Determining the disease outcome by cytokine storm during infectious diseases and targeting cytokines during sepsis: Possible therapeutic options
Abstract
The term ‘sepsis’ can be referred to as the dysfunction of organ(s) because of dysregulated and uncontrolled response of the host to that particular infection. As per statistics, more than 15 million sepsis cases have been recorded every year with around a 20% mortality rate. So, it is needless to mention the major threat this cytokine storm-induced syndrome is posing to public health throughout the world as both infectious and noninfectious diseases are involved with cytokine storm. A lot of evidence can be found on the major pathophysiological impact of cytokines during an infection, but no specific or effective treatment is available to target any inflammation effectively in sepsis. Numerous research has pointed out that it is possible to reduce the rate of mortality in severe sepsis by administering a low dosage of corticosteroids but unfortunately, no clinical benefits have been recorded during a large-scale clinical trial. But it is proven in a meta-analysis that anti-TNF treatment had been able to demonstrate a major reduction in the mortality rate of sepsis. The review would highlight some of the therapeutic interventions currently available to treat sepsis to get an overview. We would also focus on the association of cytokine storm in inducing sepsis.
INTRODUCTION
A wide range of pathogens originating from different sources might be the reason for sepsis causing the diagnosis and prognosis very complicated because of several symptoms [1]. Sepsis usually results from a bloodstream infection caused by bacteria and is considered to be a major clinical concern as it might cause a life-threatening situation or even organ dysfunction due to dysregulated immune response [2]. Such abnormally regulated immune response is apparent by the primary hyper-inflammatory response mainly driven by chemokines and cytokines [3]. Sepsis has been identified as one of the most serious clinical burdens with the estimated cost of hospitalizations at $38 billion as of 2017 which had been around $20 billion as of 2011 [4]. Among the pathogens that cause sepsis, the most prevalent ones include Klebsiella pneumoniae, Escherichia coli, Pseudomonas aeruginosa, Staphylococcus epidermidis, and Staphylococcus aureus [5]. Lower respiratory tract infections could lead to sepsis, and they are one of the most frequent nosocomial infections in ICU [1]. In terms of the usage of mechanical ventilation, the risk factors of developing lower respiratory tract infection increases which could also lead to sepsis, and based on statistics, ventilator-associated pneumonia can vary up to 66% of the patients requiring ventilation [6]. From the beginning of 1970, the pathogenesis of sepsis has been considered as the involvement of imprudent immune inflammation thus suggesting the importance of the downregulation of immunity [7]. Cytokines are one of the most dominant pleiotropic regulators of immunity and they play a major role in the complicated pathophysiology of sepsis having both the pro-inflammatory and anti-inflammatory properties that are proficient to coordinate defense mechanisms for invading pathogens [8]. At the same time, they might dysregulate the immune response while promoting inflammation resulting in tissue damage [8]. A wide range of inflammatory responses is regulated by cytokines that include the migration of certain immune cells to the location of the infection which is highly required for the prevention of systemic infection. But if the release of cytokines is poorly regulated, endothelial dysfunction could occur which would be characterized by increased capillary permeability as well as vasodilation; eventually causing hemoconcentration, hypertension, edema, and macromolecular extravasation [9].
The relation between sepsis and cytokine storm has been considered to be essential in the discovery of effective therapeutic interventions. Focusing on this aspect would allow researchers to unveil a different outlook on cytokines and their elevated levels in sepsis patients [12]. Since growth factors play a major role in the sepsis pathogenesis and the colony stimulating factors induce cell proliferation, the escalating cells are responsible for the increased levels of cytokines [31]. The involvement of other inflammatory cytokines such as IL-6, IL-7, IL-12 and IL-1β is also evident in sepsis [12]. With rigorous research on the interconnectedness between cytokine storm and sepsis, it could be possible to pave the path toward the implementation of novel potential treatments in reducing the mortality rate of sepsis.
The lack of clinically predictive and relevant animal models happens to be one of the major obstacles to the development of effective therapeutics in treating sepsis; nevertheless, more than hundreds of animal models have been used by researchers over the past few years [80]. But some therapeutics agents have shown positive results in sepsis treatment whereas the traditional interventions revealed some crucial adverse impacts; for instance, high dose corticosteroids worsen the disease outcome in sepsis patients by making them vulnerable to several secondary infections [60]. That is why it is very important to assess the therapeutic interventions and weigh their negative impacts against the positive ones before their initial implementation in sepsis.
The initial treatment for sepsis is administering a wide range of antibiotics though a very specific therapeutic intervention would be required especially in terms of negative blood culture where the causative agent of the infection remains unknown while avoiding increased microbial resistance to antibiotics available now [10]. IG therapy, anti-TNF mAb, and the usage of CSFs could be effective though a wide range of controversies remains regarding their effectiveness in reducing sepsis mortality [1]. Apart from that, the usage of moderate-dose steroids, limiting the overall tidal volume in lung complications, early goal-directed therapy, and intensive insulin therapy could have some positive impact in reducing sepsis-related death rates as well [11].
RELATION BETWEEN CYTOKINE STORM AND SEPSIS
The term ‘cytokine storm’ sometimes can also be denoted as ‘cytokine cascade’ which happens to be one of the main reasons for the diverse, remote, and local signs that are associated with an infection. At long last, if the threshold is crossed somehow, sepsis could be observed which has a major impact on both mortality and morbidity [12]. The very first association of cytokine storm had been observed in Graft Versus Host Disease (GVHD) back in 1993 [13]. After that, a major interconnectedness has been observed between cytokine storm and influenza [14]. Later studies have revealed its association with a wide range of fungal, bacterial as well as viral infections [15]. Cytokines can be termed as small proteins weighing less than 80 kDa [16] and they can be categorized into various groups including interleukins, interferons, chemokines, growth factors, and tumor necrosis factors [12]. One of the main actions of interleukins is in the growth and differentiation of leukocytes, interferons regulate the innate immunity while activating different antiviral properties, chemokines control the whole process of chemotaxis and the recruitment of leukocytes, and tumor necrosis factors activate the cytotoxic T lymphocytes [15].
The term ‘interleukin’, sometimes denoted as IL had been selected in describing a particular group of proteins that are produced by either the tissue macrophages or monocytes for mediating communication between associated leukocytes [17]. IL-1 was the very first interleukin to be brought to light, which had been identified as one of the most essential factors enhancing the T-cell responses to antigens or mitogens [18]. Interleukins are one of the most essential ones released in the infectious processes. The artificial categorization of interleukins can be divided into pro-inflammatory interleukins and anti-inflammatory interleukins. During sepsis, the involvement of several inflammatory cytokines including IL-1β, IL-12, IL-6, and IL-7 can be seen [12]. Apart from that, the levels of IL-1β had been noticed to be higher in the patients who have died in comparison with the survivors [19].
IL-6 is considered pleiotropic and their role in cytokine storm has always been very complex. Several inflammatory diseases including autoimmune diseases, cardiovascular diseases, and cancer were observed to have high IL-6 levels. Moreover, IL-6 had also been shown high association with the severity of sepsis, and with a higher number of IL-6 demonstrated much worse outcomes among the sepsis patients [20]. The interconnectedness between sepsis and IL-6 could be associated with the activation of complement pathways [21].
