Evaluation of oxidative stress activity and the levels of homocysteine, vitamin B12, and DNA methylation among women with breast cancer
Abstract
Breast cancer (BC) is the most common malignant tumor in women and the leading cause of cancer deaths worldwide. This work was conducted to estimate the roles of oxidative stress, vitamin B12, homocysteine (HCY), and DNA methylation in BC disease progression. Sixty BC patients (age range 33–80 years) and 30 healthy controls were recruited for this study. Patients with BC were split to group 1 consisted of stage II BC women (low level), and group 2 consisted of patients in stages III and IV (high level). Malondialdehyde (MDA), glutathione peroxidase 3 (GPX3), HCY, and vitamin B12 levels in the study groups were measured. Also, the 5-methylcytosine (5mC) global DNA methylation levels were evaluated. The results showed a significant increase in HCY, and MDA in BC patients compared to healthy controls, with evident increases observed in those with advanced-stage BC (stages III and IV). They were accompanied by significantly reduced levels of 5mC, with a positive correlation between 5mC and the different stages of BC. Also, patients in advanced stages and those with a poor prognosis were exposed to low levels of vitamin B12 and GPX3 (except for the patients in stage IV, which showed increased GPX3 levels). The findings of this study suggest that the differences in global DNA methylation levels at the various phases may be used as a risk factor for developing BC, which indicates the involvement of GPX3 and HCY in BC progression.
INTRODUCTION
The most frequent malignant tumor and the primary reason for cancer-related deaths in women globally is breast cancer (BC). It is anticipated that around 2.26 million new cases of BC in women will be diagnosed globally by 2020, with roughly 685,000 women dying because of the disease [1]. However, the architecture of BC is extremely complicated, and transcriptional problems and gene dysregulation can manifest on many different levels. Breast cancer also appears to have genetic and epigenetic characteristics [2]. Oxidative stress is the result of absence the balance between reactive oxygen species (ROS) generation and clearance [3]. An increase in ROS production, a reduction in antioxidants that reduce ROS, or a combination of both might result in excess ROS. Lipid peroxidation is a process in which excess ROS assaults and damages membrane lipids containing polyunsaturated fatty acids or phospholipids with carbon-carbon double bonds [4].
Malondialdehyde (MDA) is a three-carbon dialdehyde that is highly reactive and is formed because of peroxidation of polyunsaturated fatty acids and arachidonic acid metabolism [5]. Due to its strong cytotoxicity, MDA is also regarded to be a tumor promoter and co-carcinogen [6]. The oxidative stress level can be assessed by measuring the production the levels of MDA. According to earlier research [7], MDA is employed as a marker in cancer. As a result, cells possess a balance system to neutralize too much ROS. This system is known as an antioxidant system, and it includes both enzyme-based antioxidants such as superoxide dismutase (SOD), catalase (CAT), and glutathione peroxidases (GPXs), as well as non-enzyme-based antioxidants that work together to reduce the oxidative state [8]. The primary mechanism defense against oxidative stress is GPX3, which is also essential in maintaining cellular redox homeostasis. By converting organic peroxides and hydrogen peroxide (H2O2) into water and the appropriate alcohols, GPX3 protects cells from oxidative stress damage through an enzymatic mechanism [9]. The toxic amino acid homocysteine (HCY), which contains sulfur and has no protein, is a byproduct of the interconversion of the two amino acids cysteine and methionine. There are two ways to metabolize homocysteine: re-methylation and trans-sulfuration [10]. Methionine is the source of HCY in the human body. Homocysteine is transformed into cysteine via the trans-sulfuration process when methionine levels are excessive. Homocysteine is re-methylated to methionine in the presence of a negative methionine balance; this reaction necessitates the cofactors methionine synthase and vitamin B12 [11].
Numerous reports suggest DNA methylation plays a master key in the development of tumor. A methyl donor, which is mainly produced by metabolism of one-carbon, is necessary for DNA methylation [12]. All methylation reactions that take place in living organisms are thought to use S-adenosylmethionine (SAM) as their primary methyl donor [13]. It can change DNA cytosine methylation and disrupt the methionine cycle by lowering intracellular SAM levels. One-carbon metabolism (OCM) is influenced by several substances, including homocysteine, methionine, folate (vitamin B9), vitamin B6, and vitamin B12. These components interact with one another in a different biochemical metabolic process. Via the intermediate impact of these molecules, one-carbon groups are transported to maintain DNA methylation, manage gene structure, and provide a basic material for different biological activities [14]. Additionally, OCM is an active operation in which reduce or increase of one component can disrupt DNA methylation and integrity of genomic. Therefore, by changing how epigenetic modifications are made, how tumor suppressor and oncogene genes are balanced, and how malignant transformation is triggered [15]. The frequent modification of DNA methylation in cancer is well known. In human cells, DNA methyl transferase enzymes (DNMTs) function primarily in the context of cytosine-guanine dinucleotides (CpG) to add a methyl group to the cytosine base at position 5 (5-methylcytosine; 5mC) [16].
This study was aimed at determining the correlation between oxidative stress, vitamin B12, homocysteine, and DNA methylation and BC risk to evaluate their role in BC disease progression.
