Investigation of PDGF-BB aptamer binding using growth factor-coated particles and flow cytometry
Abstract
Platelet-derived growth factor-BB (PDGF-BB), a multifunctional growth factor secreted by platelets, endothelial and cancer cells, functions as an efficient and accurate biomarker. PDGF- BB has been employed in various therapeutic applications. Tuning its bioactivity via a specific binding ligand could enhance the PDGF-BB functionality. Aptamer, a single-stranded oligonucleotide that exhibits a selective binding to PDGF-BB, is the molecule of our interest. This research aims to evaluate the PDGF-BB aptamer binding by growth factor-coated particles. PDGF-BB-coated particles were confirmed by enzyme-linked immunosorbent assay (ELISA), and aptamer binding evaluation was investigated by flow cytometry. To optimize the condition for binding evaluation, the washing cycle and concentration of PDGF-BB, polystyrene particle (PS) and aptamer were studied using flow cytometer. Based on the flow cytometry results, the concentration of PDGF-BB showed an optimal level on the number of fluorescent particles. The percentage of fluorescent particles increased when the washing cycle was reduced, and the particle concentration was decreased. The percentage of fluorescent particles also increased proportionally with the aptamer concentration. Using a flow cytometer to detect fluorescent signal from growth factor-coated particle is a promising strategy to study biomolecular binding.
INTRODUCTION
Angiogenesis, the formation of new blood vessels, plays an important role in many normal and pathological conditions such as during the growth and healing of tissues [1]. However, there are certain conditions where angiogenesis is unbalanced, and causes abnormal blood vessel growth. This unbalanced process leads to diseases and disorders such as solid and hematological tumors, atherosclerosis, rheumatoid arthritis and psoriasis [2]. One of the growth factors that contributes to the progression of angiogenesis is the platelet-derived growth factor (PDGF) [3]. Major sources of PDGF are platelets, endothelial and cancer cells. This growth factor regulates cell division, proliferation, and survival by interacting with receptor tyrosine kinase called platelet-derived growth factor receptor (PDGFR) [4]. In abnormal conditions, PDGF promotes tumor growth by autocrine stimulation of malignant cells, through overexpression or overactivation of PDGFR [5, 6]. Therefore, altering the bioactivity of PDGF using a specific binding ligand could be a strategy for treating cancer as well as other related disorders. Platelet-derived growth factor BB (PDGF-BB) is a member of PDGF. It exhibits a mitogenic activity that promotes cell division in a variety of cell types via its receptor, PDGFR-b. Both PDGF-BB and PDGFR-β are part of a ligand-tyrosine kinase receptor system that plays a significant role in angiogenesis [7, 8].
The specific ligands that recently have drawn much attention are aptamers. Aptamers are single-stranded oligonucleotides that bind tightly and specifically to their targets; small molecules, biomacromolecules, viruses, and cells [9]. They are used as a recognition element in several systems [9]. Aptamer sequences are screened by a strategy named systematic evolution of ligands by exponential enrichment (SELEX) [10]. Aptamers carry many beneficial properties including high affinity, ease of modification, stable molecule, low immunogenicity, and target variety [11]. Furthermore, aptamers are used for several biological and biomedical applications such as cell labeling, cell separation, bio-related materials, and drug delivery systems [12-14].
To effectively interrupt the binding interaction between PDGF-BB and its corresponding receptor, a platform for evaluating the specific binding ligands needs to be developed. In this regard, an aptamer that binds to PDGF-BB was chosen as a model molecule since it has been studied extensively. PDGF-BB aptamer shows promise in a variety of applications including biosensors and diagnostic tools and therapeutic agents [15, 16]. Recently, PDGF-BB aptamer has been developed to find applications in cancers such as breast [17], colon [18], and prostrate [19] cancer. In addition, the interaction of PDGF-BB aptamer has been characterized by computational simulation [20, 21], immunoassay [22], microcantiver [23], and radiolabeled assay [20]. This research focused on developing PDGF-BB-coated particles for use in the evaluation of PDGF-BB aptamer binding. The binding was characterized by flow cytometry. The overall concept is presented in Figure 1. The effect of aptamer concentration, growth factor concentration, particle concentration, and washing step on binding signal were investigated.
