A hybrid pretreatment strategy for delignification of Aloe vera processing waste and its effectiveness towards saccharification
Abstract
A copious amount of rigid Aloe vera leaf rind (AVLR) has been produced from the aloe gel processing industries are majorly disposed as wastes since it has no commercial value. The cell wall compositional analysis revealed that significant quantity of cellulose (46% ± 0.76, w/w) and hemicellulose (18.5% ± 0.24, w/w) which justifies as potent source for bioethanol production. However, high lignin content (13.95% ± 0.45, w/w) hinders depolymerization of polysaccharides into fermentable sugars and subsequent fermentation for ethanol production. In the present study, microwave-assisted alkali (MAA) pretreatment of AVLR was performed by varying the power level (160 W, 320 W and 480 W) which showed a maximum delignification (66.38%) at 320 W. Scanning Electron Microscope (SEM), Fourier-transform infrared spectroscopy (FTIR) and X-ray powder diffraction (XRD) based characterization were performed to study the extent of delignification in AVLR biomass. The Gas Chromatography-Mass Spectrometry (GC-MS) analysis was performed for the liquid hydrolysate obtained after MAA pretreatment at 320 W indicated that the hydrolysate contained more of oxidized phenolic hydrocarbons that can be potentially utilized for other value-added product synthesis. A comparison of saccharification efficiency was performed using two different cellulase producers namely Aspergillus niger and Aspergillus sp. A 2.8-fold increase in sugar yield was achieved by Aspergillus sp., with a maximum saccharification of 68.5% ± 0.34 on comparing with untreated AVLR biomass. This indicates the feasibility of MAA pretreatment for AVLR biomass in order to improve the accessibility of fermentable sugars available for ethanol production.
Keywords
INTRODUCTION
In recent years due to change in lifestyle patterns and increased awareness among consumers regarding their health lead to a paradigm shift in consumer preference towards natural alternatives and herbal based nutraceuticals. Aloe vera finds a notable position among the natural beverages, personal and healthcare medication due to its extensive superior pharmacological and nutritional properties [1]. For the bygone year, the market value for Aloe vera based drinks is found to be around $77.8 million and forecast to grow with 11.3% of compound annual growth rate by 2027 [2]. Many industrial units processing Aloe vera for gel/sap extraction has been already established on the basis of market demand, and it has been estimated that there are more than 300 Aloe vera processing industries in the country [3]. The peripheral rind of the Aloe vera leaf has been stripped off and the inner leaf material (i.e., aloe gel) were processed for Aloe vera juice preceded by washing/ rinsing away the latex. Aloe vera leaf rind (AVLR) which is lignocellulosic biomass impedes its usage as a cattle feed due to the bitterness (aloin – quinone derivative) and thorns on the lateral surfaces. Currently, it is applied either as a biomanure or disposed as waste. However, analysis on AVLR indicated that it constitutes α-cellulose of about 57.72% ± 2.18 (w/w) that was used for nanofibre synthesis [4]. Hellen Sathya et al. has reported that AVLR can be utilized as raw material for bioethanol by performing acid-based pretreatment and hydrolysis of cellulose to obtain reducing sugars for fermentation [5]. However, acid-based pretreatment of lignocellulosic biomass has certain operational and furfural problems [6, 7]. Therefore, recently to have a cost-effective and efficient pretreatment process, certain hybrid strategies such as thermo-chemical processes are being employed. Microwave pretreatment is one such process where several advantages that include high heating efficiency, ease of operation with instantaneous start and stop facility and uniform selective processing of biomass [7, 8]. There were successful demonstrations of employing microwave treatment to degrade lignin, modify cellulose for its increased accessibility for enzymatic treatment for hydrolysis [9]. Hence, microwave treatment could be assisted with mild chemical agents (acid/alkali) to increase the rate of pretreatment at the same time reducing the impact of inhibitors. In this present study, an attempt has been made to investigate the efficiency of the process by employing Microwave-Assisted Alkali (MAA) pretreatment of AVLR followed by enzymatic hydrolysis of cellulose using crude cellulase produced by two different strains viz., Aspergillus niger and Aspergillus sp. To the best of authors’ knowledge, this is the first study to inculcate such process strategy (MAA + cellulase hydrolysis) for AVLR to be used as raw material for second-generation bioethanol production. Through this study, the effect of different microwave radiation power and its consequence on the efficiency of cellulase-mediated hydrolysis was reported. Morphological (Scanning Electron Microscope (SEM)) and structural studies (Fourier-transform infrared spectroscopy (FTIR) and X-ray diffraction (XRD)) were done to analyze the delignification, and the intermediary degradation product released during MAA pretreatment was analysis by Gas Chromatography Mass Spectrometry (GC-MS).
MATERIALS AND METHODS
Substrate
AVLR was procrued from the aloe gel processing unit located in Tiruchirappalli, Tamil Nadu, India (10.7905° N, 78.7047° E). The feedstock was sun-dried and pulverized to 0.18 mm particle size, stored in an air-tight container under the moisture-free condition at room temperature for further use.
Microorganism and inoculum preparation
Cellulolytic fungus, Aspergillus niger was procured from culture collection center, National Chemical Laboratory (NCL), Pune. Another fungus that has been isolated from old decaying wood in a nearby locality is used for cellulase production and was identified through preliminary screening in the laboratory as Aspergillus sp. Further, the characterization of the organism to identify the species is in progress. These cultures were grown on Potato dextrose agar (PDA) and sub-cultured regularly. The purpose of using the standard cellulase producer is to compare the hydrolytic efficiency of the newly isolated strain.
Structural composition of AVLR feedstock
Proximate and biochemical analysis
Analysis of moisture content, volatile solids, and ash content for AVLR were carried out using standard protocol [10].
Estimation of lignin
Estimation of AVLR lignin content by iodometric method was done as reported by Hussain et al. where equal volume (7.5 mL) of 4 N sulphuric acid and standard 0.1 N potassium permanganate were mixed and further added to 0.05 g of AVLR biomass for oxidizing the AVLR lignin [11]. After incubation at 25 °C for about 10 minutes the reaction was subsequently stopped by the addition of 1.5 mL potassium iodide (1N), followed by titrating sodium thiosulphate (0.1 N) with the free iodine using a starch indicator. AVLR lignin content was calculated using Eq. 1.