MATERIALS AND METHODS
Study groups
Sixty breast cancer patients were randomly chosen from among the 90 Iraqi women who participated in the study, which was conducted at the Oncology Teaching Hospital, Medical City, and Baghdad, Iraq. The research was conducted between November 2020 and April 2021. The age of breast cancer patients ranges from 33–80 years. Additionally, 30 healthy women whose ages ranged from 32 to 75 years old were involved in this study. Patients with BC included in this study were divided into two groups: Group 1 was made up of stage II breast cancer patients (low level), whereas Group 2 was made up of stage III and IV patients (high level).
Collection of blood samples
The venous blood samples were drawn from positive BC patients at the time of diagnosis and five ml of disposable syringes were using in the sitting position. Each subject had a vein puncture to obtain 5 ml of blood. Three milliliters of blood were progressively forced into disposable serum tubes containing separating gel, while the remaining 2 ml were put into ethylene diamine tetraacetic acid (EDTA) tubes. The serum was then kept at -20˚C for subsequent use after the blood in the gel tubes had been allowed to coagulate at room temperature for 15 min. The blood in the EDTA tubes was subsequently used for DNA extraction and was kept at -20˚C until use.
Ethical clearance
The ethical committee of the department of biology at the College of Science at the University of Bagdad, Baghdad, Iraq, gave their stamp of approval to this work. The authorization with the reference number CSEC/1120/0082 was obtained on November 15, 2020.
Quantitative measurements of biomarkers, homocysteine, and vitamin B12 in serum samples
The quantitative measurements of biomarkers (MDA and GPX3), HCY, and vit. B12 in samples of human serum were performed using (ELISA) kit (Sun Long Biotech, China), following the manufacturer’s instructions. Before use, all reagents and samples were thawed and brought to room temperature. Then, 50 μl of standards (S1, S2, S3, S4, and S5) were added to wells of a 600 ml wash buffer, leaving one well empty to act as a blank control. The microtiter plate was mixed, covered, and kept there for half an hour at 37 °C. After 40 μl of sample dilution buffer and 10 μl of serum samples were added. The solution in all wells was discarded, and the washing solution was added to each well to wash it five times. After adding 50 μl of (Horseradish peroxidase) HRP-conjugate reagent to each well, mixing it, and covering the plate, the plate was incubated at 37°C for 30 minutes before being removed and washed five times as before. Following this, 50 μl of each chromogen solution was added to each well in the dark, gently mixed, and followed by 15 minutes of incubation at °C. The reaction was stopped by pouring the stop solution (50 μl) to each well, which caused the wells to become yellow. This caused the reaction to be complete. A spectrophotometric microplate reader set to a wavelength of 450 nm was used to determine the absorbance of the sample (ELISA reader, Mindray, India). The concentration was derived from the standard curve, which was used in the calculation.
DNA extraction
The DNA was extracted from the whole blood samples for both BC patients and control groups by using the gSYNC™ DNA Extraction Kit (Geneaid, Taiwan). The presence and purity of extracted DNA were confirmed using a Nanodrop spectrophotometer (Thermo, USA), which calculates DNA concentration (ng/μl) and examines DNA purity by measuring the absorbance at (260/280 nm). Staining DNA with ethidium bromide after running it on 1% agarose gels at 80 V for 30 minutes revealed any damage to the DNA.
Global DNA methylation procedure
Following the manufacturer’s instructions, the MethylFlashTM methylated DNA quantification Kit (Epigentek, USA) was used to measure the total 5-methylcytosine (5mC) content in DNA extracted from blood samples. For each sample, 100 ng of genomic DNA was used in the assay. To begin, a standard curve was created for a methylated polynucleotide serving as a positive control that contained 50% of 5mC. This curve was created by employing the five concentrations illustrated in Figure 1. Following the addition of the standard DNA and the sample DNA in their corresponding wells. Absorbance was measured using an ELISA reader at 450 nm. The standard curve as obtained by linear regression is shown in Figure 1, and the formula below was used to compute the proportion of 5mC in the entire DNA.
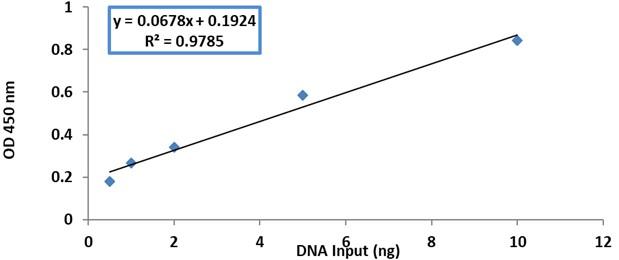
Statistical analysis
Statistical analysis was performed by using the statistical package for the social sciences (SPSS) version 23. The result was stated as Mean ± SEM. Statistical comparison between groups was analyzed using an analysis of variance (ANOVA), and a p≤0.05 was considered significant [17].
RESULTS
Oxidative stress biomarker levels in low and high stages of breast cancer
According to the statistical analysis, MDA levels were significantly (p≤0.001) higher in breast cancer patients than in the control group. As shown in Figure 2, the results of the current study showed a highly significant (p≤0.001) increased level of MDA in patients of BC with low stage compared to the control group. Furthermore, a highly significant (p≤0.001) increased level of MDA was observed in patients of BC with high stage compared to patients with a low stage and the control group. The levels of GPX3 in the control group and breast cancer patients did not differ significantly. A significant (p≤0.05) reduction was observed when comparing low-stage breast cancer patients to high-stage. Also, the results showed significantly (p≤0.05) reduction in the level of GPX3 in low-stage breast cancer patients comparing to control groups. Meanwhile, there was no significant difference (p≤0.05) in the GPX3 level in breast cancer patients at the high-level stage and control group (Figure 3).