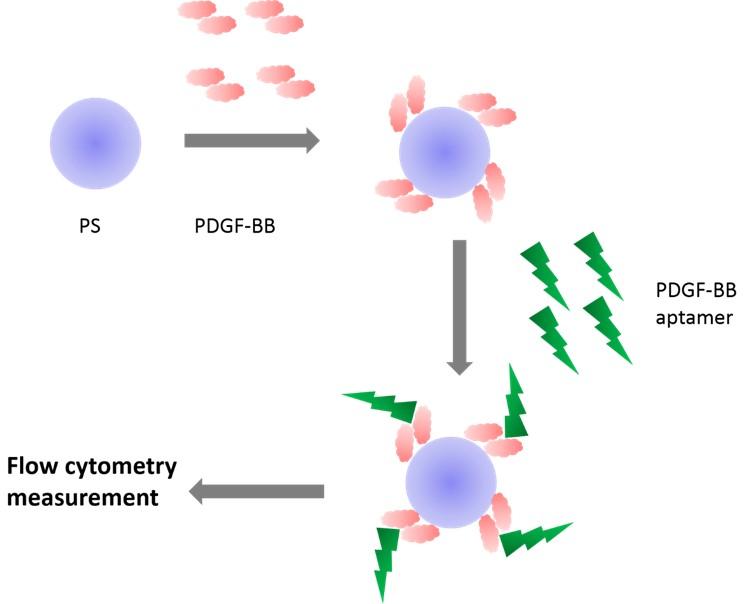
MATERIALS AND METHODS
Materials
Fluorescence-labeled PDGF-BB aptamers listed in Table 1 were purchased from Integrated DNA Technologies (Asia Pacific Production, Singapore). PS with an average diameter of 1.04 mm were purchased from Spherotech (Lake Forest, Illinois). ELISA development kit for human platelet-derived growth factor subunit B (PDGF-BB) was purchased from R&D Systems (Minneapolis, Minnesota). Phosphate buffered saline (PBS) was purchased from Sigma-Aldrich (Saint Louis, Missouri). Tween 20 was purchased from Bio-Rad laboratories (Singapore). Fetal bovine serum (FBS) collected in South America was purchased from Capricorn Scientific (Ebsdorfergrund, Germany).
Table 1. The sequence of fluorescence-labeled aptamers.
Characterization of polystyrene particles by flow cytometry
To minimize the amount of PS used in flow cytometry, the particles were serially diluted by PBS ranging from 0.0078-0.25 mg/mL. Then the obtained particle suspensions were characterized by a flow cytometer (DxFLEX, Beckman Coulter). Signals from 10,000 counts were recorded.
Feasibility of PDGF-BB coating
PS (0.0050 mg/mL) was incubated with 1,000 pg/ml PDGF-BB at room temperature for 30 minutes. After incubation, the mixture was centrifuged at 5,000 rpm for 5 minutes. PDGF-BB remained in supernatant was quantified by ELISA following the manufacturing’s protocol. In brief, 100 µL of capture antibodies with the concentration of 400 ng/mL were incubated in each well of ELISA strips for 2 hours. The well surfaces were further blocked by 300 µL of 0.05% w/v BSA for 2 hours. The wells were washed with 300 µL of PBS containing 0.05% Tween 20 for 3 times. Then, 100 µL of the solutions containing PDGF-BB standard, freshly prepared PDGF-BB, and the supernatant were transferred into the ELISA strips and then incubated for 2 hours. The strips were washed 3 times. After that, 100 µL of detection antibodies (400 ng/mL) were added, incubated for 2 hours, and then discarded. Thereafter, 100 µL of streptavidin-horseradish peroxidase (HRP) were added to the wells, incubated for 30 minutes, and then discarded. Consequently, 100 µL of the substrate was added and incubated for 30 minutes. Finally, 50 µL of stop solution was added. Subsequently, the optical density (OD) of the solutions was measured by the plate reader (Chameleon, Hidex). The λmax was set at 472 nm. The experiments were done in triplicate.
Preparation of PDGF-BB-coated particles
PS with the concentrations of 0.0078, 0.0039, and 0.0019 mg/mL were incubated with PDGF-BB at designated concentrations for 30 minutes. Next, the surface of polystyrene particles was blocked by incubating the particles with 0.075% w/v FBS for 60 minutes. To obtain PDGF-BB-coated particles, the mixture was then centrifuged to separate, and the particles were washed with PBS twice.
Investigation of PDGF-BB aptamer binding
PDGF-BB-coated particles with the designated concentrations were incubated with 50, 100, and 200 nM fluorescence-labeled PDGF-BB aptamer (T38) for 30 minutes. The suspensions were centrifuged to separate the particles from the buffer twice. Finally, the fluorescent signal from the particle was determined using the flow cytometer. The detected fluorescence was set at 525 nm (FITC channel). For control experiments, T38 sequence was replaced by T32 sequence serving as a non-binding molecule. The experiments were done in triplicate. The percentage of fluorescent particles was determined by setting the gating line at fluorescence intensity greater than 700 arbitrary units (au).
Statistical analysis
The means and standard deviation (SD) of each experiment were calculated based on triplicate datasets. Student’s t-test was used to determine a significant difference between the means of two datasets. Statistical significance was set at *P <0.05.