Estimation of hemicellulose and cellulose
As reported by Marlett and Lee, the hemicellulose content in the AVLR biomass was estimated by treating the dried AVLR biomass with 1%, v/v of 1 N sulphuric acid and incubated at 100 °C. After 4 hours of incubation, the AVLR biomass was dried overnight and total soluble sugars difference between treated and untreated (control) AVLR biomass was used for determining the hemicellulose composition [12]. The cellulose content of AVLR biomass was determined as reported by Sun et al. where 5 g of AVLR biomass was treated with an acid mixture of 100 mL of acetic acid (80%, (v/v)) and 10 mL of nitric acid (70%, (v/v)) incubated at 100 °C for about 20 minutes. Until pH remains neutral, repeated washing was done using distilled water and ethanol (95%, (v/v)) in order to remove acid and the reaction breakdown products. Then, the residual biomass was subjected to overnight drying at 60 °C in a hot air oven [13]. The dry weight fraction of final treated and initial sample gives the cellulose percentage in AVLR biomass as provided in Eq. 3. The Reducing sugar were determined by Dinitrosalicylic acid method [14].
Production of cellulase by solid state fermentation
Sugarcane bagasse has been used as a substrate for solid state fermentation (SSF) was collected from the local markets of Chennai, Tamil Nadu, India (13.0827° N, 80.2707° E). Before use in SSF, the substrate was dried and chopped into different size. The biomass was sieved to determine the different particle size of the substrate. For placing the SSF set 5 g of sugarcane bagasse having particle size of ~0.5 mm was taken in a polybag and mixed with the mineral media at a ratio of 1:3 (solid to liquid). The composition of mineral media is given as follows (g/L): Potassium dihydrogen phosphate (KH2PO4) – 2; Ferrous sulphate (FeSO4)- 0.005; Tryptone – 0.75; Calcium chloride (CaCl2) – 0.3; Manganese sulphate (MnSO4·H2O) – 0.0016; Ammonium sulphate ((NH4)2SO4) – 1.4; Magnesium sulphate (MgSO4)- 0.3; Zinc sulphate (ZnSO4)- 0.0014; Tween 80 – 2mL. The SSF sets were sterilized and inoculated with 5 mL (~2.4 x 105 cells/ mL) spore suspension of Aspergillus niger and Aspergillus sp., in respective bags for cellulase production. The SSF sets were incubated for 5 days at 32° C with relative humidity of 80%.
Cellulase extraction and assay
The fermented biomass was soaked in 15 mL of glycerol solution (5% (v/v)) followed by incubation for 2 h at room temperature. The extracellular enzyme was extracted by squeezing, filtering through cheesecloth and subjected to centrifugation (5000 rpm for about 10 minutes at 4 °C) to remove the suspending particles. The supernatant was analyzed for the total cellulase (endoglucanase and exoglucanase) and endoglucanase activities using FPA (filter paper assay) and CMC (Carboxy Methyl Cellulose) assay respectively [15]. An enzyme activity (IU/mL) was calculated as the one micromole of glucose released from the substrate during the hydrolysis reaction per milliliter of enzyme per min by using the formula as given in Eq. 4.
Microwave-assisted alkali delignification of AVLR
Laboratory microwave oven (LG, model MC2149BPB, India) was used to carry out pretreatment which provided the microwave radiation at a different power level. Sodium hydroxide solution of about 10 mL ((NaOH- 2%, w/v) was added to 1 g of dried AVLR biomass and exposed to different power levels of microwave radiation (160W, 320W and 480W) for 10 minutes. The reaction time and the power were set based on the previous studies as reported by author itself [16]. The residual AVLR biomass obtained after MAA pretreatment was neutralized by washing with 200 mL of distilled water followed by centrifugation at 5000 rpm for about 10-15 minutes to separate the solid biomass and liquid hydrolysate. Then, the solid biomass was sun dried until it attains the constant biomass weight and further storing it at room temperature for later studies. Further, the liquid hydrolysate obtained after MAA pretreatment was subjected to reducing sugar estimation and the volatile fraction of depolymerized lignin was analyzed by GC-MS [17, 18].
Cellulase hydrolysis of delignified AVLR
Enzymatic hydrolysis by crude cellulase was carried out as reported by Mukhopadhyay et al. with the reaction parameters like solid to liquid ratio 1:18 (w/v) at 50 °C for 6 h [19]. In our study, MAA pretreated AVLR biomass was mixed with crude enzyme at the ratio of 1:18 (w/v) with the obtained optimal maximum activity ((6.57 IU/mL CMC-ase and (0.89- Filter paper units/mL for – Aspergillus sp.), (3.19 IU/mL CMC-ase and 0.42 Filter paper units/mL for Aspergillus niger)) at the end of SSF. After incubation, the supernatant was analyzed for reducing sugar and Eq. 5 is employed for calculating the percentage of saccharification [20].
Structural characterization of treated and untreated AVLR
SEM characterization
SEM images were used to analyze the morphology of AVLR before and after MAA pretreatment. Dried samples were spray coated with gold particle and observed under SEM (FEI QUANTA 200, USA) [21].
FTIR characterization
To analyze the various functional groups of AVLR structural components, FTIR (Agilent Technologies, Cary 600 Series, USA) was employed where KBr pellet technique was performed using FTIR spectrometer. A spectrum range of 400 cm−1 to 4000 cm−1 and 0.5 cm−1 of spectral resolution was analyzed [22].
XRD characterization
XRD diffraction pattern for untreated and MAA pretreated AVLR was obtained using X’pert XRD system (Malvern Panalytical, AERIS- High resolution bench-top XRD, UK). The changes in crystallinity of cellulose were determined using the CuKα radiation at a wavelength of 1.54 Å and scanned with a speed of 3°min-1 from 10 to 75° (2θ) at 40 kV. Crystallinity index of the cellulose before and after pretreatment was calculated by using the following formula (Eq. 6) provided by the Segal method for native cellulose [23].
GC-MS analysis of AVLR lignin degradatory compounds
GC-MS analysis of volatile fractions of lignin degraded by-products after different polar and non-polar solvents recovery was performed (Agilent Technologies, 7890B GC system combined with 5977A MSD system, USA). A column (30 m×250 μm×0.25 μm) made of HP 5MS 5% phenyl-methyl-siloxane was used for separating the AVLR lignin degradatory compounds of the liquid hydrolysate obtained after MAA pretreatment. The carrier gas (helium) in a split ratio of 1:10 with 1.2mL/min as flow rate. The analysis was done at 45 °C for 1 min, followed by ramp (45 to 300 °C) at an interval of 10 °C/min withhold time of 1 min at 300 °C. The resulting spectra from the GC-MS analysis were compared with the reference spectra from the NIST library [18].