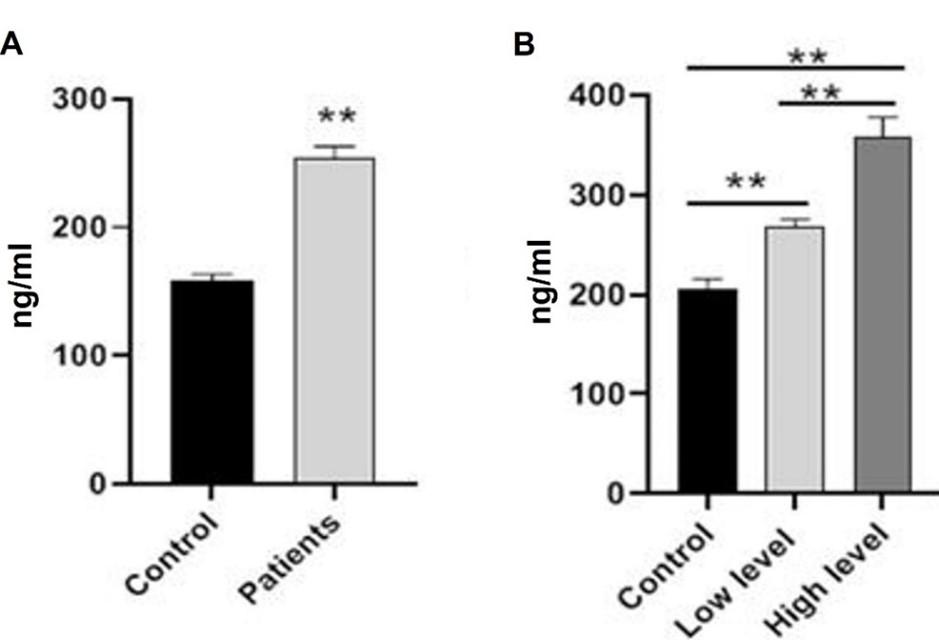
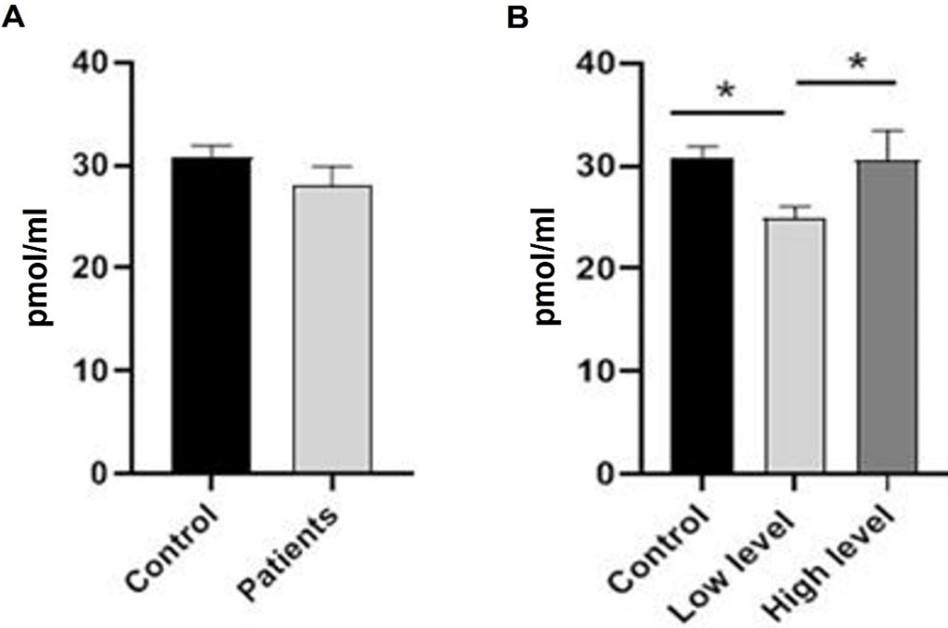
Serum vitamin B12 and homocysteine levels in breast cancer patients
Homocysteine levels of BC patients significantly (p≤0.001) increased when compared to the control group. As shown in Figure 4, there was also evidence of a highly significant rise (p≤0.001) in patients at the high-level stage compared to patients at the low-level stage and control groups, as well as a significant increase (p≤0.05) in the level of HCY in patients at the low-level stage compared to control. Patients with breast cancer had significantly (p≤0.001) lower vitamin B12 levels compared to the control group. In addition, the number of patients who were in the high-level stage dropped significantly (p≤0.001) when compared to the low-level stage and the control group. Figure 5 demonstrates that in comparison to the control group, vitamin B12 levels in patients who were at the low-level stage dropped by a statistically significant amount (p≤0.001).