RESULTS
Characteristics of polystyrene particle investigated by flow cytometry
Flow cytometric analysis is achieved by moving particles through a laser beam, and light that emerges after each pass is captured. When acquiring data on the flow cytometer, a forward scatter (FSC) and side scatter (SSC) signal from the particles are recorded. FSC describes the size of particles and SSC describes the granularity of particles [24]. 2D scatter plots of polystyrene particles at different concentrations showed no change upon decreasing the particle concentration (Figure 2A). To gather more detailed information, histograms of fluorescent signals (Figure 2B) which described the fluorescence intensity of the particles were collected. The x-axis shows fluorescence intensity, and the y-axis shows the count or number of particles in the sample. Similarly, with the scatter plot, different concentrations of polystyrene did not affect the fluorescence histogram. Hence, to save costs, the minimum concentration of polystyrene particles for further investigation was 0.0078 mg/mL as there was no difference in the signal.
To test the hypothesis that PDGF-BB could be coated onto PS, the growth factor was incubated with the particles for 30 minutes. After the incubation, the amount of PDGF-BB left in the solution was quantified by ELISA. The results showed that PDGF-BB in a freshly prepared solution was approximately four times higher than that in the supernatant (Figure 3). The majority of PDGF-BB was therefore coated on PS. After coating, the binding recognition of this growth factor toward PDGF-BB aptamer was further investigated. As reported in previous studies the coating of proteins onto polymeric substrates such as polystyrene, polyvinyl chloride, and polypropylene shows successful applications in ELISA since the coated proteins which are antigens or antibodies maintain their binding functionality to a specific target [25, 26].
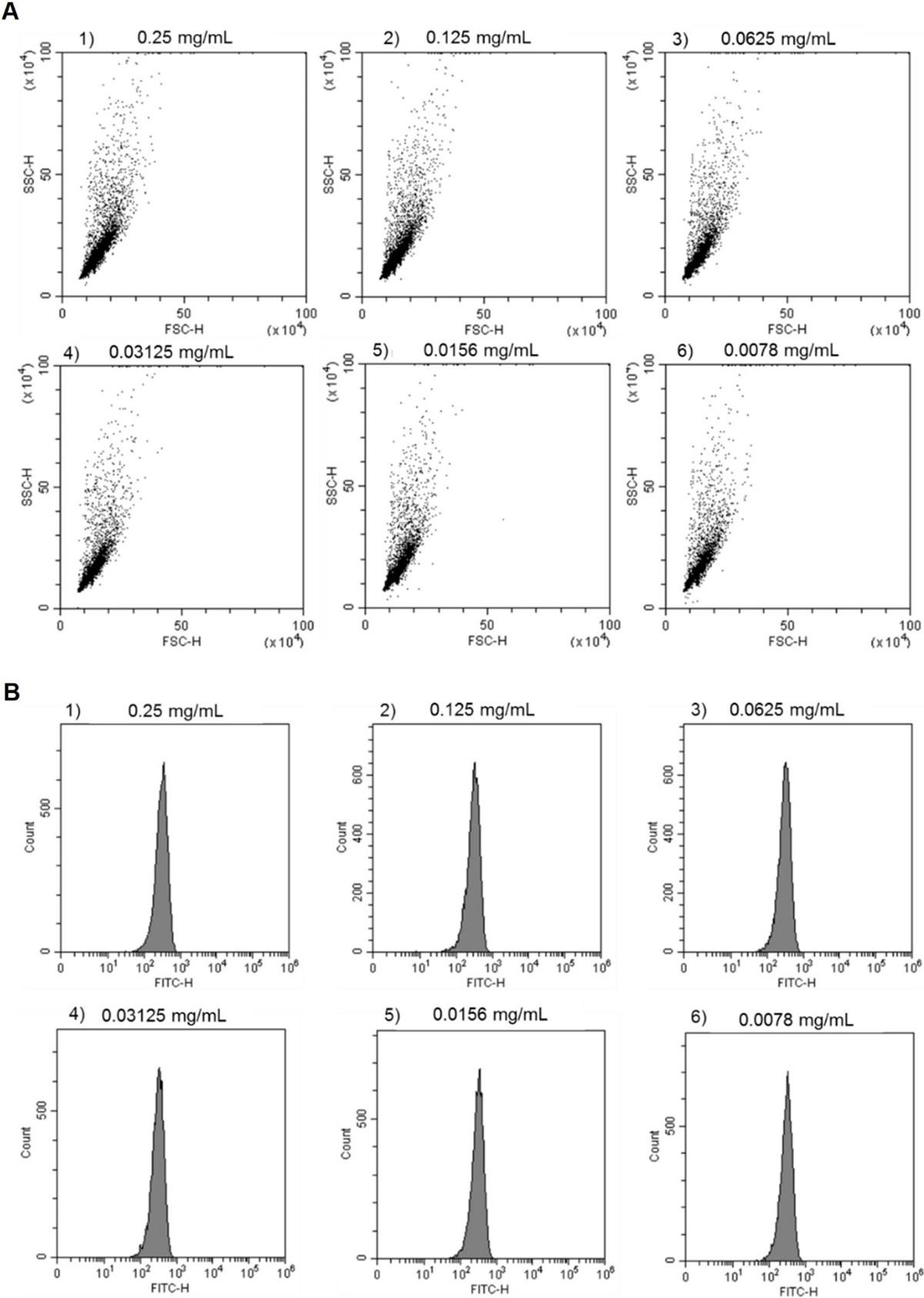
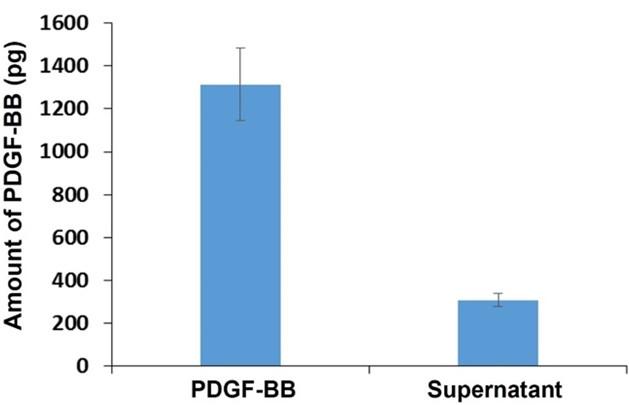
Effect of washing step