During sepsis, IL-12 is also increased [12]. They are produced by macrophages and dendritic cells. And because of this reason, they induce huge loads of INFγ [22]. IFN-γ is an effector cytokine that contributes substantially to immunity [23]. They are secreted by activated lymphocytes such as CD8 cytotoxic T cells and CD4 Th1 [24]. It is worth mentioning that IFN-γ associated signaling could have suppressive immune regulatory impacts on antitumor [25], autoimmune [26], and antiviral [27] responses. INFγ has demonstrated its ability to promote inflammatory responses but its production is reduced in sepsis [28]. It could happen because of the hyporesponsiveness of lymphocytes mostly in an immunosuppressed state [29]. IL-3 is identified as one of the key regulators of cytokine storm during sepsis [30]. IRA B cells produce the IL-3, and they fuel the inflammatory cascade with the promotion of emergency myelopoiesis [12].
Growth factors are also highly associated with the pathogenesis of sepsis. Among a wide range of growth factors secreted in sepsis, the most associated ones in inducing cytokine storm include hematopoietic targeted granulocyte-macrophage colony-stimulating factors, granulocyte colony-stimulating factors, as well as macrophage colony-stimulating factors. However, in addition to IL-3, colony-stimulating factors would induce myeloid cell proliferation and differentiation [12]. Because of this reason, a huge load of activated cells would contribute to the synthesis of elevated cytokines; thus, promoting cytokine storm [31]. Figure 1 would depict the cytokine cascade in sepsis.
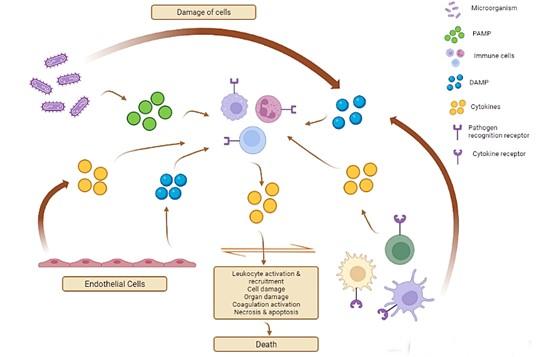
INFECTIONS ASSOCIATED WITH CYTOKINE STORM
Cytokine storms are involved with a wide range of infectious and noninfectious diseases, and it does not bring fortunate consequences in therapeutic interventions [32]. Cytokine storm was previously known as an influenza type syndrome occurring after the systemic infection including sepsis and after immunotherapy including Coley’s toxins [33]. Infections associated with Yersinia pestis had been one of the reasons for major pandemics such as the Black Death while triggering the alveolar macrophage in producing huge loads of cytokines leading to cytokine storm [34]. A wide range of disorders is resulting in from cytokine storm like primary and secondary hemophagocytic lymphohistiocytosis, sepsis, COVID-19, and associated autoinflammatory disorders [35].
Most of the patients with cytokine storm seem to be feverish and might also have a fever in terms of severe cases [36]. Moreover, patients could have fatigue, headache, anorexia, rash, myalgia, diarrhea as well as arthralgia. All these symptoms could be present because of the tissue damage by the cytokine storm. However, the acute phase physiological alternations and immune cell-mediated responses could also be responsible for this aspect. Some of the patients could also have respiratory disorders such as tachypnea and cough that could lead to acute respiratory distress syndrome (ARDS) requiring mechanical ventilation [35]. Moreover, the combination of coagulopathy, hyper-inflammation, and low counts of platelet the patients with cytokine storms could increase the risk of spontaneous hemorrhage. In severe cases, acute liver injury, renal failure, and stress-related cardiomyopathy could develop as well [37]. The combination of endothelial cell death, renal dysfunction, and acute phage hypoalbuminemia could lead to anasarca and capillary leak syndrome [38].
The term cytokine storm was first used in infectious diseases back in 2000 when research began on cytomegalovirus [39], severe acute respiratory syndrome (SARS-CoV) [40], a group of Streptococcus [41] as well as variola virus [42]. Moreover, rigorous studies had been carried out on cytokine storm because of the public interest in bird flu [15]. Even though the term had not been stated explicitly, studies have addressed potential molecular and cellular mechanisms that are contributing to cytokine storm in several viral diseases [43]; and the focus was given to influenza specifically [44].
Cytokine storm and COVID-19
The macrophages [81] and dendritic cells are primarily responsible for triggering the initial response in SARS-CoV-2, which also include the release of cytokine [82]. Additionally, the inflammatory response contributes to the destruction of lymphocytes in order to stop the infection of SARS-CoV-2. The activation of NOD, LRR-, and pyrin domain-containing protein 3; also abbreviated as NLRP-3 inflammasome alongside the dull response of histone deacetylase 2 on the Nuclear Factor Kappa Betta (NF-κB) complex is pointing out the association with cytokine storm [82]. The pathophysiological mechanisms concerning SARS-CoV-2 induced cytokine storm are illustrated in Figure 2.
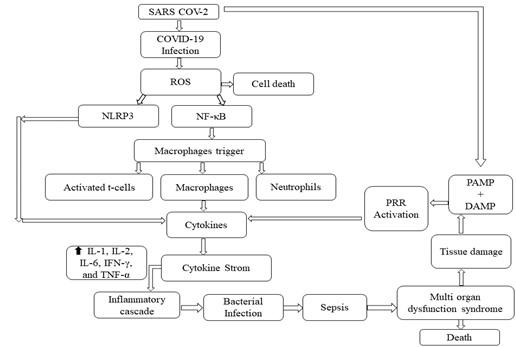
SEPSIS: THERAPEUTIC INTERVENTIONS
The most effective strategy for addressing cytokine storm is associated with the supportive care in maintaining the functions of critical organs, controlling the underlying health complications while eliminating the possible triggers that could activate an abnormal immune system all for limiting the collateral damages caused by the activated immune system [35]. Substantial progress has been identified in the progress of therapeutic interventions in reducing the mortality rate in sepsis. Some of the most effective ones include limiting the tidal volume in Acute Respiratory Distress Syndrome (ARDS) or Acute Lung Injury (ALI), early goal-directed therapy, stem cell therapy, Ig therapy, and usage of moderate dosage of steroids [11].
ALI can be seen in more than 25% of patients with sepsis [45]. A tidal volume of 6 ml/kg of the body weight (ideal) happens to be at the lower end range of physiologic ventilation; even though it has been tested on ALI and ARDS patients only [11]. Since patients with severe sepsis develop ALI and ARDS, low tidal volume therapy could prevent the further progression of these conditions. Early Goal-Directed Therapy (EGDT) is also effective against sepsis. The main purpose of this therapy is to adjust the cardiac preload, afterload as well as contractility in balancing the systemic oxygen delivery. Patients with severe sepsis have shown a positive response in maintaining proper cellular perfusion while preventing organ dysfunction [11].