Statistical analysis
The values obtained in this study are average of triplicate experiments and the standard deviation is represented as (±) calculated from mean and three independent trials. The significance between the treated and untreated AVLR group has been analysed by student t-test using GraphPad prism v.8.0. p<0.05 is considered statistically significant.
RESULTS
Analysis of AVLR structural composition
The biochemical constituents such as lignin, hemicellulose and cellulose of AVLR biomass was found to be 13.95% ± 0.450, w/w, 18.5% ± 0.24, w/w and 46% ± 0.76, w/w respectively. Table 1 represents the proximate parameters of AVLR where the total volatile solid constituted about 84.31% (w/w) that provide high scope for AVLR to be subjected for biological processing.
The values are average of triplicate experiments and the standard deviation is represented as (±) calculated from mean and three independent trials; a – wet weight basis; b – dry weight basis
Table 1. Proximate and structural component analysis of AVLR biomass.
Delignification efficiency of MAA pretreatment for AVLR biomass
In the present study, a microwave power of 160, 320 and 480 W were employed for the delignification process. After MAA pretreatment, the solid AVLR biomass and liquid hydrolysate were subjected to estimation of lignin and reducing sugar yield respectively to determine the non-targeted hydrolysis during pretreatment as given in Table 2. The MAA pretreatment showed a maximum delignification of about 66.38% (w/w) when 1 g of AVLR feedstock was mixed with 10 mL of 2%, (w/v) NaOH and exposed to the microwave irradiation at 320 W for 10 minutes. As the power is increased to 480 W, a pronounced effect in the degradation of cellulose was observed that is reflected from increased reducing sugar (0.98 mg/mL) in the liquid hydrolysate obtained from the MAA pretreatment.
Table 2. Percentage of delignification in MAA pre-treated biomass and reducing sugar yield from the liquid wash obtained after pre-treatment.
Surface morphology analysis of AVLR biomass
The surface characteristic of the untreated AVLR and MAA pretreatment AVLR biomass was analyzed by SEM. From Figure 1 (a-d), it is evident that an increase in the roughness and formation of cracks in the treated sample compared with untreated biomass, which might be due to the distortion of a highly organized cell wall surface.
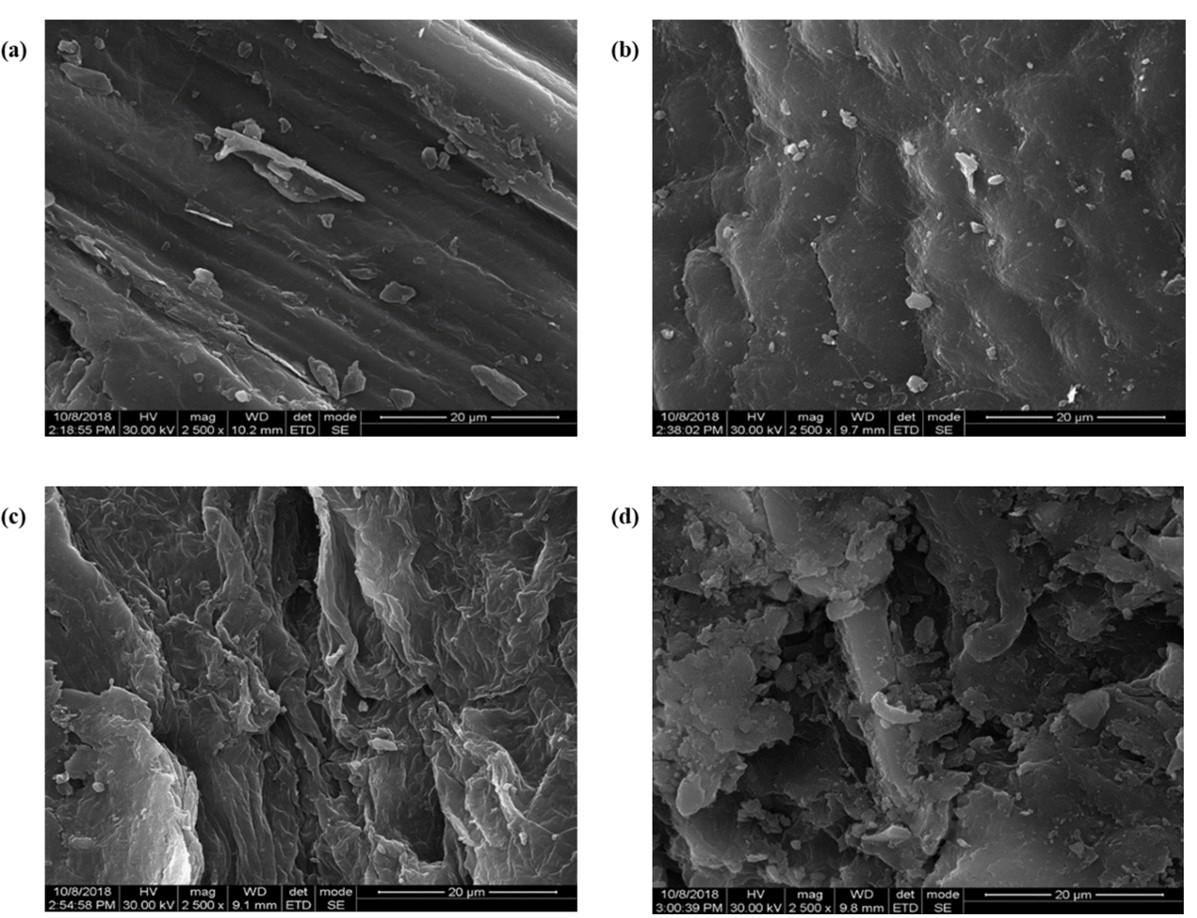
Functional group characterization of AVLR biomass
From the FTIR spectra of raw and delignified AVLR biomass Figure 2 (a-d), the core structure of lignin was inferred as syringyl group (S type) by the presence of its typical IR absorption spectra around the region of 1375 cm-1 [24]. The appearance of the peak between 1610 – 1635 cm-1 in AVLR biomass is due to the structural vibration of aromatic phenyl propane groups and the decrease in the intensity in MAA-treated sample as given in Figure 2 (b-d) suggest that the doublet phenolic lignin is reduced after pretreatment [25]. Further, the C-O and C-H stretching vibration of methyl, methylene of syringyl ring of lignin were attributed by the peak that appeared around 1316 cm-1 and 2938-2921 cm-1. One of the major important considerations for lignin removal is the syringyl (S)/ guaiacyl (G) ratio, higher the S/G ratio lower the affinity of enzyme to bind over the lignin layer [26]. The characteristic peak of guaiacyl ring is obtained around the region of 1270-1260 cm-1, 1516 cm-1 and 1161cm-1. Nearly absence of these G type lignin peaks is observed in the AVLR sample.