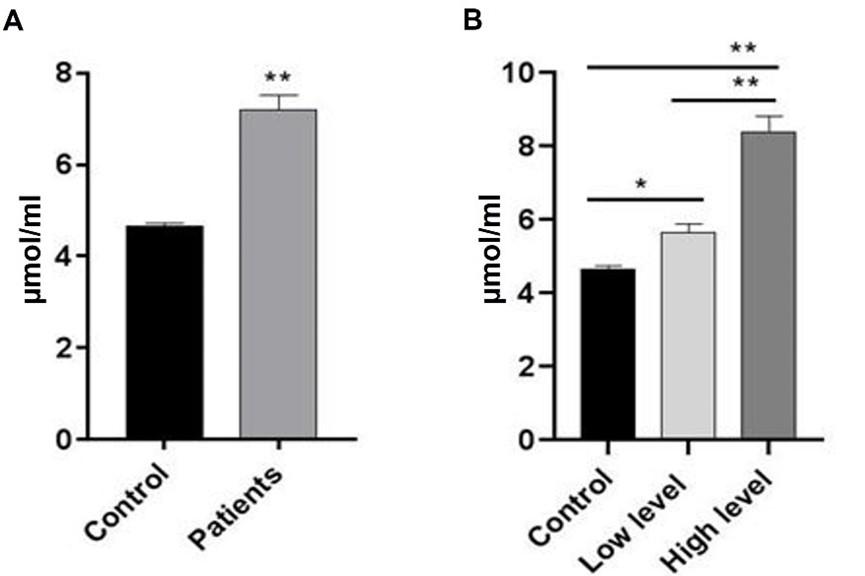
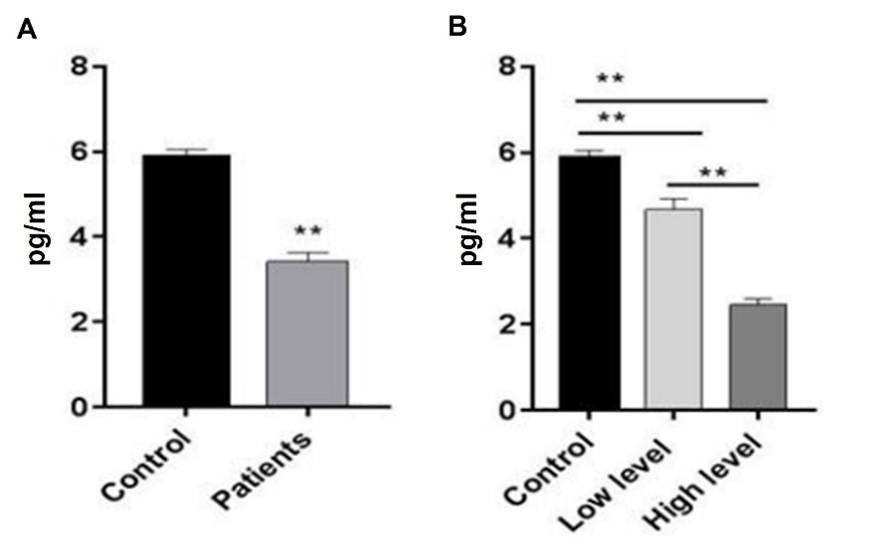
Comparison of serum biomarkers in different stages of breast cancer
Blood MDA levels were significantly (p≤0.001) higher in stage IV breast cancer patients compared to stage II and III patients. Serum MDA levels were significantly (p≤0.001) higher in stage III breast cancer patients compared to stage II patients. Glutathione peroxidase was found to be significantly (p≤0.001) higher in stage IV patients compared to stage II and III patients, but not significantly (p>0.05) different between patients in stages II and III. HCY levels were significantly (p≤0.001) higher in stage IV breast cancer patients than in stage II and III patients. In addition, there was an increase that might be considered statistically significant (p≤0.001) between phases II and III. The levels of vitamin B12 in individuals with stage IV breast cancer were shown to be statistically substantially (p≤0.001) lower when compared to those with stages II and III of the disease. According to Table 1, there were no statistically significant differences (p>0.05) between individuals diagnosed with breast cancer at stages II and III.
Table 1. Serum levels of MDA, GPX3, HCY, and vitamin B12 in stages of breast cancer groups.
Global changes in 5-methylcytosine contents in breast cancer patients
Compared to the control group, there was a highly significant (p≤0.001) reduction in 5mC levels in breast cancer patients. The patients with low levels of the disease showed a slightly significant (p≤0.05) decrease in 5mC levels compared to the control group. In addition, Figure 6 displays a highly significant (p≤0.001) lower level of 5mC in breast cancer patients at the high-level stage compared to those at the low-level stage and control.
