Hemolytic, antioxidant, and phospholipase A2 neutralization efficacy of Asparagus racemosus, Withania somnifera, Syzygium cumini, Psidium guajava, Basella alba, Morus indica, Morus laevigata, and Morus latifolia
Abstract
Bangladesh is abundant in medicinal plants. The bioactive compounds in medicinal plants provide novel therapeutic opportunity of improving human health. But the benefit-risk balance is usually less considered during formulation of traditional medicine. However, the plants included in this study were not studied before regarding their hemolytic and anti-venom properties. Thus, the current study aimed to evaluate and compare the hemolytic activity, antioxidant activity, and phospholipase A2 (PLA2) enzyme neutralization efficacy of aqueous and methanolic extracts of eight known medicinal plants including Asparagus racemosus (root), Withania somnifera (root), Syzygium cumini (leaf), Psidium guajava (leaf), Basella alba (leaf and seed), Morus indica (leaf), M. laevigata (leaf), and M. latifolia (leaf). The results of the hemolytic activity test suggested that the methanolic extracts of S. cumini, P. guajava, B. alba (leaf and seed), M. indica, M. laevigata, and M. latifolia showed significantly higher hemolytic activity compared to their aqueous extracts. The methanolic extracts of A. racemosus, W. somnifera, P. guajava, M. laevigata, and M. latifolia showed remarkable antioxidant activity. Moreover, the results of the PLA2 enzyme neutralization activity test revealed that both aqueous and methanolic extracts of P. guajava, S. cumini, and B. alba (leaf) have significant PLA2 neutralization capacity. Altogether, the aqueous extracts of all plants, except A. racemosus root, have ignorable hemolytic effect. Both of the aqueous and methanolic extracts of M. latifolia showed the maximum antioxidant activity. Additionally, P. guajava and S. cumini leaf aqueous extracts have promising PLA2 inhibitory effect which may become the potential source of bioactive compounds for anti-venom drug development.
INTRODUCTION
Plants are regarded as a precious source of natural wealth in the majority of the developing nations in the world, including Bangladesh. Plants are used in a number of healthcare initiatives. Medicinal plants and plant-derived products have been utilized in traditional medicinal systems since ancient times. Traditional medicine formulation utilized more than 500 medicinal plant species for treating numerous ailments [1]. Substances found in medicinal plants are known as active principles. Plants that are rich in active compounds are of great interest in term of therapeutics [2]. Recent industrial processes and advanced scientific techniques focus on bioactive molecules. Combining different medicinal plants or plant parts deserves particular attention since such herbal mixtures are the source of increased biologically active compounds [3]. Herbal drugs were used in various formulations of numerous plant products. Approximately 80% of the drugs that are being taken in recent days are medicinal plant-derived [4]. In modern times, many medicines made from plants are used to treat illnesses like Alzheimer's disease, cancer, diabetes, diarrhea, heart disease, Parkinson's disease, and sores. According to the report of the World Health Organization (WHO), around 80% people in developing countries are depending on their local medicinal plants, whereas one-fourth of the prescription medicines and 11% medicines that are specified as vital are plant-derived [5]. In recent years, more people are interested in plant-based medicines as a way of finding alternatives to chemical drugs that work well and have fewer side effects [6]. However, the development of medicine from a plant source requires a number of steps. The toxicity of the active molecule is considered a key factor during drug design [7]. The active components of plants used as medications have activity including antioxidant, anti-inflammatory, anti-hemolytic, anti-diarrheal, antibacterial, anti-parasitic, antiviral, etc.
The major insight into the relationship between chemicals and living things at the cellular level is revealed by hemolytic activity. Any agent that hemolyzes healthy cells is cytotoxic. It is important to figure out how well medicinal plants work and if they are dangerous. Plant extracts can have a positive effect on the membrane of red blood cells, while many plants have severe negative effects. Therefore, the commonly used medicinal plant species must be undergone for hemolytic activity evaluation. In vitro hemolytic assay utilizing spectroscopic techniques offers an efficient and simple method for quantifying hemolysis.
Plant-derived biologically active compounds may function as replacement of natural antioxidants in human body [8]. Antioxidants interrupt free radical chain reactions and retain proper cellular signaling in human physiology which prevents cell damage [9]. Numerous chronic and degenerative diseases, such as aging, atherosclerosis, cancer, diabetes mellitus, ischemic heart disease, and neurodegeneration are caused by oxidative cell damage arising from free radicals. Free radicals and other reactive oxygen species (ROS) are the byproducts of aerobic metabolism, produced by the body's natural processes and sometimes by environmental factors and pollutants [10, 11]. They play an important role in many physiological functions, including defense, inflammation, signal transduction, adhesion, proliferation, apoptosis, etc. Plant-based natural antioxidants are of most interest globally because they are non-toxic. Many free radical-scavenging antioxidants come from fruits, vegetables, and teas. Antioxidant-rich food plants protect against carcinogenesis. Dietary supplementation strengthens the antioxidant system and prevents chronic illness. Thus, plant species must be searched for effective pharmacological antioxidants.