From the Figure 2 (b and d), the appearance of peak around 874 cm-1 region was attributed to the C-O-C stretching of monomeric units of cellulose at the β-(1, 4) glycosidic linkage [27] and the increase in peak intensity at 1103 cm-1 which could be observed only after pretreatment suggest that the MAA pretreatment of AVLR resulted in delignification of biomass and exposed the cellulose layer. One of the significant functional groups of polymeric lignin i.e. methyl- CH3 that links the poly aromatic structure whose transmittance at 1427 cm-1 is relatively reduced after the pretreatment suggest that the deformation of lignin to some extent and distortion of the crystalline structure of cellulose at 320 W which could add justification to the above discussion as the peak also contributes to the symmetric bending of CH2 in cellulose crystallinity [28]. The transmittance at 2930–2910 cm-1 were assigned to the stretching vibration of –CH, as well as 3450–3300 cm-1 for –OH groups present in lignin and the broadening of this band indicates the loosening of cellulose after the pretreatment [29]. Peaks between 1600 and 1635 cm-1 (aromatic skeletal vibrations) imply the splitting of aliphatic side chains of lignin during microwave irradiation.
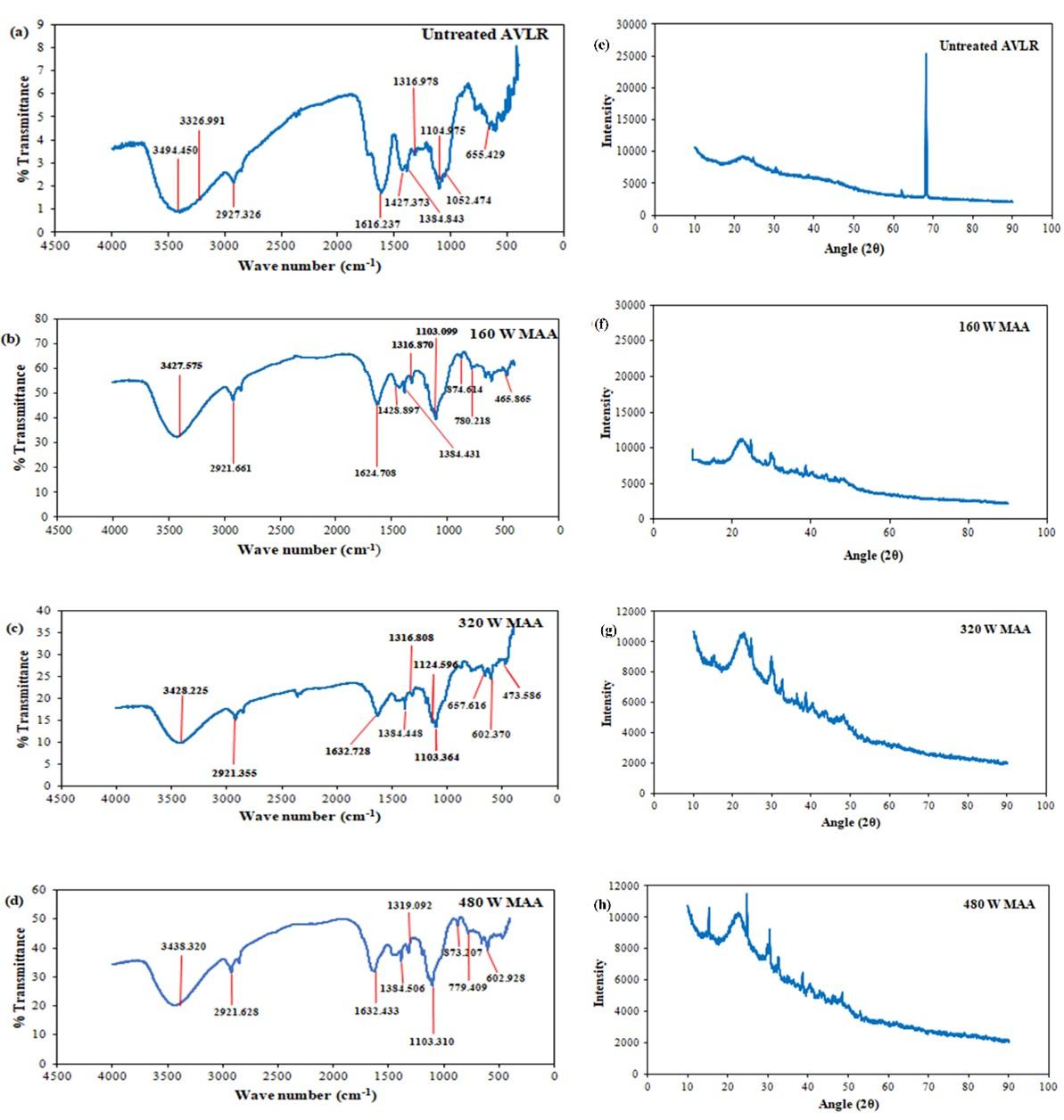
Crystallinity analysis of raw and MAA pretreated AVLR
The XRD spectra of untreated and treated biomass at different power levels have been illustrated in Figure 2 (e-h). As shown in Table 3, the crystallinity index (CrI) of the AVLR feedstock before and after MAA treatment was calculated from XRD spectra. The CrI value (21.57%) at 320 W was slightly less compared to 160 W (28.53%) which might be due to the disruption of some of the crystalline structure of cellulose which can be further justified by a peak disappearance (around 1427cm-1) which corresponds to the symmetric bending of CH2 in cellulose crystallinity. Therefore, it has been observed that pretreated biomass is highly amorphous which enables the enhanced cellulase accessibility for saccharification. Further, an increase in the microwave power (480 W) did not lead to a significant increase in CrI value (16.74) after MAA pretreatment when compared to other microwave power as shown in Table 3. As expected, the CrI value of the biomass was increased after the pretreatment than the untreated sample that supports the slight reduction in the transmittance of the peak in the vicinity of 1320 cm-1 corresponding to the distortion of lignin in the pretreated biomass as discussed earlier in the FTIR analysis Figure 2 (b-d).