Mesenchymal Stem Cells (MSCs) have several anti-immune regulatory and anti-inflammatory roles as it has the potential for the inhibition of the secretion of cytokines (pro-inflammatory) including IL-1, IL-12, IL-6, IFN-γ, and TNF-α; thus, suppressing the cytokine storm activation [46]. Moreover, mesenchymal stem cells can secrete IL-10, hepatocyte, and keratinocyte which would assist collectively to inhibit the formation of fibrosis while repairing the damaged tissues of the lung [47]. Ig therapy is proven to facilitate the systemic neutralization and opsonization of bacteria, nonetheless, the success rate in clinical trials is only modest [1]. But because of very minimal certainty in reducing sepsis mortality [48], the Surviving Sepsis Guideline panel stopped recommending in 2016 [49].
The impact of steroids in treating patients with sepsis has been highly debated for a long time. Several randomized, well-designed and controlled trials have failed to show positive impacts of steroids in improving the survival of the patients with sepsis [50]. But some strong evidence highlights the usage of steroids in patients diagnosed with refractory septic shock. Moderate dosages of steroids might restore the sensitivity of cells to vasopressors [51]. It could potentially reduce the intensity of inflammatory responses while decreasing the possibility of organ dysfunction. That is why low-dose steroid therapy could be well tolerated [52]. A lower dose has the potential to induce a greater response as per a meta-analysis [53].
In patients with ARDS, the exogenous corticosteroid administration entirely blocked the NFκb in the lungs [54]. Corticosteroids have also been proven to be effective in suppressing the renal iNOS activities right after endotoxemia for preventing hypoxic injury; thus, improving the renal oxygen delivery and restoring oxygen consumption [55]. Moreover, corticosteroids also improved the glomerular endothelium permeability among patients with septic shock [56]. The positive effects of corticosteroids on organ perfusions have also been proven for the brain [57] and heart [58]. Even though several positive aspects can be observed in the administration of corticosteroids in sepsis patients, the rationale for its usage is dependent on the concepts of critical health deterioration concerning the insufficiency of corticosteroids [59]. It is worth mentioning that high dose corticosteroids (30 mg of methylprednisolone/kg of body weight) do not have any positive impact on sepsis patients, and it does not improve the survival rate either; instead, worsen the situation by making the patient more susceptible to secondary infections [60].
Apart from that, different colony-stimulating factors like Granulocyte-Macrophage Colony Stimulating Factor (GM-CSF) and Granulocyte-Colony Stimulating Factor (G-CSF) have been examined as therapeutics in increasing the macrophage and neutrophil number for improving the bacterial clearance process in sepsis [1]. But again, only modest improvement had been seen in the patient recovery [61], mostly among the patients who had sepsis-related immunosuppression [62].
Researchers have identified the positive impact of intensive insulin therapy for hyperglycemia in sepsis patients. It is demonstrated that if the glucose level of blood is maintained at 4.4 to 6.1 mmol/liter (80-110 mg/deciliter), it is possible to reduce mortality and morbidity among the patients who have been critically ill and received conventional therapy [63]. The frequency of episodes of sepsis was reduced to 46 percent by intensive insulin therapy and the patients with bacteremia treated with this intensive insulin therapy had low mortality compared to the patients who received conventional therapy [64]. Moreover, the death rate caused by a multiple-organ failure in sepsis was also reduced in insulin therapy regardless of the diabetes history of the patients. The mechanism associated with the antiapoptotic impact of insulin also plays a major role [65]. Insulin is effective in preventing apoptotic cell death from several stimuli as it activates the phosphatidylinositol 3-kinase-Akt pathway [66].
Anti-TNF treatment
Tumor necrosis factor (TNF) is a protein weighing 17 kDa and made up of more than 150 amino acids; being produced by activated T lymphocytes, natural killer (NK) cells, and macrophages [67]. Research has shown that TNF might be the reason for the symptoms associated with both endotoxemia and malaria [68]. Cytotoxic factors are released during infection, and it is seen that TNF had been capable of inducing tissue injury and shock [69]; while the anti-TNF antibodies could prevent the lethality and complexities caused by bacteremia [70]. Because of this reason, anti-TNF treatment could be one of the most effective therapeutic interventions in reducing the sepsis mortality rate [71]. Some of the chosen inhibitors of tumor necrosis factors have been proven effective in limiting inflammation and joint injury to manage rheumatoid arthritis [72]. During their therapeutic development, similar anti-TNF agents had been tested in sepsis [73]. The preclinical studies on sepsis had shown that the level of TNF was elevated while some of the inhibition of TNF prevented deaths [74].
But ongoing investigations have pointed out that, selective anti-TNF agents produced remarkable survival benefits among sepsis patients. These agents are still undergoing vast investigation. Apart from that, results have shown that if patients with severe sepsis are given immunotherapy with associated anti-TNF agents, it is possible to reduce the overall patient mortality. In patients with shock or high IL-6, anti-TNF therapy has been proven to reduce the death rate though it was not statistically significant. [75]. However, one of the major setbacks of the assessment of anti-TNF factor as an effective therapeutic intervention is associated with large scale trials which could be logistically complicated [71].
Over the past decades, hundreds of diverse animal models have been used by the researchers for sepsis and the studies have been used as a major developmental pathway for new sepsis therapeutic agents [80]. Mice can be seen as one of the most common animals used in sepsis models as the inbred strains are available and they pose little to no threat to anyone working in the laboratory. However, the usage of genetically modified strains creates a great opportunity in exploring the significance of specific gene products in sepsis pathogenesis [80]. The below table would highlight some of the pharmacological agents that have been evaluated in the sepsis animal model with the potential results.
Table 1. Sepsis animal model.
POTENTIAL MANAGEMENT STRATEGY AGAINST CYTOKINE STORM
Sepsis patients are sometimes treated in intensive care facilities since they need intense therapeutic support and close monitoring [93]. Among some of the early treatments in addressing the issue, blood purification techniques have been implemented [94]. Some of the most common ones include haemoperfusion, haemofiltration, continuous or intermittent high-volume haemofiltration as well as plasmapheresis. The main purpose of such approaches is to ensure ‘immune homeostasis’ that reduces the damage caused by the host’s dysregulated response to a particular infection [95]. It could be heralded by a significant rise of cytokines, contributing to the severe systemic impact of sepsis mostly in septic shock [96].
The usage of CytoSorb ® in cytokine haemoadsorption in sepsis and septic shock had been discussed by a panel of clinicians. It is a sorbent technology for reducing cytokine levels. The CytoSorb ® device is made up of a single use haemoadsorption cartridge that is possible to use with standard blood pumps like a haemoperfusion device [97]. The CytoSorb cartridge is a CE-marked device that is capable of lowering elevated cytokines in severe sepsis [95]. The cartridge is usually filled with sorbent beads which are made from porous polymers that ensure capturing and adsorbing cytokines when the blood is passed through the device. The whole process is dependent on concentration and because of this reason, if the blood contains higher levels of cytokines, the level would be reduced faster [95]. The removal of cytokines had been confirmed in a feasibility study and the rate of cytokine extraction had been 30% [98]. One of the major findings of the study was that the plasma concentration of both TNF-α and IL-6 had been reduced after the first hour of the therapy, but the concentration of IL-10 was not reduced. Moreover, the most significant removal was noticed in IL-6; whereas the least had been the tumor necrosis factors [98].