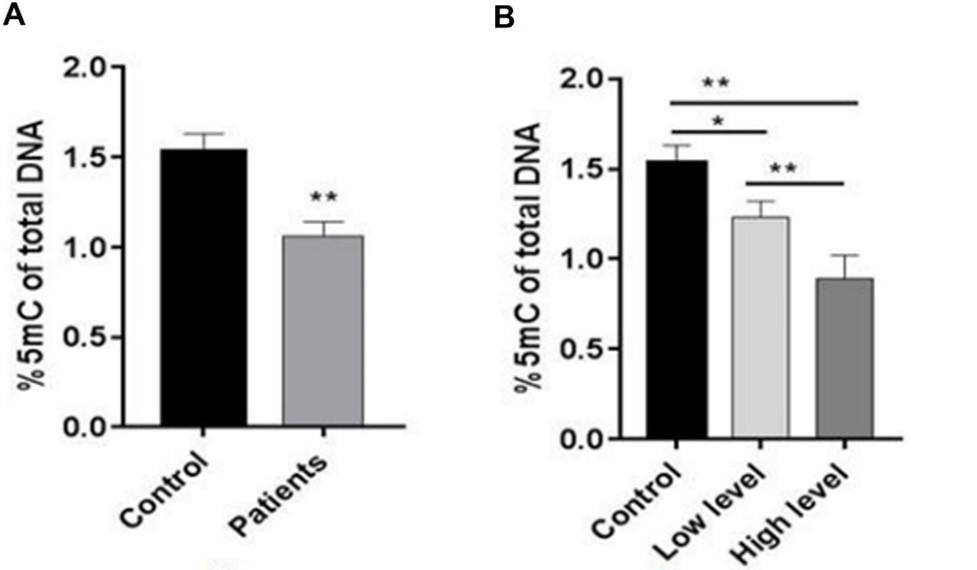
Comparison of global 5-methylcytosine in different stages of breast cancer patients
The level of 5mC decreased significantly (p≤0.01) in stage IV (No12) breast cancer patients compared to stage II patients (No.30). However, no significant differences (p>0.05) between stage IV and III (No.18) breast cancer patients were observed. Also, there was a significant (p≤0.01) decrease in the 5mC level in stage III patients compared to stage II patients (Table 2).
Table 2. Comparison of global 5-methylcytosine (5mC) in different stages of breast cancer.
DISCUSSION
Breast cancer is one of the most public diseases among middle-aged and older women worldwide. Due to the increasing number the patients of breast cancer and their generally young age, breast cancer therapy and prognosis are receiving increased attention. Appropriate biomarkers aid in the prediction of prognosis and the selection of the best treatment for each patient [18, 19]. The results also indicated there was a highly significant rise in the level of MDA in patients with breast cancer in the high-level stage compared to patients in the low-level stage and control group. Also, a significantly higher increase in the level of MDA in patients with breast cancer in the low-level stage compared to control group was observed. This observation agrees with the findings of Baskaran et al. [20], who noted a highly significant degree of lipid peroxidation in breast cancer patients compared to controls. The results additionally demonstrated that patients in advanced stages of the disease and those with a bad prognosis were subjected to high levels of oxidative stress. The majority of ROS are produced by tumor cells, and surgical excision of malignant cells has been shown to reduce excessive MDA levels [21].
Additionally, a correlation between tumor growth and MDA serum levels, and rising MDA levels in the blood may signal the advancement of a tumor as it is shown by recent a study [22]. The results of the present study on the GPX3 status in cancer patients revealed that both patients with stage II and stage III breast cancer had somewhat lower levels of GPX3. In contrast to those with stage II and stage III breast cancer, an unexpectedly increased GPX3 in patients with stage IV breast cancer during the same period was observed. Glutathione peroxidases are at the forefront of cellular redox balance maintenance and oxidative stress defense [23]. Diverse antioxidant defense mechanisms are essential for regulating the formation of free radicals. By disrupting the pro-oxidant/antioxidant equilibrium, increased free radical production, inactivation of antioxidant enzymes, or excessive antioxidant intake all lead to oxidative damage [24]. Therefore, the lower levels of GPX3 in stages II and III may be explained by increased utilization to scavenge lipid peroxides and sequestration by tumor cells [25]. On the other hand, women with late-stage breast cancer had a dramatic elevation of GPX3 (stage IV). These results support previous research that demonstrated higher GPX3 expression is linked to ovarian cancer, is more common in tumors that are farther along in their development and is linked to poor patient survival [26]. The outcomes of this study are in line with Jardim et al. that showed the elevated GPX3 expression in breast cancer was linked with shorter overall survival time breast cancer patients as well as poor response to certain treatment [27]. Enhanced antioxidant enzyme activity is an adaptive response to free radical (ROS)-induced cell damage. Additionally, elevated ROS levels may activate signaling pathways that ultimately result in the production of GPX3 mRNA. The overexpression of GPX3 may detoxify hydrogen peroxides, toxins, and carcinogens [28]. It is observed that between early-stage (I/II) and late-stage (III/IV)/recurrent individuals, there was a significant difference in GPX3 levels [29], indicating that GPX3 could be used as a biomarker of disease progression.
During the breakdown of ingested proteins, HCY, a mediate sulfur-containing amino acid, is created from methionine. The levels of Plasma HCY are influenced by dietary nutrients such as vit. B12, B6 and folic acid, and others [30]. Akilzhanova et al. similarly reported an increase in HCY levels in breast cancer patients in their study. According to [31], who established a correlation between high plasma HCY levels and an increased risk of breast cancer, high plasma HCY levels may be a metabolic breast cancer risk factor. As a cofactor for various enzymes involved in the metabolism of HCY, vitamin B12 is necessary for the conversion of HCY to methionine [32]. Therefore, HCY breakdown would be hindered by vitamin B12 shortage, as shown in this study, and lower activity of the implicated metabolic enzymes, resulting in a buildup of intracellular HCY, rapid excretion into the circulation, and finally a rise in its level [33]. It has been proven that breast carcinogenesis is promoted by vitamin B12 deficiency, which increases HCY synthesis. However, the molecular signaling pathways underlying HCY-mediated mammary cancer remain unclear.