PDGF-BB-coated particles were incubated with T38 and T32 aptamer and then washed with buffer solution for 2, 5 and 7 cycles before collecting flow cytometry data. Flow cytometry histogram (Figure 4A) presented fluorescent signals from tested particles at different washing cycles. The particles treated with T38 aptamer showed a fluorescence shift toward the right-hand side of the horizontal axis, in comparison to the control experiments which were untreated and T32 aptamer-treated particles. The fluorescence shift indicated the binding ability of T38 aptamer. As depicted in Figure 4B, the percentage of fluorescent particle intensity decreased when increasing the washing cycle, because the fluorescence-labeled aptamers that bind specifically to the particles were washed away in each washing cycle. Therefore, the particles treated with the T38 aptamer with 2 cycles of washing exhibited the highest percentage of fluorescent particles.
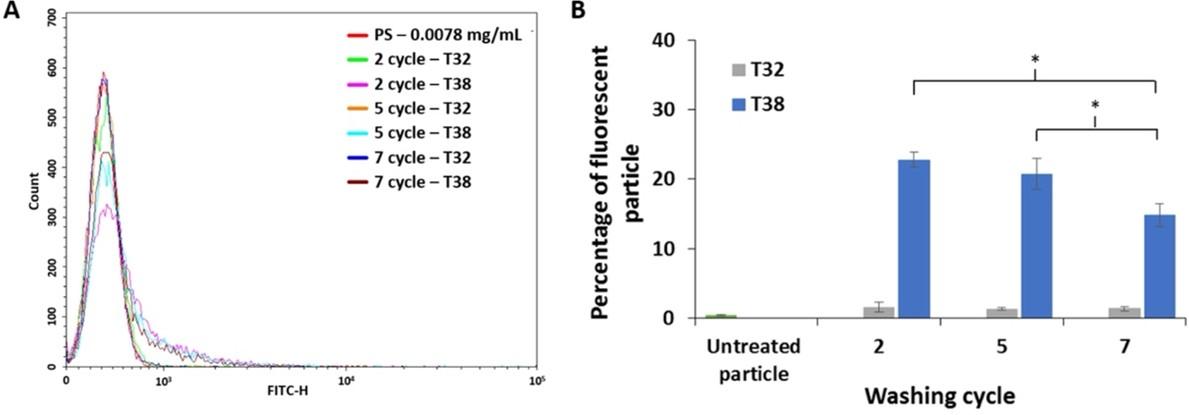
Effect of PDGF-BB concentration on aptamer binding ability
The concentration of PDGF-BB was another parameter that had an impact on aptamer binding ability. Flow cytometry histogram (Figure 5A) presented a fluorescence intensity on varying concentrations of PDGF-BB: 50, 100, and 500 ng/mL. The particles treated with T38 aptamer exhibited fluorescence shift in comparison to the untreated and particles treated with T32 aptamer. Thus, T38 aptamer has binding ability toward PDGF-BB-coated particles. The percentage of fluorescent particles for the samples that had been treated with 50, 100, and 500 ng/mL of PDGF-BB was shown in Figure 5B. The PS treated with 100 ng/ml of PDGF-BB emitted more fluorescence than the particles treated with 50 and 500 ng/mL of the growth factor. This indicated the optimal level of PDGF-BB for coating. At 50 ng/mL of PDGF-BB, the PS was not coated by this growth factor efficiently resulting in less detected fluorescent signal. Whereas a higher amount of PDGF-BB used for PS coating could result in either unbound or detached growth factors remaining in the particle suspension, leading to fewer available aptamers on the PS. Consequently, the detected fluorescent signal was low.