In septic shock, the usage of haemoadsorption with CytoSorb has been supported by several experimental evidence; nevertheless, the evidence is not significant from human studies. More than 100 cases have been identified where the CytoSorb was used in clinical scenarios and the treatment had been well-tolerated though the clinical studies have not been conducted on large scale [99]. Patients with high severity of illness would be more likely to benefit from the intervention [100]. A refractory shock that has been indicated by high doses of vasopressor support alongside early organ failure with cytokine storm must be preferred as well [101].
FUTURE PROSPECTS
The current therapeutics and clinical trials in reducing cytokine storm have not been effective and failed to decrease the inflammatory responses even with the advancement in transcriptomic, genomics, metabolomics, and proteomic progress of sepsis [12]. The proposed study would be helpful for researchers to get further insight into the association of sepsis and cytokine storm while proposing effective therapeutics. Since a complete overview of the orchestration of cytokine storm is required for highly effective therapeutics, the study could be of help by taking into consideration this aspect.
It is very important to understand the cytokine profile in sepsis patients as it could be effective in assessing the severity of the disease and predicting mortality, resulting in much better patient management. Targeting cytokines during sepsis is still considered one of the major therapeutic challenges despite the huge amount of explanation on the pathophysiology of cytokines in an infection. This study would focus more on the unexplored aspects of cytokine targeting in sepsis. Corticosteroids have been used as a therapeutic intervention that decreases inflammation while inhibiting the activation of both the AP-1 and NF-κB pathways [76]. Several studies have identified that a low dosage of corticosteroids has the potential to reduce mortality in both severe septic shock and sepsis [77]. But unfortunately, large-scale clinical trials did not show any clinical benefits in the systematic usage of corticosteroids in sepsis [78]. Some targeted therapies have also been considered and tested. Even though the capture of compounds including IL-1, LS, and TNF-α has shown positive preclinical results in mice models but failed to reduce the sepsis mortality [79]. The study would also focus on assessing different therapeutic interventions that have already been tested and compare their positive and negative impact on reducing sepsis mortality in cytokine storm.
A wide range of therapeutic interventions are clinically available for treating patients with sepsis, but it is important to assess the adverse impact they could deteriorate the patient’s health conditions. The review accumulates some prosperous and promising interventions in reducing the mortality of sepsis and some of them are focused on sorbent technology that helps lower the cytokine levels [97]. Moreover, the study is also highlighting the association between cytokine storm and sepsis while depicting some of the mechanisms of infections associated with cytokine storm. Future researchers interested who are interested in discovering effective therapeutic interventions could be guided by this research since an overview of sepsis pathogenesis in association with cytokine storm would be provided to them alongside the existing therapeutic interventions and cytokine storm management strategy. The progress of this review can be evaluated by the organization of information on sepsis pathogenesis, the involvement of elevated cytokines and the management strategies in reducing the death rate. With more research on sepsis, it would be possible to develop promising immunomodulating treatment strategies while unveiling unexplored and undiscovered pathophysiological features of sepsis [8].
CONCLUSIONS
Sepsis is still one of the major concerns for both researchers and clinicians. Even though rigorous studies have carried on throughout the years, but no substantial therapeutic development has been observed in large clinical trials. One of the main reasons is associated with the lack of adequate understanding of the pathophysiology and interconnectedness with cytokine storm since it is characterized as a dynamic and complex disease process. Moreover, heterogeneous patients are affected by sepsis with diverse comorbidities and etiologies; making the effective discovery of potential therapeutics more challenging and aggravating. Researchers have been unveiling all associated pathophysiologic processes in sepsis and the essential regulatory roles played by cytokines in the progression of the disease. As of now, intensive insulin therapy, anti-TNF treatment and low dosage of steroid administration are giving a ray of hope, nonetheless having setbacks in large-scale clinical trials. We hope that the ongoing research in this aspect would help expand our knowledge of the mechanism of the disease while developing novel strategies to fight sepsis which would bring only positive results in the clinical trials.
ACKNOWLEDGMENT
We would like to show our gratitude to the corresponding author Mr. Fahd Bin Zahed, core faculty member of the Department of Biochemistry and Microbiology, North South University, Dhaka, Bangladesh for his remarkable assistance in nurturing the idea that substantially enhanced the quality of the manuscript.
AUTHOR CONTRIBUTIONS
FBZ conceived the idea and prepared the outline of the review. TA and AH performed the literature search and data extraction, analysis of extracted data and manuscript preparation. FBZ supervised the manuscript preparation and prepared the final draft. All authors read and accepted the final version of the manuscript.
CONFLICTS OF INTEREST
There is no conflict of interest among the authors.
References
- [1]Greenfield KG, Badovinac VP, Griffith TS, Knoop KA. Sepsis, cytokine storms, and immunopathology: the divide between neonates and adults. ImmunoHorizons, 2021. 5(6): 512–522.
- [2]Shankar-Hari M, Phillips GS, Levy ML, Seymour CW, Liu VX, Deutschman CS, Angus DC, Rubenfeld G D, Singer M. Developing a New Definition and Assessing New Clinical Criteria for Septic Shock. JAMA, 2016, 315(8): 775.
- [3]van der Poll T, van de Veerdonk FL, Scicluna BP, Netea, MG. The immunopathology of sepsis and potential therapeutic targets. Nature Reviews Immunology, 2017, 17(7): 407–420.
- [4]Rudd KE, Johnson SC, Agesa KM, Shackelford KA, Tsoi D, Kievlan DR, Colombara DV, Ikuta KS, Kissoon N, Finfer S, Fleischmann-Struzek C, Machado FR, Reinhart KK, Rowan K, Seymour CW, Watson, R. S., West, T. E., Marinho, F., Hay, S. I., & Lozano, R. Global, regional, and national sepsis incidence and mortality, 1990–2017: analysis for the Global Burden of Disease Study. The Lancet, 2020. 395(10219): 200–211.
- [5]Dolin, H. H., Papadimos, T. J., Chen, X., & Pan, Z. K. Characterization of Pathogenic Sepsis Etiologies and Patient Profiles: A Novel Approach to Triage and Treatment. Microbiology Insights, 2019: 12.
- [6]Soussan, R., Schimpf, C., Pilmis, B., Degroote, T., Tran, M., Bruel, C., & Philippart, F. Ventilator-associated pneumonia: The central role of transcolonization. Journal of Critical Care, 2019, 50, 155–161.
- [7]Llewelyn, M., & Cohen, J. Superantigens: microbial agents that corrupt immunity. The Lancet Infectious Diseases, 2002, 2(3), 156–162.