Table 3. Crystallinity index percentage of AVLR biomass after MAA pretreatment.
Intermediary degradation products of MAA pre-treated AVLR
The GC-MS spectra that reflect the intermediary degradation product obtain after MAA treatment and solvent extraction have been shown in Figure 3 (a-d). The purpose of using solvent extraction with different polar solvents (acetonitrile, methanol) and non-polar solvents (hexane, chloroform) is to fractionate the lignin-depolymerized by-products/intermediates based on the chemical nature. From the chromatograph, it was clear that depolymerized lignin products that range from aromatic and aliphatic hydrocarbons along with some low molecular weight compounds such as alcohol, acid, ester, etc. The relative proportion of these components obtained from different solvents has been represented in a heatmap Figure 3 (e). The major compounds obtained using polar solvents were represented in Table 4 that includes aromatic hydrocarbons (pyridine, Benzene, 1,3-bis(1,1-dimethylethyl)-, Benzaldehyde, 4-propyl) and comparatively less phenolic compounds (phenol, 2,4-bis(1,1-dimethylethyl)-, phenol, 2,6-bis(1,1-dimethylethyl)-4-ethyl-) and small number of esters, acids and compounds with methoxy group were detected. It is notable that the relative area% of the aromatic hydrocarbon from liquid hydrolysate was relatively high in non-polar solvents (20.51%) than polar solvents (14.21%).
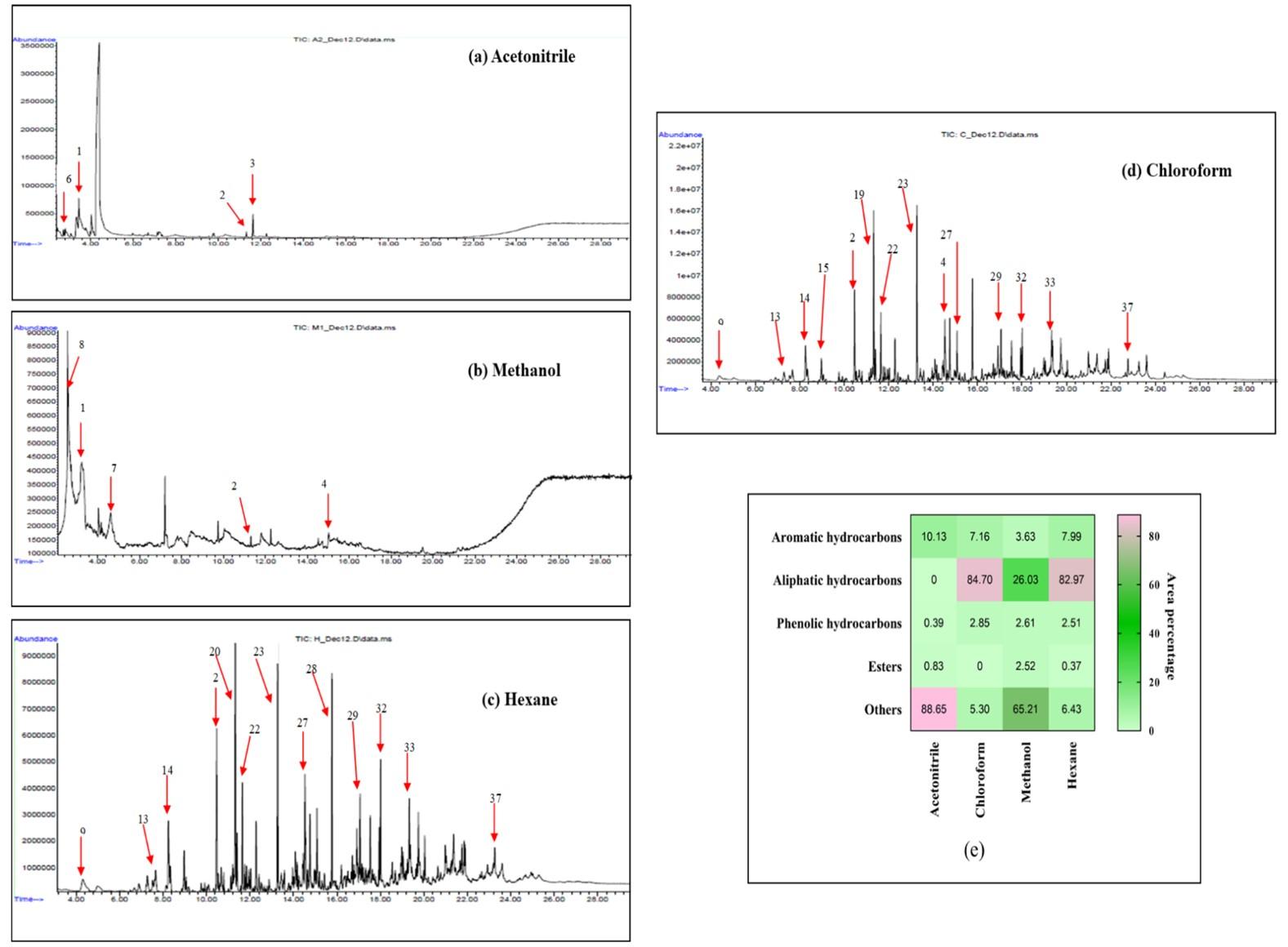
Table 4. Profile of intermediary degradatory products obtained through solvent extraction after MAA pretreatment.
Enzymatic hydrolysis of cellulose in AVLR biomass
In the current study, AVLR biomass that resulted in maximum delignification through MAA pretreatment (320 W) was subjected to the enzymatic hydrolysis by adding cellulase produced from the Aspergillus niger and Aspergillus sp., under SSF. The crude enzymes obtained with maximum cellulase activity were directly subjected to the saccharification process of MAA delignified AVLR biomass. After subjecting hydrolysis for 6 h with the pre-fixed conditions 1:18 (w/v) solid to liquid ratio, 50 °C, Aspergillus niger with 3.19 IU/mL (CMC-ase) and 0.42 (FPU/mL) activity has resulted in the reducing sugar yield of about 281.11 ± 1.43 mg/g, whereas, Aspergillus sp., resulted in 350.00 ± 2.32 mg/g of reducing sugar with 6.57 IU/mL CMC-ase and 0.89 FPU/mL activity as shown in Figure 4. Among the strains used, Aspergillus sp. resulted in a maximum saccharification percentage of about 68.5% ± 0.34 for treated biomass when compared to untreated biomass (24.26% ± 0.51) with a 2.8% of fold increase. Similarly, for Aspergillus niger it was observed that the saccharification percentage for treated AVLR sample was found to be 55% ± 2.50 than untreated sample (7.56% ± 0.02).