Vitamin B12 deficiency induced HCY-mediated tumor growth in Mouse mammary tumor virus (MMTV-ErbB2) transgenic mice by activating ErbB2/MAPK/PI3K/Akt signaling pathway. S-adenosylmethionine (SAM) activity for DNA methylation and gene regulation can be negatively impacted by vitamin B12 deficiency, which in turn increases the risk of breast cancer [34]. Additionally, it was discovered in this study that patients with late-stage breast cancer had significantly higher HCY levels than healthy individuals. These results are in line with other researchers who discovered considerably higher HCY in individuals whose breast cancer had spread through metastasis in advanced stages [35]. Homocysteine oxidative damage to cells, pro-oxidant activity, and an increase in free radicals and hydrogen peroxide are potential associations between elevated HCY levels and cancer [36].
Furthermore, earlier research has shown that HCY produces S-adenosylhomocysteine intracellular accumulation and promotes estrogen oxidative metabolites (catechol estrogens) [37]. A lot of anomalies in DNA methylation in cancer are responsible for altered gene expression, including the inactivation of tumor suppressor genes by hypermethylation and the triggering of pro-metastatic genes by hypomethylation [38]. Tumor cells exhibit two types of abnormal DNA methylation: both hypermethylation of CpG islands in promoter regions and hypomethylation of DNA throughout the whole of the cell are seen. Repetitive sequences show global DNA hypomethylation in cancer cells, but specific CpG islands near the promoters of tumor suppressor genes have been shown to have hypermethylation in cancer cells [39]. The extent of DNA hypomethylation across the entire genome is estimated by the sum of 5-mC in dinucleotide CpG sites. Most 5-mC sites are found in repetitive DNA sequences, which account for more than 50% of the human genome. In healthy tissue, these repetitive DNA sequences are heavily methylated; studies have shown that cancer cells exhibit global DNA hypomethylation in repetitive sequences while exhibiting hypermethylation at specific CpG islands in the promoters of tumor suppressor genes [40]. By counting the total number of 5-mC in dinucleotide CpG sites, the amount of genome-wide DNA hypomethylation can be calculated.
Over 50% of the human genome is made up of repetitive DNA sequences, which also make up most 5-mC sites and are heavily methylated in healthy tissue. In contrast, hypomethylation of these sequences significantly contributes to global DNA hypomethylation in cancer [40]. Hypomethylation in repetitive elements can cause genomic instability and reactivation of transposable element expression, whereas hypomethylation in gene bodies can disrupt alternative splicing [41]. Patients with stage IV breast cancer showed a significant reduction in the level of 5mC. This result supported the findings of Pasha et al., who discovered that methylation levels were significantly lower in advanced tumor stages in breast cancer patients than in stages I and II [42]. It was found in 75% of individuals with benign conditions and 96% of those with breast cancer, indicating that it might be utilized to detect tumorigenesis even before a physical breast examination. By activating the production of pro-metastatic genes including heparanase, MMP2 (encoding matrix metalloproteinase 2), and uPA (encoding urokinase plasminogen activator), DNA demethylation plays a critical role in cancer [43]. Demethylation may play a causal role in cancer metastasis since demethylating drugs increase the invasiveness of non-metastatic breast cancer cells [44]. DNA methylation is a reversible process, it has been demonstrated that reversing the un-methylation of breast cancer and liver cancer cell lines decreases the capacity of these cells to invade or metastasize [45].
CONCLUSION
The findings of the study reveal that there is a significant increase in MDA in patients of breast cancer compared to healthy subjects, with clear improvements shown in those with advanced-stage breast cancer. They were accompanied by significantly reduced levels in GPX3 levels (except for the patients in stage IV). Furthermore, a significantly increase in the HCY levels of breast cancer patients in advanced stages as compared to healthy subjects. Additionally, the patients in advanced stages of breast cancer and those with a poor prognosis experienced low levels of vitamin B12 and 5mC. These findings suggest that involvement of GPX3 and HCY in progression of BC, with the possibility of using variations in global DNA methylation levels at different stages as a risk factor for BC.
ACKNOWLEDGMENT
The authors are highly appreciative of all the patients for their great cooperation in collecting samples; they were an important part of this research. Also, the authors wish to acknowledge the staff of the Oncology Teaching Hospital, Medical City, Baghdad, Iraq, for their help in collecting the samples.
AUTHOR CONTRIBUTIONS
The experiments were designed and supervised by Rakad Mohammed Khamas (RMKh). RMKh and Rana Hanan Khudhair (RHK) performed the experiments. In addition to providing research assistance for data collection, RMKh and RHK helped with sample collection, documenting raw data, and collecting data. RMKh and RHK analyzed the data and interpreted the results. RMKh and RHK drafted and edited the manuscript. All the authors acknowledged that they shared responsibility for the work’s correctness and integrity.
CONFLICTS OF INTEREST
There is no conflict of interest among the authors.
References
- [1]Winter P, Kotowicz B, Fuksiewicz M, Kowalska M, Jagiello-Gruszfeld A, Nowecki Z. Predictive value of serum aurora a, thymidine kinase 1, and HER3/ErbB3 in breast cancer patients before neoadjuvant treatment. Research Square .2022; 12 January (V1).