- [8]Schulte, W., Bernhagen, J., & Bucala, R. Cytokines in Sepsis: Potent Immunoregulators and Potential Therapeutic Targets—An Updated View. Mediators of Inflammation, 2013, 1–16.
- [9]Rivers, E., Nguyen, B., Havstad, S., Ressler, J., Muzzin, A., Knoblich, B., Peterson, E., & Tomlanovich, M. Early Goal-Directed Therapy in the Treatment of Severe Sepsis and Septic Shock. New England Journal of Medicine, 2001. 345(19), 1368–1377.
- [10]Textoris, J., Wiramus, S., Martin, C., & Leone, M. Overview of antimicrobial therapy in intensive care units. Expert Review of Anti-Infective Therapy, 2011, 9(1), 97–109.
- [11]Vincent, J.-L., Abraham, E., Annane, D., Bernard, G., Rivers, E., & Van den Berghe, G. (2002). Critical Care, 6(Suppl 3), S1.
- [12]Chousterman, B. G., Swirski, F. K., & Weber, G. F. Cytokine storm and sepsis disease pathogenesis. Seminars in Immunopathology, 2017. 39(5), 517–528.
- [13]Ferrara, J. L., Abhyankar, S., & Gilliland, D. G. Cytokine storm of graft-versus-host disease: a critical effector role for interleukin-1. Transplantation Proceedings, 1993. 25(1 Pt 2), 1216–1217.
- [14]Yokota, S. [Influenza-associated encephalopathy–pathophysiology and disease mechanisms]. Nihon Rinsho. Japanese Journal of Clinical Medicine, 2003. 61(11), 1953–1958.
- [15]Tisoncik, J. R., Korth, M. J., Simmons, C. P., Farrar, J., Martin, T. R., & Katze, M. G. Into the Eye of the Cytokine Storm. Microbiology and Molecular Biology Reviews, 2012. 76(1), 16–32.
- [16]Chung, K. F. Cytokines. Asthma and COPD. Asthma and COPD (Second Edition), 2009. 327–341. https://doi.org/10.1016/b978-0-12-374001-4.00027-4
- [17]Trotta, P. P. Cytokines: An Overview. American Journal of Reproductive Immunology, 1991. 25(3), 137–141.
- [18]Gery, I., & Waksman, B. H. Potentiation of the T-lymphocyte response to mitogens. Journal of Experimental Medicine, 1972. 136(1), 143–155.
- [19]Mera, S., Tatulescu, D., Cismaru, C., Bondor, C., Slavcovici, A., Zanc, V., Carstina, D., & Oltean, M. Multiplex cytokine profiling in patients with sepsis. APMIS, 2010. 119(2), 155–163.
- [20]Gouel-Chéron, A., Allaouchiche, B., Guignant, C., Davin, F., Floccard, B., & Monneret, G. Early Interleukin-6 and Slope of Monocyte Human Leukocyte Antigen-DR: A powerful association to predict the development of sepsis after major trauma. 2012. PLoS ONE, 7(3), e33095.
- [21]Riedemann, N. C., Guo, R., Hollmann, T. J., Gao, H., Neff, T. A., Reuben, J. S., Speyer, C. L., Sarma, J. V., Wetsel, R. A., Zetoune, F. S., & Ward, P. A. Regulatory role of C5a in LPS‐induced IL‐6 production by neutrophils during sepsis. The FASEB Journal, 2003, 18(2), 1–16.
- [22]Hsieh, C., Macatonia, S., Tripp, C., Wolf, S., O’Garra, A., & Murphy, K. Development of TH1 CD4+ T cells through IL-12 produced by Listeria-induced macrophages. Science, 1993. 260(5107), 547–549.
- [23]Castro, F., Cardoso, A. P., Gonçalves, R. M., Serre, K., & Oliveira, M. J. Interferon-Gamma at the Crossroads of Tumor Immune Surveillance or Evasion. Frontiers in Immunology, 2018. 9.
- [24]Kasahara, T., Hooks, J. J., Dougherty, S. F., & Oppenheim, J. J. Interleukin 2-mediated immune interferon (IFN-gamma) production by human T cells and T cell subsets. The Journal of Immunology, 1983, 130(4), 1784–1789.
- [25]Lee, S.-J., Jang, B.-C., Lee, S.-W., Yang, Y.-I., Suh, S.-I., Park, Y.-M., Oh, S., Shin, J.-G., Yao, S., Chen, L., & Choi, I.-H. Interferon regulatory factor-1 is prerequisite to the constitutive expression and IFN-γ-induced upregulation of B7-H1 (CD274). FEBS Letters, (2006). 580(3), 755–762. 3
- [26]Ferber, I. A., Brocke, S., Taylor-Edwards, C., Ridgway, W., Dinisco, C., Steinman, L., Dalton, D., & Fathman, C. G. Mice with a disrupted IFN-gamma gene are susceptible to the induction of experimental autoimmune encephalomyelitis (EAE). The Journal of Immunology, (1996).156(1), 5–7.
- [27]Shrestha, B., Wang, T., Samuel, M. A., Whitby, K., Craft, J., Fikrig, E., & Diamond, M. S. Gamma Interferon Plays a Crucial Early Antiviral Role in Protection against West Nile Virus Infection. Journal of Virology, 2006. 80(11), 5338–5348.
- [28]Ono, S., Ueno, C., Aosasa, S., Tsujimoto, H., Seki, S., & Mochizuki, H. Severe sepsis induces deficient interferon-gamma and interleukin-12 production, but interleukin-12 therapy improves survival in peritonitis. The American Journal of Surgery, (2001).182(5), 491–497.
- [29]Romero, C. R., Herzig, D. S., Etogo, A., Nunez, J., Mahmoudizad, R., Fang, G., Murphey, E. D., Toliver-Kinsky, T., & Sherwood, E. R. The role of interferon-γ in the pathogenesis of acute intra-abdominal sepsis. Journal of Leukocyte Biology, (2010). 88(4), 725–735.
- [30]Weber, G. F., Chousterman, B. G., He, S., Fenn, A. M., Nairz, M., Anzai, A., Brenner, T., Uhle, F., Iwamoto, Y., Robbins, C. S., Noiret, L., Maier, S. L., Zönnchen, T., Rahbari, N. N., Schölch, S., Klotzsche-von Ameln, A., Chavakis, T., Weitz, J., Hofer, S., & Weigand, M. A. Interleukin-3 amplifies acute inflammation and is a potential therapeutic target in sepsis. Science, (2015). 347(6227), 1260–1265.
- [31]Hamilton, J. A. Colony-stimulating factors in inflammation and autoimmunity. Nature Reviews. Immunology, (2008). 8(7), 533–544.
- [32]Suntharalingam, G., Perry, M. R., Ward, S., Brett, S. J., Castello-Cortes, A., Brunner, M. D., & Panoskaltsis, N. Cytokine Storm in a Phase 1 Trial of the Anti-CD28 Monoclonal Antibody TGN1412. New England Journal of Medicine, (2006). 355(10), 1018–1028.
- [33]COLEY, W. B. The treatment of malignat tumors by repeated inoculations of erysipelas. The American Journal of the Medical Sciences, 1893, 105(5), 487–510.