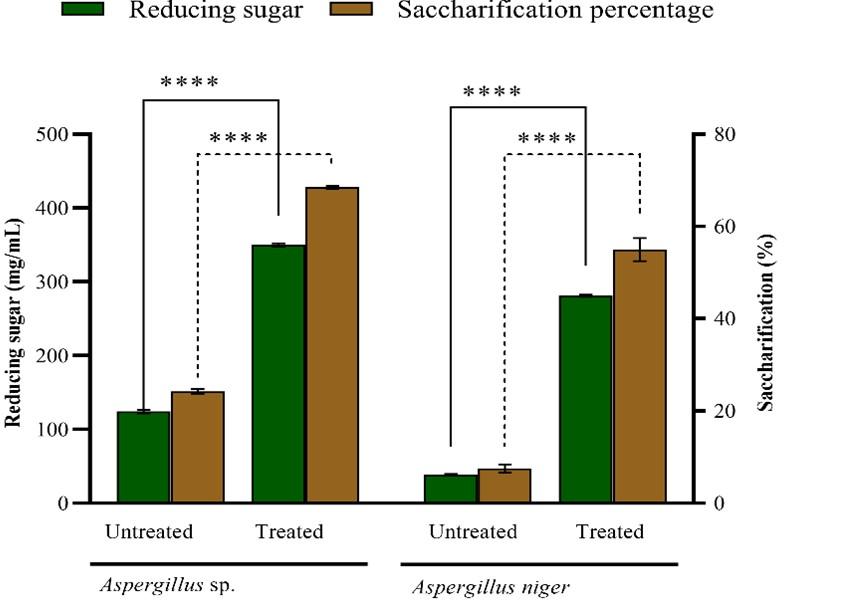
DISCUSSION
The main purpose of the study is to establish an efficient hybrid pretreatment strategy where MAA pretreatment for AVLR was performed to enhance the accessibility of cellulose for subsequent fermentation perhaps serve as a promising alternative strategy to the conventional acid pretreatment. AVLR, an industrial processing waste generated in copious amount from Aloe vera during gel extraction has no commercial application. The presence of a high fraction of holocellulosic components (cellulose and hemicellulose- 64.5%, w/w) affirmed that the AVLR biomass as a potent lignocellulose candidate for bioethanol production. However, the presence of significant quantity of lignin (13.95%, w/w) is a prime bottleneck in accessing of holocellulosic component for bioethanol production. Therefore, there is a requirement for an efficient pretreatment step to carry over saccharification and fermentation. The cell wall composition of AVLR utilized in this study was nearly equal to the previous studies [4] with slight variations. Seasonal, geographical differences and methods used for the structural component analysis might be the reason for the slight difference.
During MAA pretreatment, optimum microwave power (up to 480 W) has been utilized to avoid the wastage of energy [30] and achieve effective removal of AVLR lignin (66.38%, w/w) with a residual lignin of 4.68%, w/w by rapid oscillation of polar substance due to microwave irradiation. Likewise, Keshwani and Cheng found the lignin reduction of about 70% in switch grass and coastal Bermuda grass using MAA pretreatment [31]. This study showed the feasibility of utilizing NaOH (2%, w/v) for microwave pretreatment of AVLR biomass that could be capable of achieving almost similar depolymerization percentage (66%, (w/w)) while comparing with the acid microwave pretreatment of AVLR biomass [16]. A study by Nomanbhay et al. resulted in 74% of lignin removal when oil palm empty fruit bunch fiber was pretreated with 3% NaOH solution at 180 W for about 12 minutes [32]. Similarly, various studies on NaOH pretreatment assisted with microwave irradiation for rice straw has been carried out in order to increase the enzymatic hydrolysis and subsequent fermentation [21, 33]. Thus, the alkali (NaOH) assisted microwave pretreatment rather than using acid could circumvent the pre-requisite for the acid corrosion resistant reactor. The possible reason behind the solubilization of lignin in aqueous NaOH solution is that it could act as a microwave absorber and transfers the energy to the organic molecules thereby enhancing the efficiency of the delignification process.
Further, to elucidate the efficiency of MAA pretreatment of AVLR biomass, the structural and functional characterization of untreated and pretreated AVLR has been analyzed by SEM, FTIR and XRD analysis. In SEM analysis, the disintegration of cell wall matrix of AVLR biomass observed after MAA pretreatment might be due to the lignin solubilization [33]. Thus, it could enhance the surface area of the AVLR biomass, which ultimately increases the cellulase accessibility for the biomass degradation. According to Singh et al. the similar kind of morphological changes where distortion of cell wall was observed in the microwave- NaOH treated rice straw [21]. Based on the FTIR analysis, AVLR biomass with higher S type lignin, rich S/G lignin ratio and low guaiacyl units might be unsuitable for enzymatic hydrolysis due to its less affinity towards laccase (ligninolytic enzyme) [26]. Therefore, a hybrid pretreatment strategy like microwave assisted alkali delignification was adapted in this study and the result indicates that the MAA pretreatment was effective for the delignification of lignocellulosic biomass that can be used for subsequent saccharification.
Whereas the effectiveness of MAA pretreatment on inducing the changes in the cellulose crystallinity of AVLR biomass for enhanced enzymatic saccharification was studied using XRD diffraction patterns. The crI value of the MAA treated AVLR was observed to be increased with a microwave irradiation of 160 W and 320 W, further increase in irradiation power decreased the crI value. This might be due to the unique feature of the microwave heating which creates hotspots in heterogeneous complex of AVLR biomass that perhaps resulted in the disintegration of some useful components caused by explosion effect between particles at higher microwave power [34]. As observed from the GC-MS chromatogram, the alkyl substituted derivative compounds of monomeric phenols are present in the liquid hydrolysate of pretreated AVLR biomass which is mainly because of the aromatic nature of lignin that corroborated with the results obtained using ethanol reported by Cheng et al. during the study with alkali lignin [35]. In case of non-polar solvents, the liquid hydrolysate is rich in light organic fractions of aliphatic hydrocarbons (n-alkanes) and significantly fewer arenes [36, 37].