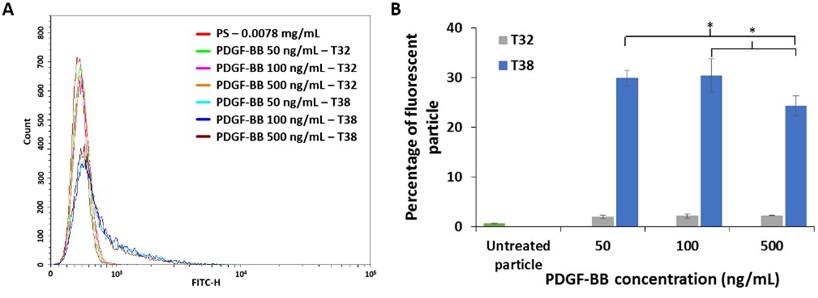
Effect of particle concentration
After aptamer incubation, fluorescent signals of PS at concentration of 0.0078, 0.0039 and 0.00195 mg/mL was recorded (Figure 6A). In comparison to the control experiments, PS treated with T38 aptamer exhibited a shift in fluorescent signals indicating the aptamer binding. By fixing the amount of PDGF-BB and varying the amount of PS, the mean percentage of fluorescent particles increased as the concentration of PS decreased (Figure 6B). This indicated less availability of PDGF-BB coated on PS upon increasing the number of particles. Moreover, the 0.00195 mg/ml PS had the highest fluorescence intensity percentage, and this PS concentration would be suitable for verification of PDGF-BB aptamer binding in further experiments.
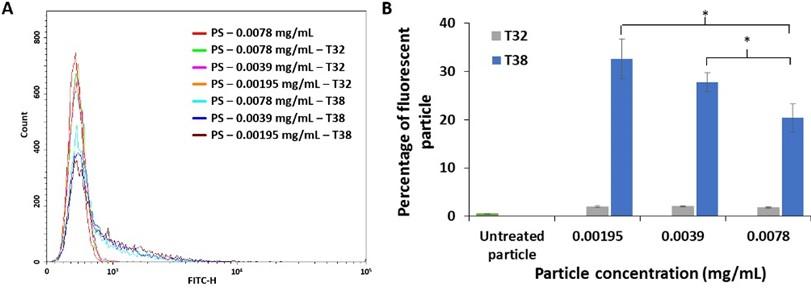
Effect of aptamer concentration
The histogram (Figure 7A) demonstrated a fluorescence intensity of PS at designated concentrations of aptamer: 50, 100, and 200 nM. When compared to the untreated and T32-treated particles, PS treated with T38 aptamer exhibited a fluorescence shift. The percentage of fluorescent particles increased proportionally with the aptamer concentration (Figure 7B). At 200 nM T38 aptamer, the fluorescence percentage was the highest level for the experiment condition. This led us to use the 200 nM aptamer for the evaluation of the aptamer binding since it might yield a distinguishable fluorescent signal.
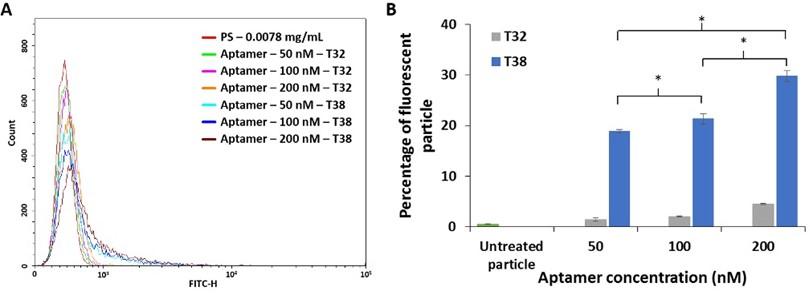
Investigation of PDGF-BB aptamer binding
The experiment was further carried out to examine the binding of four aptamer sequences reported in our previous work. The optimal conditions of the washing cycle, concentration of PDGF-BB, concentration of polystyrene particles, and concentration of aptamer for the experiment were 2 cycles, 100 ng/mL, 0.0078 and 0.00195 mg/mL, and 200 nM, respectively. At both concentrations of PS, the percentage of fluorescent particles treated with T34, T36 and T38 aptamer was greater than that treated with T32 aptamer and untreated particles (Figure 8). This indicated that T34, T36 and T38 aptamer inherited binding ability toward PDGF-BB. Moreover, the binding ability of T36 and T38 sequences was distinctive, while that of T34 sequence was moderate, and T32 sequence showed no binding. The findings agreed with the previous works.