- [34]Pechous, R. D., Sivaraman, V., Price, P. A., Stasulli, N. M., & Goldman, W. E. Early Host Cell Targets of Yersinia pestis during Primary Pneumonic Plague. PLoS Pathogens, (2013).9(10), e1003679.
- [35]Fajgenbaum, D. C., June, C. H. Cytokine Storm. New England Journal of Medicine, (2020). 383(23), 2255–2273.
- [36]Porter, D. L., Levine, B. L., Kalos, M., Bagg, A., & June, C. H. Chimeric Antigen Receptor–Modified T Cells in Chronic Lymphoid Leukemia. New England Journal of Medicine, (2011). 365(8), 725–733.
- [37]Templin, C., Ghadri, J. R., Diekmann, J., Napp, L. C., Bataiosu, D. R., Jaguszewski, M., Cammann, V. L., Sarcon, A., Geyer, V., Neumann, C. A., Seifert, B., Hellermann, J., Schwyzer, M., Eisenhardt, K., Jenewein, J., Franke, J., Katus, H. A., Burgdorf, C., Schunkert, H., & Moeller, C. Clinical Features and Outcomes of Takotsubo (Stress) Cardiomyopathy. New England Journal of Medicine, (2015). 373(10), 929–938.
- [38]Schwartzentruber, D. J. Guidelines for the Safe Administration of High-Dose Interleukin-2. Journal of Immunotherapy, (2001). 24(4), 287–293.
- [39]Barry, S. M., Johnson, M. A., Janossy, G. Cytopathology or immunopathology? The puzzle of cytomegalovirus pneumonitis revisited. Bone Marrow Transplantation, (2000). 26(6), 591–597.
- [40]Huang, K.-J., Su, I.-J., Theron, M., Wu, Y.-C., Lai, S.-K., Liu, C.-C., Lei, H.-Y. An interferon-?-related cytokine storm in SARS patients. Journal of Medical Virology, (2004). 75(2), 185–194.
- [41]Bisno, A., Brito, M., & Collins, C. Molecular basis of group A streptococcal virulence. The Lancet Infectious Diseases, (2003). 3(4), 191–200.
- [42]Jahrling, P. B., Hensley, L. E., Martinez, M. J., LeDuc, J. W., Rubins, K. H., Relman, D. A., & Huggins, J. W. Exploring the potential of variola virus infection of cynomolgus macaques as a model for human smallpox. Proceedings of the National Academy of Sciences of the United States of America, (2004). 101(42), 15196–15200.
- [43]Hussell, T., & Goulding, J. Structured regulation of inflammation during respiratory viral infection. The Lancet Infectious Diseases, (2010). 10(5), 360–366. https://doi.org/10.1016/s1473-3099(10)70067-0
- [44]Peiris, J. S. M., Cheung, C. Y., Leung, C. Y. H., & Nicholls, J. M. Innate immune responses to influenza A H5N1: friend or foe? Trends in Immunology, (2009). 30(12), 574–584.
- [45]Martin, G. S., & Bernard, G. R. Airway and lung in sepsis. Intensive Care Medicine, (2001). 27(S1), S63–S79.
- [46]Uccelli, A., & de Rosbo, N. K. The immunomodulatory function of mesenchymal stem cells: mode of action and pathways. Annals of the New York Academy of Sciences, (2015).1351(1), 114–126.
- [47]Lee, J. W., Fang, X., Krasnodembskaya, A., Howard, J. P., & Matthay, M. A. Concise Review: Mesenchymal Stem Cells for Acute Lung Injury: Role of Paracrine Soluble Factors. Stem Cells, (2011). 29(6), 913–919.
- [48]Soares, M. O., Welton, N. J., Harrison, D. A., Peura, P., Hari, M. S., Harvey, S. E., Madan, J. J., Ades, A. E., Palmer, S. J., & Rowan, K. M. An evaluation of the feasibility, cost and value of information of a multicentre randomised controlled trial of intravenous immunoglobulin for sepsis (severe sepsis and septic shock): incorporating a systematic review, meta-analysis and value of information analysis. Health Technol Assess. 2012;16(7):1-186.
- [49]Rhodes, A., Evans, L. E., Alhazzani, W., Levy, M. M., Antonelli, M., Ferrer, R., Kumar, A., Sevransky, J. E., Sprung, C. L., Nunnally, M. E., Rochwerg, B., Rubenfeld, G. D., Angus, D. C., Annane, D., Beale, R. J., Bellinghan, G. J., Bernard, G. R., Chiche, J.-D., Coopersmith, C., & De Backer, D. P. Surviving Sepsis Campaign: International Guidelines for Management of Sepsis and Septic Shock: 2016. Intensive Care Medicine, (2017). 43(3), 304–377.
- [50]Cronin, L., Cook, D. J., Carlet, J., Heyland, D. K., King, D. B. M., Lansang, M. A. D., & Fisher, J. Corticosteroid treatment for sepsis. Critical Care Medicine, (1995). 23(8), 1430–1439.
- [51]Bollaert, P.-E., Charpentier, C., Levy, B., Debouverie, M., Audibert, G., Larcan, A. Reversal of late septic shock with supraphysiologic doses of hydrocortisone. Critical Care Medicine, (1998). 26(4), 645–650.
- [52]Briegel, J., Forst, H., Haller, M., Schelling, G., Kilger, E., Kuprat, G., Hemmer, B., Hummel, T., Lenhart, A., Heyduck, M., Stoll, C., & Peter, K. Stress doses of hydrocortisone reverse hyperdynamic septic shock. Critical Care Medicine, (1999). 27(4), 723–732.
- [53]Annane, D., Bellissant, E., Bollaert, P.-E., Briegel, J., Confalonieri, M., De Gaudio, R., Keh, D., Kupfer, Y., Oppert, M., & Meduri, G. U. Corticosteroids in the Treatment of Severe Sepsis and Septic Shock in Adults. JAMA, (2009). 301(22), 2362.
- [54]Meduri, G. U., Muthiah, M. P., Carratù, P., Eltorky, M., & Chrousos, G. P. Nuclear Factor-ĸB- and Glucocorticoid Receptor α- Mediated Mechanisms in the Regulation of Systemic and Pulmonary Inflammation during Sepsis and Acute Respiratory Distress Syndrome. Neuroimmunomodulation, (2005). 12(6), 321–338.
- [55]ohannes, T., Mik, E. G., Klingel, K., Dieterich, H.-J., Unertl, K. E., & Ince, C. Low-dose dexamethasone-supplemented fluid resuscitation reverses endotoxin-induced acute renal failure and prevents cortical microvascular hypoxia. Shock, (2009). 31(5), 521–528.
- [56]Rinaldi, S., Adembri, C., Grechi, S., & De Gaudio, A. R. Low-dose hydrocortisone during severe sepsis: Effects on microalbuminuria. Critical Care Medicine, (2006). 34(9), 2334–2339.