These degradatory compounds tapped through distillation and fractionation that could be further utilized for value-added products which provide an economic edge over the process. For example, benzaldehyde is used as a denaturant in cosmetics; pyridine and benzene were applied in the manufacture of dyes and rubber [38-40]. Additionally, MAA pretreatment of AVLR biomass resulted in no traces of toxic compounds such as furfural, 5-hydroxymethylfurfural and furan derivatives that were produced during acid microwave pretreatment [16] that could inhibit the subsequent saccharification and fermentation.
In order to compare the efficiency of cellulase producers, a standard cellulase producer i.e. Aspergillus niger was utilized in this study and the newly isolated species Aspergillus sp., is found to be potential candidate for saccharification. An enhanced saccharification (68.5%) observed in the MAA treated AVLR sample under un-optimized condition justifies the efficient lignin removal and providing a suitable exposed cellulose layer for saccharification without the formation of any toxic inhibitory compounds unlike in acid assisted microwave pretreatment resulted in 63% of saccharification [16]. Nomanbhay et al. reported that the reducing sugar yield of 411 mg/g when oil palm empty fruit bunch was subjected to one stage microwave pretreatment with 3% NaOH at 180 W for about 12 minutes [32]. Further optimization experiments are to be executed for saccharification to increase the fermentable sugar yield available for bioethanol production. Nevertheless, reducing sugar yield (350.00 ± 2.32 mg/g) obtained after MAA pretreatment is comparable to the reported studies on different lignocelluloses [41-43].
This study postulates that, MAA pretreatment of AVLR proceeded by the subsequent saccharification (MAA+ cellulase hydrolysis) was carried out for the first time. The presence of oxidized phenolic hydrocarbons in liquid hydrolysate after MAA pretreatment seem to be utilized in other high value-added product synthesis. With the global efforts of maintaining sustainable development, the utilization of natural products as well as industrial processing waste could be a good alternative and thereby AVLR finds a notable position as it could ensure a zero-waste policy. Thus, the adaptation of sustainable biorefinery based strategy for AVLR could balance both bioeconomy and ecology at the same time.
CONCLUSIONS
In this study, MAA pretreatment of AVLR biomass at 320 W has showed a significant removal of AVLR lignin by 66.38% and the subsequent cellulose hydrolysis by Aspergillus sp., increased the fermentable sugar release by 2.8-fold as compared to the untreated AVLR biomass. In addition, the intermediary degradation products obtained after MAA pretreatment could be used as value-added chemicals through its commercial utilization. Therefore, AVLR could serve as a suitable substrate for biofuel and value-added chemicals production thereby paving ways for sustainable zero waste utilization strategy.
ACKNOWLEDGEMENT
The authors would like to acknowledge the Department of Biotechnology, School of Bioengineering, SRM Institute of Science and Technology for the financial support provided for the research as well as infrastructure to carry out the research work.
AUTHORS CONTRIBUTION
GR has contributed to execution of the experiment, data generation, data analysis, and drafting the manuscript. SJ has provided the inputs towards the conceptualization, methodology, critical suggestion, and technical evaluation of the manuscript.
CONFLICTS OF INTEREST
There is no conflict of interest among the authors.
References
- [1]Upadhyay RK. Nutraceutical, therapeutic, and pharmaceutical potential of Aloe vera: A review. Int J Green Pharm. 2018. http://dx.doi.org/10.22377/ijgp.v12i01.160.1.
- [2]Grand view research report (2022). https://www.grandviewresearch.com/industry-analysis/aloe-vera-drinks-market (Accessed on June 04, 2022).
- [3]Farming India report (2019). https://www.farmingindia.in/aloe-vera-farming/ (Accessed on June 08, 2022).
- [4]Nizam NHM, Rawi NFM, Ramle SFM, Aziz AA, Abdullah CK, Rashedi A, Kassim MHM. Physical, thermal, mechanical, antimicrobial and physicochemical properties of starch-based film containing aloe vera: A review. J Mater Res Technol. 2021; 15: 1572–1589.
- [5]Hellen Sathya DJ, Turakhia AM, Kumar MA, Balaji N, Selvanaveen S, Vinodhini G et al. Bioethanol from saccharified lignocellulosic rich Aloe vera rinds using Saccharomyces cerevisiae MTCC 4779. Energy source part A, Recovery utilization and environmental effects. 2017; 39(13): 1347–1352.
- [6]Kim J-H, Cho S-M, Choi J-H, Jeong H, Lee SM, Koo B, Choi I-G. A simultaneous conversion and extraction of furfural from pentose in dilute acid hydrolysate of quercus mongolica using an aqueous biphasic system. Appl Sci. 2020; 11: 163.
- [7]Kumar AK, Sharma S. Recent updates on different methods of pretreatment of lignocellulosic feedstocks: A review. Bioresour Bioprocess. 2017.
- [8]Ethaib S, Omar R, Kamal SMM, Biak DRA, Zubaidi SL. Microwave-Assisted Pyrolysis of Biomass Waste: A Mini Review. Processes 2020; 8(9): 1190.
- [9]Li X, Shi Y, Kong W, Wei J, Song W, Wang S. Improving enzymatic hydrolysis of lignocellulosic biomass by bio-coordinated physicochemical pretreatment—A review. Energy Rep. 2022; 8: 696–709.
- [10]AOAC. Official methods of analysis. Association of Official Analytical Chemists, Inc. Maryland, USA.
- [11]Hussain MA, Huq ME, Rahman SM, Ahmed S. Estimation of lignin in jute by titration method. Pak J Biol Sci. 2002; 5(5): 521-522.
- [12]Marlett JA, Lee SC. Dietry fiber, lignocellulose and hemicellulose contents of selected foods determined by modified and unmodified van soest procedures. J Food Sci. 1980; 45(6): 1688–1893.
- [13]Sun JX, Sun XF, Zhao H, Sun RC. Isolation and characterization of cellulose from sugarcane bagasse. Polym Degrad Stabil. 2004; 84(2): 331-339..
- [14]Miller GL. Use of dinitrosalicylic acid reagent for determination of reducing sugar. Anal Chem. 1959; 31(3): 426-428.
- [15]Eveleigh DE, Mandels M, Andreotti R, Roche C. Measurement of saccharifying cellulase. Biotechnol Biofuels. 2009; 2: 21.