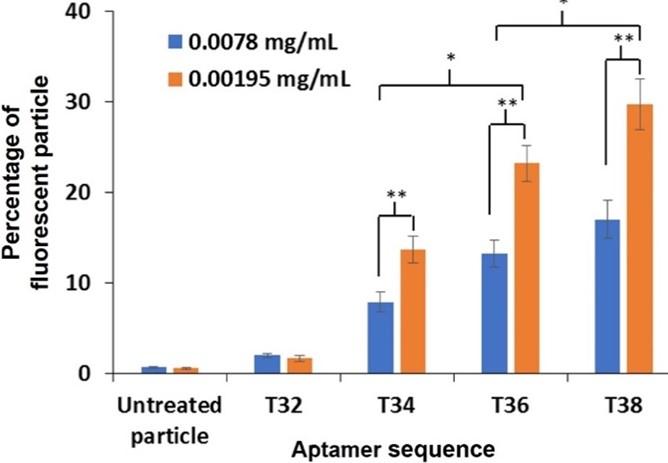
DISCUSSION
The molecule of our interest is PDGF-BB aptamer which originally contains 86 nucleotides. It demonstrates specific binding with a dissociation constant (KD) of 10-10 M [27]. This aptamer shows promise in several applications. For cancer application, PDGF-BB aptamer had an anti-proliferation effect on colorectal cancer cells, since the aptamer could down-regulate ERK1/2 signaling pathway [16]. Combinational treatment of PDGF-BB aptamer and Taxol, a chemotherapy drug, could enhance antitumor effect of chemotherapy in tumor stroma [6]. Afterward, a molecular hybrid composed of PDGF-BB aptamer, nucleolin aptamer, cholesterol, and doxorubicin exhibited key characteristics from each integrated species. It is a promising platform for colon cancer treatments [18]. In diagnosis, PDGF-BB aptamer has been used to be a probe for highly sensitive and selective detection [28]. In drug delivery systems, programmable protein release systems utilizing aptamer-functionalized hydrogel have been reported [29, 30]. In addition, our previous work has demonstrated that ELISA coupled with DNA hybridization was a promising strategy for studying the binding interaction of PDGF-BB aptamer [22]. This inspired us to extend the usage of polystyrene material for growth factor immobilization and use it for aptamer binding characterization. The characterization platform was designed to detect a signal from growth factor-coated particles using a flow cytometer.
A wide range of research including immunology, cell biology, pathology, and microbiology, use flow cytometry to accomplish cellular investigations such as immunophenotyping, DNA analysis, apoptosis, and cell sorting [31-33]. The flow cytometer has the capability to characterize suspended particles. In literature, the flow cytometer was used to screen aptamers binding to ciprofloxacin. The procedures were carried out by immobilizing DNA library onto PS and sorting the particles by the flow cytometer [34]. In addition, flow cytometry was used to confirm that PS was successfully functionalized with PDGF-BB aptamer [35, 36]. Furthermore, a magnetic nanogel comprising of liposome, cholesterol-bearing pullulan, and iron oxide nanoparticles was developed for drug delivery application, and one of the key characterizations for this nanogel system was a flow cytometer. The flow cytometric results indicated that the formation of the nanogel was reversible [37].
CONCLUSION
To conclude this work, PDGF-BB-coated particles were successfully prepared. The conditions for optimization including washing cycle, and concentration of PDGF-BB, PS, and aptamer were studied. Based on the flow cytometry results, the number of fluorescent particles increased when decreasing PS concentration and the washing cycle. The PDGF-BB concentration showed optimal value on the fluorescent signals. The number of fluorescent particles increased proportionally with the aptamer concentration. Aptamer binding investigation confirmed that T34, T36, and T38 aptamer had the binding ability to PDGF-BB. The growth factor-coated particle coupled with flow cytometry is a promising and alternative strategy for evaluating biomolecular binding.
ACKNOWLEDGEMENT
The authors gratefully acknowledge the financial support provided by Faculty of Science and Technology, Thammasat University (Contract No. SciGR16/2565). The authors thank Mrs. Sam Ormond, Clinical Research Center, Faculty of Medicine, Thammasat University for comments on the manuscript. The authors also thank Dr. Yuthana Tantirungrotechai for his suggestions.