- [57]Vachharajani, V., Vital, S., Russell, J., Scott, L. K., Granger, D. N. Glucocorticoids inhibit the cerebral microvascular dysfunction associated with sepsis in obese mice. Microcirculation, (2006). 13(6), 477–487.
- [58]Hafezi-Moghadam, A., Simoncini, T., Yang, Z., Limbourg, F. P., Plumier, J.-C., Rebsamen, M. C., Hsieh, C.-M., Chui, D.-S., Thomas, K. L., Prorock, A. J., Laubach, V. E., Moskowitz, M. A., French, B. A., Ley, K., Liao, J. K. Acute cardiovascular protective effects of corticosteroids are mediated by non-transcriptional activation of endothelial nitric oxide synthase. Nature Medicine, (2002). 8(5), 473–479.
- [59]Marik, P. E., Pastores, S. M., Annane, D., Meduri, G. U., Sprung, C. L., Arlt, W., Keh, D., Briegel, J., Beishuizen, A., Dimopoulou, I., Tsagarakis, S., Singer, M., Chrousos, G. P., Zaloga, G., Bokhari, F., & Vogeser, M. Recommendations for the diagnosis and management of corticosteroid insufficiency in critically ill adult patients: Consensus statements from an international task force by the American College of Critical Care Medicine. Critical Care Medicine, (2008). 36(6), 1937–1949.
- [60]Zhang, M., Chen, H., Yang, Z., Zhang, M., Wang, X., Zhao, K., Li, X., Xiu, N., Tong, F., & Wang, Y. 17β-Estradiol Attenuates LPS-Induced Macrophage Inflammation In Vitro and Sepsis-Induced Vascular Inflammation In Vivo by Upregulating miR-29a-5p Expression. Mediators of Inflammation, 2021, 1–16.
- [61]Mathias, B., Szpila, B. E., Moore, F. A., Efron, P. A., & Moldawer, L. L. A Review of GM-CSF Therapy in Sepsis. Medicine, (2015). 94(50), e2044.
- [62]Bo, L., Wang, F., Zhu, J., Li, J., & Deng, X. Granulocyte-colony stimulating factor (G-CSF) and granulocyte-macrophage colony stimulating factor (GM-CSF) for sepsis: a meta-analysis. Critical Care, (2011). 15(1), R58.
- [63]Van den Berghe, G., Wouters, P., Weekers, F., Verwaest, C., Bruyninckx, F., Schetz, M., Vlasselaers, D., Ferdinande, P., Lauwers, P., & Bouillon, R. Intensive Insulin Therapy in Critically Ill Patients. New England Journal of Medicine, (2001). 345(19), 1359–1367. https://doi.org/10.1056/nejmoa011300
- [64]Hotchkiss, R. S., & Karl, I. E. The Pathophysiology and Treatment of Sepsis. New England Journal of Medicine, (2003). 348(2), 138–150.
- [65]Tang, R., Jia, L., Li, Y., Zheng, J., & Qi, P. Narciclasine attenuates sepsis-induced myocardial injury by modulating autophagy. Aging, (2021). 13(11), 15151–15163.
- [66]Ehrnthaller, C., Braumüller, S., Kellermann, S., Gebhard, F., Perl, M., & Huber-Lang, M. Complement Factor C5a Inhibits Apoptosis of Neutrophils—A Mechanism in Polytrauma? Journal of Clinical Medicine, (2021). 10(14), 3157.
- [67]Atzeni, F., & Sarzi-Puttini, P. Tumor Necrosis Factor. Brenner’s Encyclopedia of Genetics, (2013). 229–231.
- [68]Clark, I. A., Alleva, L. M., Mills, A. C., & Cowden, W. B. Pathogenesis of Malaria and Clinically Similar Conditions. Clinical Microbiology Reviews, (2004). 17(3), 509–539. https://doi.org/10.1128/CMR.17.3.509-539.2004
- [69]Tracey, K. J., Beutler, B., Lowry, S. F., Merryweather, J., Wolpe, S., Milsark, I. W., Hariri, R. J., Fahey, T. J., Zentella, A., Albert, J. D., Shires, G. T., & Cerami, A. Shock and Tissue Injury Induced by Recombinant Human Cachectin. Science, (1986). 234(4775), 470–474.
- [70]Tracey, K. J., Beutler, B., Lowry, S. F., Merryweather, J., Wolpe, S., Milsark, I. W., Hariri, R. J., Fahey, T. J., Zentella, A., Albert, J. D., Shires, G. T., & Cerami, A. Shock and Tissue Injury Induced by Recombinant Human Cachectin. Science, (1986). 234(4775), 470–474.
- [71]Qiu, P., Cui, X., Sun, J., Welsh, J., Natanson, C., & Eichacker, P. Q. Antitumor Necrosis Factor Therapy Is Associated With Improved Survival in Clinical Sepsis Trials. Critical Care Medicine, (2013). 41(10), 2419–2429.
- [72]halayasingam, N., & Isaacs, J. D. Anti-TNF therapy. Best Practice & Research Clinical Rheumatology, (2011). 25(4), 549–567.
- [73]Cohen, J., & Carlet, J. INTERSEPT. Critical Care Medicine, (1996). 24(9), 1431–1440.
- [74]Beutler, B., Milsark, I., & Cerami, A. Passive immunization against cachectin/tumor necrosis factor protects mice from lethal effect of endotoxin. Science, (1985).229(4716), 869–871.
- [75]Lv, S., Han, M., Yi, R., Kwon, S., Dai, C., & Wang, R. Anti-TNF-α therapy for patients with sepsis: a systematic meta-analysis. International Journal of Clinical Practice, (2014). 68(4), 520–528.
- [76]Rhen, T., & Cidlowski, J. A. Antiinflammatory action of glucocorticoids–new mechanisms for old drugs. The New England Journal of Medicine, (2005). 353(16), 1711–1723.
- [77]Annane, D. Effect of treatment with low doses of hydrocortisone and fludrocortisone on mortality in patients with septic shock. JAMA, (2002). 288(7), 862.
- [78]Sprung, C. L., Annane, D., Keh, D., Moreno, R., Singer, M., Freivogel, K., Weiss, Y. G., Benbenishty, J., Kalenka, A., Forst, H., Laterre, P.-F., Reinhart, K., Cuthbertson, B. H., Payen, D., & Briegel, J. Hydrocortisone Therapy for Patients with Septic Shock. New England Journal of Medicine, (2008). 358(2), 111–124.
- [79]Opal, S. M., Laterre, P.-F., Francois, B., LaRosa, S. P., Angus, D. C., Mira, J.-P., Wittebole, X., Dugernier, T., Perrotin, D., Tidswell, M., Jauregui, L., Krell, K., Pachl, J., Takahashi, T., Peckelsen, C., Cordasco, E., Chang, C.-S., Oeyen, S., Aikawa, N., & Maruyama, T. Effect of Eritoran, an Antagonist of MD2-TLR4, on Mortality in Patients With Severe Sepsis. JAMA, (2013). 309(11), 1154.
- [80]Fink, M. P. (2013). Animal models of sepsis. Virulence, 5(1), 143–153.
