress in pregnancy and reproduction. Obstet Med 2016;9:113–6.
- [88]Vesce F, Giugliano E, Bignardi S, Cagnazzo E, Colamussi C, Marci R, et al. Vaginal lactoferrin administration before genetic amniocentesis decreases amniotic interleukin-6 levels. Gynecol Obstet Invest 2014;77:245–9.
- [89]Trentini A, Maritati M, Cervellati C, Manfrinato MC, Gonelli A, Volta CA, et al. Vaginal lactoferrin modulates PGE2, MMP-9, MMP-2, and TIMP-1 amniotic fluid concentrations. Mediators Inflamm 2016.
- [90]Maritati M, Comar M, Zanotta N, Seraceni S, Trentini A, Corazza F, et al. Influence of vaginal lactoferrin administration on amniotic fluid cytokines and its role against inflammatory complications of pregnancy. J Inflamm (United Kingdom) 2017;14:1–8.
- [91]Trentini A, Maritati M, Rosta V, Cervellati C, Manfrinato MC, Hanau S, et al. Vaginal Lactoferrin Administration Decreases Oxidative Stress in the Amniotic Fluid of Pregnant Women: An Open-Label Randomized Pilot Study. Front Med 2020;7:555.
- [92]Safaeian L, Javanmard SH, Mollanoori Y, Dana N. Cytoprotective and antioxidant effects of human lactoferrin against H2O2-induced oxidative stress in human umbilical vein endothelial cells. Adv Biomed Res 2015;4:188–188.
- [93]Wynn TA. Cellular and molecular mechanisms of fibrosis. J Pathol 2008;214:199–210.
- [94]Hsu YH, Chiu IJ, Lin YF, Chen YJ, Lee YH, Chiu HW. Lactoferrin contributes a renoprotective effect in acute kidney injury and early renal fibrosis. Pharmaceutics 2020;12.
- [95]Hessin A, Hegazy R, Hassan A, Yassin N, Kenawy S. Lactoferrin enhanced apoptosis and protected against thioacetamide-induced liver fibrosis in rats. Maced J Med Sci 2015;3:195–201.
- [96]Cutone A, Lepanto MS, Rosa L, Scotti MJ, Rossi A, Ranucci S, et al. Aerosolized bovine lactoferrin counteracts infection, inflammation and iron dysbalance in a cystic fibrosis mouse model of pseudomonas aeruginosa chronic lung infection. Int J Mol Sci 2019;20:2128.
- [97]Salsgiver EL, Fink AK, Knapp EA, LiPuma JJ, Olivier KN, Marshall BC, et al. Changing epidemiology of the respiratory bacteriology of patients with cystic fibrosis. Chest 2016;149:390–400.
- [98]LiPuma JJ. The changing microbial epidemiology in cystic fibrosis. Clin Microbiol Rev 2010;23:299–323.
- [99]Emerson J, Rosenfeld M, McNamara S, Ramsey B, Gibson RL. Pseudomonas aeruginosa and other predictors of mortality and morbidity in young children with cystic fibrosis. Pediatr Pulmonol 2002;34:91–100.
- [100]Reid DW, Withers NJ, Francis L, Wilson JW, Kotsimbos TC. Iron deficiency in cystic fibrosis: Relationship to lung disease severity and chronic Pseudomonas aeruginosa infection. Chest 2002;121:48–54.
- [101]Reid DW, Lam QT, Schneider H, Walters EH. Airway iron and iron-regulatory cytokines in cystic fibrosis. Eur Respir J 2004;24:286–91.
- [102]Reid DEW, Carroll V, O’May C, Champion A, Kirov SM. Increased airway iron as a potential factor in the persistence of Pseudomonas aeruginosa infection in cystic fibrosis. Eur Respir J 2007;30:286–92.
- [103]Ghio AJ, Roggli VL, Soukup JM, Richards JH, Randell SH, Muhlebach MS. Iron accumulates in the lavage and explanted lungs of cystic fibrosis patients. J Cyst Fibros 2013;12:390–8.
- [104]Ward DM, Kaplan J. Ferroportin-mediated iron transport: Expression and regulation. Biochim Biophys Acta – Mol Cell Res 2012;1823:1426–33.
- [105]Hogardt M, Heesemann J. Microevolution of pseudomonas aeruginosa to a chronic pathogen of the cystic fibrosis lung. Curr Top Microbiol Immunol 2013;358:91–118.
- [106]Singh PK, Parsek MR, Greenberg EP, Welsh MJ. A component of innate immunity prevents bacterial biofilm development. Nature 2002;417:552–5.
- [107]Valenti P, Antonini G. Lactoferrin: An important host defence against microbial and viral attack. Cell Mol Life Sci 2005;62:2576–87.
- [108]Frioni A, Conte MP, Cutone A, Longhi C, Musci G, Di Patti MCB, et al. Lactoferrin differently modulates the inflammatory response in epithelial models mimicking human inflammatory and infectious diseases. BioMetals 2014;27:843–56.
- [109]Berlutti F, Schippa S, Morea C, Sarli S, Perfetto B, Donnarumma G, et al. Lactoferrin downregulates pro-inflammatory cytokines upexpressed in intestinal epithelial cells infected with invasive or noninvasive Escherichia coli strains. Biochem. Cell Biol., vol. 84, Biochem Cell Biol; 2006, p. 351–7.