- [16]Rajeswari G, Arutselvy B, Jacob S. Delignification of Aloe vera Rind by Mild Acid Associated Microwave Pretreatment to Persuade Enhanced Enzymatic Saccharification. Waste Biomass Valor. 2019; 11: 5965–5975.
- [17]Li C, Knierim B, Manisseri C, Arora R, Scheller HV, Auer M et al. Comparison of dilute acid and ionic liquid pretreatment of switchgrass: Biomass recalcitrance, delignification and enzymatic saccharification. Bioresour Technol. 2010; 101(13): 4900–4906.
- [18]Varanasi P, Singh P, Auer M, Adams PD, Simmons BA, Singh S. Survey of renewable chemicals produced from lignocellulosic biomass during ionic liquid pretreatment. Biotechnol Biofuels. 2013; 28: 6(1)-14.
- [19]Mukhopadhyay M, Kuila A, Tuli DK, Banerjee R. Enzymatic depolymerization of Ricinus communis, a potential lignocellulosic for improved saccharification. Biomass Bioener. 2011; 35: 3584-3591.
- [20]Aggarwal N, Goyal V, Saini A, Yadav A, Gupta R. Enzymatic saccharification of pretreated rice straw by cellulases from Aspergillus niger BK01. 3 Biotech. 2017; 7: 158.
- [21]Singh R, Tiwari S, Srivastava M, Shukla A. Microwave Assisted Alkali Pretreatment of Rice Straw for Enhancing Enzymatic Digestibility. J Energy. 2014; 3: 1-7.
- [22]Trinsoutrot I, Monrozier LJ, Cellier J, Waton H, Alamercery S, Nicolardot B. Assessment of the biochemical composition of oilseed rape (Brassica napus L.) 13C-labelled residues by global methods, FTIR and 13C NMR CP/MAS. Plant and Soil. 2001; 234: 61–72.
- [23]Segal L, Creely JJ, Martin AE, Conrad CM. An Empirical Method for Estimating the Degree of Crystallinity of Native Cellulose Using the X-Ray Diffractometer. Text Res J 1969; 29: 786–794.
- [24]Manara P, Zabaniotou A, Vanderghem C, Richel A. Lignin extraction from Mediterranean agro-wastes: Impact of pretreatment conditions on lignin chemical structure and thermal degradation behavior. Catal Today. 2014; 223: 25–34.
- [25]Corredor DY, Salazar JM, Hohn KL, Bean S, Bean B, Wang D. Evaluation and Characterization of Forage Sorghum as Feedstock for Fermentable Sugar Production. Appl Biochem Biotech. 2009; 158(1): 164–179.
- [26]Avanthi A, Banerjee R. A strategic laccase mediated lignin degradation of lignocellulosic feedstocks for ethanol production. Ind Crops Prod. 2016; 92: 174–185.
- [27]Liu CF, Xu F, Sun JX, Ren JL, Curling S, Sun RC et al. Physicochemical characterization of cellulose from perennial ryegrass leaves (Lolium perenne). Carbohydr Res. 2006; 341(16): 2677–2687.
- [28]Fan M, Dai D, Huang B. Fourier Transform Infrared Spectroscopy for Natural Fibres. Fourier Transform – Materials Analysis. In Salih S (ed). IntechOpen. 2012.
- [29]Xu F, Yu J, Tesso T, Dowell F, Wang D. Qualitative and quantitative analysis of lignocellulosic biomass using infrared techniques: A mini-review. Appl Energ. 2013; 104: 801–809.
- [30]Ethaib S, Omar R, Kamal SMM, Biak DRA. Microwave-assisted pretreatment of lignocellulosic biomass: A review. J Eng Sci Technol. 2015; 10: 97-109.
- [31]Keshwani DR, Cheng JJ. Microwave-based alkali pretreatment of switchgrass and coastal bermudagrass for bioethanol production. Biotechnol Progr. 2009; 26(3): 644–652.
- [32]Nomanbhay SM, Hussain R, Palanisamy K. Microwave-Assisted Alkaline Pretreatment and Microwave Assisted Enzymatic Saccharification of Oil Palm Empty Fruit Bunch Fiber for Enhanced Fermentable Sugar Yield. J Sustain Bioenergy Syst. 2013; 3(1): 7-17.
- [33]Phitsuwan P, Permsriburasuk C, Baramee S, Teeravivattanakit T, Ratanakhanokchai K. Structural Analysis of Alkaline Pretreated Rice Straw for Ethanol Production. Int J Polym Sci. 2017.
- [34]Pang F, Xue S, Yu S, Zhang C, Li B, Kang Y. Effects of microwave power and microwave irradiation time on pretreatment efficiency and characteristics of corn stover using combination of steam explosion and microwave irradiation (SE–MI) pretreatment. Bioresour Technol. 2012; 118: 111–119.
- [35]Cheng S, Wilks C, Yuan Z, Leitch M, Xu C. (Charles). Hydrothermal degradation of alkali lignin to bio-phenolic compounds in sub/supercritical ethanol and water–ethanol co-solvent. Polym Degrad Stab. 2012; 97(6): 839–848.
- [36]Movil-Cabrera O, Rodriguez-Silva A, Arroyo-Torres C, Staser JA. Electrochemical conversion of lignin to useful chemicals. Biomass Bioener. 2016; 88: 89–96.
- [37]Piskorz J, Majerski P, Radlein D, Scott DS. Conversion of lignins to hydrocarbon fuels. Energ Fuel. 1989; 3: 723–726.
- [38]Cosmetic info report (2016). https://www.cosmeticsinfo.org/ingredients/benzaldehyde/ (Accessed on April 04, 2022).
- [39]New World Encyclopedia report (2015). https://www.newworldencyclopedia.org/entry/Pyridine (Accessed on April 04, 2022).
- [40]New World Encyclopedia report (2016). https://www.newworldencyclopedia.org/entry/Benzene (Accessed on April 04, 2022).
- [41]Gupta R, Mehta G, Khasa YP, Kuha RC. Fungal delignification of lignocellulosic biomass improves the saccharification of cellulosics. Biodegradation. 2011; 22(4): 797–804.
- [42]Han L, Feng J, Zhang S, Ma Z, Wang Y, Zhang X. Alkali pretreated of wheat straw and its enzymatic hydrolysis. Braz J Microbiol. 2012; 43(1): 1517-8382.
- [43]Kataria R, Ruhal R, Babu R, Ghosh S. Saccharification of alkali treated biomass of Kans grass contributes higher sugar in contrast to acid treated biomass. Chem Eng J. 2013; 230: 36–47.