- [2]Dai X, Cheng H, Bai Z, Li J. Breast cancer cell line classification and its relevance with breast tumor subtyping. Journal of Cancer. 2017; 8(16): 3131.
- [3]Marrocco I, Altieri F, Peluso I. Measurement and clinical significance of biomarkers of oxidative stress in humans. Oxidative medicine and cellular longevity. 2017.
- [4]Papac-Milicevic N, Busch CL, Binder CJ. Malondialdehyde epitopes as targets of immunity and the implications for atherosclerosis. Advances in Immunology. 2016; 131: 1-59.
- [5]Jadoon S, Malik A. A review article on the formation, mechanism and biochemistry of MDA and MDA as a biomarker of oxidative stress. International Journal of Advanced Research.2017; 5: 811-818.
- [6]Salzman R, Pácal L, Tomandl J, Kaňková K, Tóthová E, Gál B, Salzman P. Elevated malondialdehyde correlates with the extent of primary tumor and predicts poor prognosis of oropharyngeal cancer. Anticancer Research. 2009; 29(10): 4227-4231.
- [7]Cherian DA, Peter T, Narayanan A, Madhavan SS, Achammada S, Vynat GP. Malondialdehyde as a marker of oxidative stress in periodontitis patients. Journal of pharmacy and bioallied sciences. 2019; 11(Suppl 2): S297.
- [8]He L, He T, Farrar S, Ji L, Liu T, Ma X. Antioxidants maintain cellular redox homeostasis by elimination of reactive oxygen species. Cellular Physiology and Biochemistry. 2017; 44(2): 532-553.
- [9]Behnisch-Cornwell S, Wolff L, Bednarski PJ. The Effect of Glutathione Peroxidase-1 Knockout on Anticancer Drug Sensitivities and Reactive Oxygen Species in Haploid HAP-1 Cells. Antioxidants. 2020; 9(12): 1300.
- [10]Hasan T, Arora R, Bansal AK, Bhattacharya R, Sharma GS, Singh LR. Disturbed homocysteine metabolism is associated with cancer. Experimental and molecular medicine. 2019;51(2): 1-13.
- [11]Lin J, Lee IM, Song Y, Cook NR, Selhub J, Manson JE, Zhang SM. Plasma homocysteine and cysteine and risk of breast cancer in women. Cancer research. 2010 ;70(6): 2397-2405.
- [12]Newman AC, Maddocks OD. One-carbon metabolism in cancer. British journal of cancer2017;116(12): 1499-1504.
- [13]Ouyang Y, Wu Q, Li J, Sun S, Sun S. S‐adenosylmethionine: a metabolite critical to the regulation of autophagy. Cell proliferation. 2020; 53(11): e12891.
- [14]Shuvalov O, Petukhov A, Daks A, Fedorova O, Vasileva E, Barlev NA. One-carbon metabolism and nucleotide biosynthesis as attractive targets for anticancer therapy. Oncotarget. 2017; 8(14): 23955.
- [15]Yang J, Li H, Deng H, Wang Z. Association of one-carbon metabolism-related vitamins (folate, B6, B12), homocysteine and methionine with the risk of lung cancer: systematic review and meta-analysis. Frontiers in oncology. 2018;8: 493.
- [16]Skvortsova K, Stirzaker C, Taberlay P. The DNA methylation landscape in cancer. Essays in biochemistry. 2019; 63(6): 797-811.
- [17]Duncan RC, Knapp RG, Miller MC. Introductory Biostatics of health Science. John Wileyad Sons, London. 1983.
- [18]Kim MS, Kim SY, Kim JH, Park B, Choi HG. Depression in breast cancer patients who have undergone mastectomy: a national cohort study. PloS one. 2017;12(4):e0175395.
- [19]Hong W, Dong E. The past, present and future of breast cancer research in China. Cancer letters. 2014;351(1): 1-5.
- [20]Baskaran D, Singh AK, Prabasheela B. Antioxidant and lipid peroxidation status in pre- and post-operative breast carcinoma. International Journal of Pharma and Bio Sciences. 2011; 2 (1): 450-454.
- [21]Salzman R, Pácal L, Tomandl J, Kaňková K, Tóthová E, Gál B, Salzman P. Elevated malondialdehyde correlates with the extent of primary tumor and predicts poor prognosis of oropharyngeal cancer. Anticancer research. 2009;29(10): 4227-4231.
- [22]Lepara Z, Lepara O, Fajkić A, Rebić D, Alić J, Spahović H. Serum malondialdehyde (MDA) level as a potential biomarker of cancer progression for patients with bladder cancer. Romanian Journal of Internal Medicine. 2020; 58(3): 146-152.
- [23]Behnisch-Cornwell S, Wolff L, Bednarski PJ. The Effect of glutathione peroxidase-1 knockout on anticancer drug sensitivities and reactive oxygen species in haploid HAP-1 cells. Antioxidants. 2020; 9(12):1300.
- [24]Gupta RK, Patel AK, Kumari R, Chugh S, Shrivastav C, Mehra S, Sharma A N. Interactions between oxidative stress, lipid profile and antioxidants in breast cancer: a case control study. Asian Pacific Journal of Cancer Prevention. 2012;13(12):6295-6298.