AUTHOR CONTRIBUTIONS
BS and SS were involved in conception and design of the experiments. SS, PW, and KV contributed to perform the experiments. SS, PR, and BS analyzed data. SS contributed to drafting the article. JA, PR, and BS contributed to revising it critically for important intellectual content. All authors made the final approval of the version to be published.
CONFLICTS OF INTEREST
There is no conflict of interest among the authors.
References
- [1]Ansari MJ, Bokov D, Markov A, Jalil AT, Shalaby MN, Suksatan W, et al. Cancer combination therapies by angiogenesis inhibitors; a comprehensive review. Cell Communication and Signaling. 2022;20 (1): 49.
- [2]Singh SK, Bhusari S, Singh R, Saxena A, Mondhe D, Qazi GN. Effect of acetyl 11-keto β-boswellic acid on metastatic growth factor responsible for angiogenesis. Vascular Pharmacology. 2007;46:333-7.
- [3]Jian K, Yang C, Li T, Wu X, Shen J, Wei J, et al. PDGF-BB-derived supramolecular hydrogel for promoting skin wound healing. Journal of Nanobiotechnology. 2022;20.
- [4]Kramer F, Dernedde J, Mezheyeuski A, Tauber R, Micke P, Kappert K. Platelet-derived growth factor receptor b activation and regulation in murine myelofibrosis. Haematologica. 2020;105:2083-94.
- [5]Heldin C-H. Autocrine PDGF stimulation in malignancies. Ups J Med Sci. 2012;117:83-91.
- [6]Pietras K, Rubin K, Sjoblom T, Buchdunger E, Sjoquist M, Heldin CH, et al. Inhibition of PDGF receptor signaling in tumor stroma enhances antitumor effect of chemotherapy. Cancer Research. 2002;62:5476-84.
- [7]Saik JE, Gould DJ, Keswani AH, Dickinson ME, West JL. Biomimetic Hydrogels with Immobilized EphrinA1 for Therapeutic Angiogenesis. Biomacromolecules. 2011;12:2715-22.
- [8]Masters KS. Covalent Growth Factor Immobilization Strategies for Tissue Repair and Regeneration. Macromolecular Bioscience. 2011;11:1149-63.
- [9]Vorobyeva M, Vorobjev P, Venyaminova A. Multivalent Aptamers: Versatile Tools for Diagnostic and Therapeutic Applications. Molecules. 2016;21:21.
- [10]Soontornworajit B, Wang Y. Nucleic acid aptamers for clinical diagnosis: cell detection and molecular imaging. Anal Bioanal Chem. 2011;399:1591-9.
- [11]Zhou J, Soontornworajit B, Snipes MP, Wang Y. Development of a novel pretargeting system with bifunctional nucleic acid molecules. Biochem Biophys Res Commun. 2009;386:521-5.
- [12]Yao FJ, An YC, Li XD, Li ZY, Duan JH, Yang XD. Targeted Therapy of Colon Cancer by Aptamer-Guided Holliday Junctions Loaded with Doxorubicin. Int J Nanomed. 2020;15:2119-29.
- [13]Khademi Z, Lavaee P, Ramezani M, Alibolandi M, Abnous K, Taghdisi SM. Co-delivery of doxorubicin and aptamer against Forkhead box M1 using chitosan-gold nanoparticles coated with nucleolin aptamer for synergistic treatment of cancer cells. Carbohydr Polym. 2020;248:10.
- [14]Wei HY, Zhao Z, Wang YM, Zou J, Lin QY, Duan YX. One-Step Self-Assembly of Multifunctional DNA Nanohydrogels: An Enhanced and Harmless Strategy for Guiding Combined Antitumor Therapy. ACS Appl Mater Interfaces. 2019;11:46479-89.
- [15]Razmi N, Baradaran B, Hejazi M, Hasanzadeh M, Mosafer J, Mokhtarzadeh A, et al. Recent advances on aptamer-based biosensors to detection of platelet-derived growth factor. Biosensors and Bioelectronics. 2018;113:58-71.
- [16]Sae-Lim S, Soontornworajit B, Rotkrua P. Inhibition of colorectal cancer cell proliferation by regulating platelet-derived growth factor B signaling with a DNA aptamer. Asian Pacific Journal of Cancer Prevention. 2019;20:487-94.
- [17]Hasanzadeh M, Razmi N, Mokhtarzadeh A, Shadjou N, Mahboob S. Aptamer based assay of plated-derived grow factor in unprocessed human plasma sample and MCF-7 breast cancer cell lysates using gold nanoparticle supported α-cyclodextrin. Int J Biol Macromol. 2018;108:69-80.