- [81]Schulert, G. S., & Cron, R. Q. The genetics of macrophage activation syndrome. Genes & Immunity, (2020). 21(3), 169–181.
- [82]Bhaskar, S., Sinha, A., Banach, M., Mittoo, S., Weissert, R., Kass, J. S., Rajagopal, S., Pai, A. R., & Kutty, S. Cytokine storm in COVID-19—immunopathological mechanisms, clinical considerations, and therapeutic approaches: the reprogram consortium position paper. Frontiers in Immunology, (2020).11.
- [83]Myers, A. K., Robey, J. W., & Price, R. M. Relationships between tumour necrosis factor, eicosanoids and platelet-activating factor as mediators of endotoxin-induced shock in mice. British Journal of Pharmacology, (1990). 99(3), 499–502.
- [84]Sha, T., Sunamoto, M., Kitazaki, T., Sato, J., Ii, M., & Iizawa, Y. Therapeutic effects of TAK-242, a novel selective Toll-like receptor 4 signal transduction inhibitor, in mouse endotoxin shock model. European Journal of Pharmacology, (2007). 571(2-3), 231–239.
- [85]Creasey, A. A., Chang, A. C., Feigen, L., Wün, T. C., Taylor, F. B., & Hinshaw, L. B. Tissue factor pathway inhibitor reduces mortality from Escherichia coli septic shock. Journal of Clinical Investigation, (1993). 91(6), 2850–2860.
- [86]Giral, M., Balsa, D., Ferrando, R., Merlos, M., Garcia-Rafanell, J., & Forn, J. Effects of UR-12633, a new antagonist of platelet-activating factor, in rodent models of endotoxic shock. British Journal of Pharmacology, (1996). 118(5), 1223–1231.
- [87]Opal, S. M., Palardy, BA, J. E., Parejo, BA, N. A., & Creasey, A. A. The activity of tissue factor pathway inhibitor in experimental models of superantigen-induced shock and polymicrobial intra-abdominal sepsis. Critical Care Medicine, (2001). 29(1), 13–17.
- [88]Alexander, H. R., Doherty, G. M., Buresh, C. M., Venzon, D. J., & Norton, J. A. A recombinant human receptor antagonist to interleukin 1 improves survival after lethal endotoxemia in mice. Journal of Experimental Medicine, (1991). 173(4), 1029–1032.
- [89]Hinshaw, L. B., Beller-Todd, B. K., Archer, L. T., Benjamin, B., Flournoy, D. J., Passey, R., & Wilson, M. F. Effectiveness of Steroid/Antibiotic Treatment in Primates Administered LD100 Escherichia coli. Annals of Surgery, (1981). 194(1), 51–56.
- [90]Van Zee, K. J., Moldawer, L. L., Oldenburg, H. S., Thompson, W. A., Stackpole, S. A., Montegut, W. J., Rogy, M. A., Meschter, C., Gallati, H., Schiller, C. D., Richter, W. F., Loetscher, H., Ashkenazi, A., Chamow, S. M., Wurm, F., Calvano, S. E., Lowry, S. F., & Lesslauer, W. Protection against lethal Escherichia coli bacteremia in baboons (Papio anubis) by pretreatment with a 55-kDa TNF receptor (CD120a)-Ig fusion protein, Ro 45-2081. Journal of Immunology (Baltimore, Md.: 1950), (1996). 156(6), 2221–2230.
- [91]Camerota, Anthony J., Creasey, Abla A., Patla, V., Larkin, Vera A., & Fink, Mitchell P. Delayed treatment with recombinant human tissue factor pathway inhibitor improves survival in rabbits with gram‐negative peritonitis. the journal of infectious diseases (1998)., 177(3), 668–676.
- [92]Redl, H., Schlag, G., Paul, E., Bahrami, S., Buurman, W. A., Strieter, R. M., Kunkel, S. L., Davies, J., & Foulkes, R. Endogenous modulators of TNF and IL-1 response are under partial control of TNF in baboon bacteremia. American Journal of Physiology-Regulatory, Integrative and Comparative Physiology, (1996). 271(5), R1193–R1198.
- [93]Dellinger, R. P., Levy, M. M., Rhodes, A., Annane, D., Gerlach, H., Opal, S. M., Sevransky, J. E., Sprung, C. L., Douglas, I. S., Jaeschke, R., Osborn, T. M., Nunnally, M. E., Townsend, S. R., Reinhart, K., Kleinpell, R. M., Angus, D. C., Deutschman, C. S., Machado, F. R., Rubenfeld, G. D., & Webb, S. Surviving Sepsis Campaign: International Guidelines for Management of Severe Sepsis and Septic Shock, 2012. Intensive Care Medicine, (2013). 39(2), 165–228.
- [94]Zhou, F., Peng, Z., Murugan, R., & Kellum, J. A. Blood Purification and Mortality in Sepsis. Critical Care Medicine, (2013). 41(9), 2209–2220.
- [95]Honore, P. M., Hoste, E., Molnár, Z., Jacobs, R., Joannes-Boyau, O., Malbrain, M. L. N. G., & Forni, L. G. Cytokine removal in human septic shock: Where are we and where are we going? Annals of Intensive Care, (2019). 9(1).
- [96]Angus, D. C., & van der Poll, T. Severe Sepsis and Septic Shock. New England Journal of Medicine (2013)., 369(9), 840–851.
- [97]Gruda, M. C., Ruggeberg, K.-G., O’Sullivan, P., Guliashvili, T., Scheirer, A. R., Golobish, T. D., Capponi, V. J., & Chan, P. P. Broad adsorption of sepsis-related PAMP and DAMP molecules, mycotoxins, and cytokines from whole blood using CytoSorb® sorbent porous polymer beads. PLOS ONE, (2018). 13(1), e0191676.
- [98]Kellum, J. A., Venkataraman, R., Powner, D., Elder, M., Hergenroeder, G., & Carter, M. Feasibility study of cytokine removal by hemoadsorption in brain-dead humans*. Critical Care Medicine, (2008). 36(1), 268–272.
- [99]Kogelmann, K., Jarczak, D., Scheller, M., & Drüner, M. Hemoadsorption by CytoSorb in septic patients: a case series. Critical Care, (2017). 21(1).
- [100]Friesecke, S., Träger, K., Schittek, G. A., Molnar, Z., Bach, F., Kogelmann, K., Bogdanski, R., Weyland, A., Nierhaus, A., Nestler, F., Olboeter, D., Tomescu, D., Jacob, D., Haake, H., Grigoryev, E., Nitsch, M., Baumann, A., Quintel, M., Schott, M., & Kielstein, J. T. International registry on the use of the CytoSorb® adsorber in ICU patients. Medizinische Klinik – Intensivmedizin Und Notfallmedizin, (2017). 114(8), 699–707.
- [101]Hawchar F, László I, Öveges N, Trásy D, Ondrik Z, Molnar Z. Extracorporeal cytokine adsorption in septic shock: A proof of concept randomized, controlled pilot study. Journal of Critical Care, 2019, 49: 172–178.