- [25]Sarıkaya E, Doğan S. Glutathione Peroxidase in Health and Diseases. IntechOpen book, biochemistry, London, UK. 2020; 17: 49.
- [26]Worley BL, Kim YS, Mardini J, Zaman R, Leon KE, Vallur PG. Hempel N. GPX3 supports ovarian cancer progression by manipulating the extracellular redox environment. Redox biology. 2019; 25; 101051.
- [27]Jardim BV, Moschetta MG, Leonel C, Gelaleti GB, Regiani VR, Ferreira LC, de Campos Zuccari DA. Glutathione and glutathione peroxidase expression in breast cancer: an immunohistochemical and molecular study. Oncology reports. 2013; 30(3): 1119-1128.
- [28]Wang C, Yu J, Wang H, Zhang J, Wu N. Lipid peroxidation and altered antioxidant status in breast adenocarcinoma patients. Drug research. 2014; 64(12): 690-692.
- [29]Agnani D, Camacho-Vanegas O, Camacho C, Lele S, Odunsi K, Cohen S, Martignetti JA. Decreased levels of serum glutathione peroxidase 3 are associated with papillary serous ovarian cancer and disease progression. Journal of ovarian research. 2011; 4(1): 1-8.
- [30]Plazar N, Jurdana M. Hyperhomocysteinemia and the role of B vitamins in cancer. Radiology and oncology. 2010; 44(2): 79-85.
- [31]Akilzhanova A, Nurkina Z, Momynaliev K, Ramanculov E, Zhumadilov Z, Rakhypbekov T, Takamura N. Genetic profile and determinants of homocysteine levels in Kazakhstan patients with breast cancer. Anticancer research. 2013; 33(9): 4049-4059.
- [32]Froese DS, Fowler B, Baumgartner MR. Vitamin B12, folate, and the methionine remethylation cycle—biochemistry, pathways, and regulation. Journal of inherited metabolic disease. 2019 ;42(4): 673-685.
- [33]Xiao Z, Yao G, Liu Y, Zhao C. A Folate-and vitamin B12-deficient diet decreases the latency and increases the incidence of mammary tumors in MMTV-ErbB2 transgenic mice. Research Square.2021. 23April (V1).
- [34]Kim JA, Choi R, Won H, Kim S, Choi HJ, Ryu JM, Lee SY. Serum vitamin levels and their relationships with other biomarkers in Korean breast cancer patients. Nutrients. 2020;12(9): 2831.
- [35]Gatt A, Makris A, Cladd H, Burcombe RJ, Smith JM, Cooper P, Makris M. Hyperhomocysteinemia in women with advanced breast cancer. International Journal of Laboratory Hematology. 2007; 29(6): 421-425.
- [36]Chiang FF, Wang HM , Lan YC, Yang MH, Huang SC, Huang YC. High homocysteine is associated with increased risk of colorectal cancer independently of oxidative stress and antioxidant capacities.Clinical nutrition. 2014;33(6):1054-1060.
- [37]Naushad SM, Reddy CA, Kumaraswami K, Divyya S, Kotamraju S, Gottumukkala SR, Kutala VK. Impact of hyperhomocysteinemia on breast cancer initiation and progression: epigenetic perspective. Cell biochemistry and biophysics. 2014;68(2): 397-406.
- [38]Mahmood N, Arakelian A, Cheishvili D, Szyf M, Rabbani SA. S‐adenosylmethionine in combination with decitabine shows enhanced anti‐cancer effects in repressing breast cancer growth and metastasis. Journal of cellular and molecular medicine. 2020;24(18): 10322-10337.
- [39]Kerachian MA, Kerachian M. Long interspersed nucleotide element-1 (LINE-1) methylation in colorectal cancer. Clinica Chimica Acta. 2019; 488: 209-214.
- [40]Ehrlich M. DNA hypomethylation in cancer cells. Epigenomics. 2009; 1(2):239-259.
- [41]Chi HC, Tsai CY, Tsai MM, Lin KH. Impact of DNA and RNA methylation on radiobiology and cancer progression. International journal of molecular sciences. 2018; 19(2): 555.
- [42]Pasha HA, Rezk NA, Riad MA. Circulating cell free nuclear DNA, mitochondrial DNA and global DNA methylation: potential noninvasive biomarkers for breast cancer diagnosis. Cancer Investigation. 2019; 37(9): 432-439.
- [43]Ezeani MC, Onyenekwe CC, Meludu SC, Chianakwana GU, Anyiam DC, Emegoakor CD, Amilo GI. Assessment of DNA methylation patterns in breast tumours and apparently healthy subjects in Anambra state, Nigeria. Integrative Cancer Science and Therapeutics. 2017;4(3): 1-6.
- [44]Chik F, Szyf M. Effects of specific DNMT gene depletion on cancer cell transformation and breast cancer cell invasion; toward selective DNMT inhibitors. Carcinogenesis. 2011;32(2): 224-232.
- [45]Stefanska B, Huang J, Bhattacharyya B, Suderman M, Hallett M, Han ZG, Szyf M. Definition of the landscape of promoter DNA hypomethylation in liver cancer. Cancer research. 2011; 71(17): 5891-5903.