- [18]Rotkrua P, Lohlamoh W, Watcharapo P, Soontornworajit B. A molecular hybrid comprising AS1411 and PDGF-BB aptamer, cholesterol, and doxorubicin for inhibiting proliferation of SW480 cells. J Mol Recognit. 2021;34.
- [19]Cao X, Song Q, Sun Y, Mao Y, Lu W, Li L. A SERS-LFA biosensor combined with aptamer recognition for simultaneous detection of thrombin and PDGF-BB in prostate cancer plasma. Nanotechnology. 2021;32.
- [20]Davies DR, Gelinas AD, Zhang C, Rohloff JC, Carter JD, O’Connell D, et al. Unique motifs and hydrophobic interactions shape the binding of modified DNA ligands to protein targets. Proc Natl Acad Sci U S A. 2012;109:19971-6.
- [21]Vu CQ, Rotkrua P, Soontornworajit B, Tantirungrotechai Y. Effect of PDGF-B aptamer on PDGFR beta/PDGF-B interaction: Molecular dynamics study. J Mol Graph. 2018;82:145-56.
- [22]Vu CQ, Rotkrua P, Tantirungrotechai Y, Soontornworajit B. Oligonucleotide Hybridization Combined with Competitive Antibody Binding for the Truncation of a High-Affinity Aptamer. ACS Comb Sci. 2017;19:609-17.
- [23]Zhang HY. The specific binding between aptamer and its target molecules investigated by microcantilever sensor. Advanced Materials Research2013. p. 455-62.
- [24]Bakke AC. The principles of flow cytometry. Laboratory Medicine. 2001;32:207-11.
- [25]Sakamoto S, Putalun W, Vimolmangkang S, Phoolcharoen W, Shoyama Y, Tanaka H, et al. Enzyme-linked immunosorbent assay for the quantitative/qualitative analysis of plant secondary metabolites. Journal of Natural Medicines. 2018;72:32-42.
- [26]Japp NC, Souchek JJ, Sasson AR, Hollingsworth MA, Batra SK, Junker WM. Tumor Biomarker In-Solution Quantification, Standard Production, and Multiplex Detection. Journal of Immunology Research. 2021;2021.
- [27]Green LS, Jellinek D, Jenison R, Ostman A, Heldin CH, Janjic N. Inhibitory DNA ligands to platelet-derived growth factor B-chain. Biochemistry. 1996;35:14413-24.
- [28]Qiu L-P, Wu Z-S, Shen G-L, Yu R-Q. Highly Sensitive and Selective Bifunctional Oligonucleotide Probe for Homogeneous Parallel Fluorescence Detection of Protein and Nucleotide Sequence. Analytical Chemistry. 2011;83:3050-7.
- [29]Battig MR, Soontornworajit B, Wang Y. Programmable Release of Multiple Protein Drugs from Aptamer-Functionalized Hydrogels via Nucleic Acid Hybridization. Journal of the American Chemical Society. 2012;134:12410-3.
- [30]Soontornworajit B, Zhou J, Snipes MP, Battig MR, Wang Y. Affinity hydrogels for controlled protein release using nucleic acid aptamers and complementary oligonucleotides. Biomaterials. 2011;32:6839-49.
- [31]Zand E, Froehling A, Schoenher C, Zunabovic-Pichler M, Schlueter O, Jaeger H. Potential of flow cytometric approaches for rapid microbial detection and chracterization in the food Industry—a review. Foods. 2021;10.
- [32]Chiang SCC, Bleesing JJ, Marsh RA. Current Flow Cytometric Assays for the Screening and Diagnosis of Primary HLH. Frontiers in immunology. 2019;10:1740.
- [33]Adan A, Alizada G, Kiraz Y, Baran Y, Nalbant A. Flow cytometry: basic principles and applications. Critical Reviews in Biotechnology. 2017;37:163-76.
- [34]Fang X, Li W, Gao T, Ain Zahra QU, Luo Z, Pei R. Rapid screening of aptamers for fluorescent targets by integrated digital PCR and flow cytometry. Talanta. 2022;242.
- [35]Soontornworajit B, Zhou J, Wang Y. A hybrid particle-hydrogel composite for oligonucleotide-mediated pulsatile protein release. Soft Matter. 2010;6:4255-61.
- [36]Soontornworajit B, Zhou J, Zhang Z, Wang Y. Aptamer-functionalized in situ injectable hydrogel for controlled protein release. Biomacromolecules. 2010;11:2724-30.
- [37]Mizuta R, Sasaki Y, Katagiri K, Sawada SI, Akiyoshi K. Reversible conjugation of biomembrane vesicles with magnetic nanoparticles using a self-assembled nanogel interface: single particle analysis using imaging flow cytometry. Nanoscale Advances. 2022;4:1999-2010.