- [110]Sessa R, Di Pietro M, Filardo S, Bressan A, Rosa L, Cutone A, et al. Effect of bovine lactoferrin on Chlamydia trachomatis infection and inflammation. Biochem Cell Biol 2017;95:34–40.
- [111]Legrand D. Lactoferrin, a key molecule in immune and inflammatory processes. Biochem Cell Biol 2012;90:252–68.
- [112]Valenti P, Rosa L, Capobianco D, Lepanto MS, Schiavi E, Cutone A, et al. Role of lactobacilli and lactoferrin in the mucosal cervicovaginal defense. Front Immunol 2018;9:1.
- [113]Malhotra JD, Kaufman RJ. Endoplasmic reticulum stress and oxidative stress: A vicious cycle or a double-edged sword? Antioxidants Redox Signal 2007;9:2277–93.
- [114]Uddin MJ, Pak ES, Ha H. Carbon monoxide releasing molecule-2 protects mice against acute kidney injury through inhibition of ER stress. Korean J Physiol Pharmacol 2018;22:567–75.
- [115]Sano R, Reed JC. ER stress-induced cell death mechanisms. Biochim Biophys Acta – Mol Cell Res 2013;1833:3460–70.
- [116]Xu C, Bailly-Maitre B, Reed JC. Endoplasmic reticulum stress: Cell life and death decisions. J Clin Invest 2005;115:2656–64.
- [117]Guo C, Xue H, Guo T, Zhang W, Xuan WQ, Ren YT, et al. Recombinant human lactoferrin attenuates the progression of hepatosteatosis and hepatocellular death by regulating iron and lipid homeostasis in: Ob / ob mice. Food Funct 2020;11:7183–96.
- [118]Bhattacharya A, Wei Q, Shin JN, Abdel Fattah E, Bonilla DL, Xiang Q, et al. Autophagy Is Required for Neutrophil-Mediated Inflammation. Cell Rep 2015;12:1731–9.
- [119]Li L, Zhang X, Le W. Autophagy Dysfunction in Alzheimer’s Disease. Neurodegener Dis 2010;7:265–71.
- [120]He L, Zhang J, Zhao J, Ma N, Kim SW, Qiao S, et al. Autophagy: The last defense against cellular nutritional stress. Adv Nutr 2018;9:493–504.
- [121]Sohn M, Kim K, Uddin MJ, Lee G, Hwang I, Kang H, et al. Delayed treatment with fenofibrate protects against high-fat diet-induced kidney injury in mice: the possible role of ampk autophagy. Am J Physiol – Ren Physiol 2017;312:F323–34.
- [122]Stern ST, Adiseshaiah PP, Crist RM. Autophagy and lysosomal dysfunction as emerging mechanisms of nanomaterial toxicity. Part Fibre Toxicol 2012;9:1–17.
- [123]Park YG, Jeong JK, Lee JH, Lee YJ, Seol JW, Kim SJ, et al. Lactoferrin protects against prion protein-induced cell death in neuronal cells by preventing mitochondrial dysfunction. Int J Mol Med 2013;31:325–30.
- [124]Kusminski CM, Scherer PE. Mitochondrial dysfunction in white adipose tissue. Trends Endocrinol Metab 2012;23:435–43.
- [125]Brand MD, Nicholls DG. Assessing mitochondrial dysfunction in cells. Biochem J 2011;435:297–312.
- [126]Wang J, Yu Y, Hashimoto F, Sakata Y, Fujii M, Hou DX. Baicalein induces apoptosis through ROS-mediated mitochondrial dysfunction pathway in HL-60 cells. Int J Mol Med 2004;14:627–32.
- [127]Pak ES, Uddin MJ, Ha H. Inhibition of src family kinases ameliorates lps-induced acute kidney injury and mitochondrial dysfunction in mice. Int J Mol Sci 2020;21:1–17.
- [128]Park KW, Jin BK. Thrombin-induced oxidative stress contributes to the death of hippocampal neurons: Role of neuronal NADPH oxidase. J Neurosci Res 2008.
- [129]Kruzel ML, Actor JK, Radak Z, Bacsi A, Saavedra-Molina A, Boldogh I. Lactoferrin decreases LPS-induced mitochondrial dysfunction in cultured cells and in animal endotoxemia model. Innate Immun 2010;16:67–79.
- [130]ClinicalTrials.gov Identifier: NCT03481790. Lactoferrin Versus Ferrous Sulphate for Treatment of Iron Deficiency Anaemia During Pregnancy 2018.
- [131]ClinicalTrials.gov Identifier: NCT03367013. Lactoferrin Infant Feeding Trial – LIFT_Canada 2020.
- [132]ClinicalTrials.gov Identifier: NCT03163212. Safety and Tolerability of Lactoferrin/FOS in Very Low Birth Weight Infants 2017.
- [133]Mayeur S, Spahis S, Pouliot Y, Levy E. Lactoferrin, a Pleiotropic Protein in Health and Disease. Antioxidants Redox Signal 2016;24:813–36.
- [134]Kondapi AK. Targeting cancer with lactoferrin nanoparticles: recent advances. Nanomedicine 2020;15:2071–83.