Numerous active plant constituents are promising contenders for the development of anti-venom drug molecules. Traditional medicine practitioners have characterized numerous plant-based remedies based on their experiences and observations. People who are involved in agriculture and their family members are the most vulnerable people to snakebite; thus, snakebite has been defined as “disease of poverty” [12]. Serious morbidity and mortality issues are caused by snake envenomation, especially in Asia. WHO defined snakebite as a “neglected tropical disease”, but persons having snakebite seek emergency care facility in rural and tribal areas, particularly in Bangladesh, India, and Nepal [13]. Approximately 100,000 victims die among 2.7 million snakebite victims each year. According to WHO’s report, around 80% of the population throughout the world depend on traditional medicine to get rid of numerous ailments [14]. Fortunately, WHO took an initiative to lessen the snakebite-induced mortality and disabilities worldwide to 50% by 2030 [15]. Phospholipase A2 (PLA2), a venom enzymatic entity, is responsible for various toxic effects, such as cardiac arrest, deformations, kidney failure, and amputations [16]. Snake poison can be treated with alkaloids, acids, steroids, glycoproteins, phenols, terpenoids, and other chemical groups that are found in plant products. Medicinal plants with anti-venom properties could be used to help people who have been bitten by snakes right away. This is especially important in rural areas. In Bangladesh, different plant species are being used for a long time to treat snakebites, but there is no in-deep study to elucidate whether they work or are safe.
The plants including Asparagus racemosus (root), Withania somnifera (root), Syzygium cumini (leaf), Psidium guajava (leaf), Basella alba (leaf and seed), Morus indica (leaf), M. laevigata (leaf), and M. latifolia (leaf) were not studied for elucidating their hemolytic effect and the protective aptitude against snake venom before in Bangladesh. Thus, the present study aimed to evaluate hemolytic activity, antioxidant properties, and PLA2 enzyme neutralization efficacy of the aqueous and methanolic extracts of the abovementioned eight medicinal plants growing in Bangladesh. Another objective of this study was comparing the effect of aqueous and methanolic extracts of the plants.
MATERIALS AND METHODS
Selection and collection of plant materials
The nine plant samples from eight medicinal plants were selected based on their medicinal properties (Table 1). Roots of A. racemosus and W. somnifera, fresh leaves and seeds of B. alba were collected from the Botanical Garden, University of Rajshahi, Bangladesh. Fresh leaves of P. guajava and S. cumini were collected from the Regional Fruit Research Station, Rajshahi, Bangladesh. Fresh leaves of M. indica, M. laevigata, and M. latifolia plants were collected from the Bangladesh Sericulture Research and Training Institute, Rajshahi, Bangladesh. Importantly, A. racemosus, W. somnifera, S. cumini, P. guajava, and B. alba are available under accession number RR098, RR564, RR242, RR302, and RR078, respectively in the herbarium of the Department of Botany in University of Rajshahi, Bangladesh. M. indica, M. laevigata, and M. latifolia are available under accession number BSRM-42, BSRM-29, and BSRM-50, respectively in the herbarium of the Bangladesh Sericulture Research and Training Institute (BSRTI), Rajshahi, Bangladesh.
Table 1. A short description of the plant samples used in this experiment.
Preparation of plant extracts
Firstly, the collected fresh leaves, roots, and seeds were washed well with distilled water. Subsequently, they were washed with 70% ethanol and finally again with distilled water. Then the collected materials were dried at room temperature (RT). The dried materials were kept in an air incubator at 65°C for 7-10 days. After drying well, the plant materials were powdered using an electric grinder machine. The powder was stored in an airtight container and kept in a dark, cool place for further use. For aqueous and methanolic extract preparation, 25 mL of distilled water and methanol were taken into 18 (9 for aqueous extract and 9 for methanolic extract) different test tubes and 2 g of powdered plant material was added to each corresponding test tube. They were mixed well by vortexing and then sonicated at 20 Hz for 5 min at 30 sec intervals after each 30 sec sonication at RT. Then, the extracts were incubated at RT for 1 h and filtered with a vacuum pump filtration system as described by Hasan et al. [26]. Finally, the filtrated crude extracts were stored at 4°C for further use.
Hemolytic activity test
Ex vivo hemolytic activity test was carried out according to the methods described by Yang et al. [27] with minor modification. For this assay, fresh human blood was collected in a citrated tube and mixed gently. This study was performed following the guideline set by the Institutional Animal, Medical Ethics, Biosafety, and Biosecurity Committee (IAMEBBC) for Experimentations on Animal, Human, Microbes, and Living Natural Sources (No. 31/320/IAMEBBC/IBSc), Institute of Biological Sciences (IBSc), University of Rajshahi, Rajshahi, Bangladesh. Of note, blood from a healthy donor was collected with consent. The tubes were centrifuged at 5000 rpm (Heraeus Multifuge X1R, Thermo Scientific, USA) for 20 min to separate the red blood cells (RBC). After that, the RBC was washed 4 times with 1% PBS. Then a total of 18 samples (9 for aqueous extracts and 9 for methanolic extracts) were taken into marked tubes to evaluate their hemolytic activity by using three different concentrations including 25 µL, 50 µL, and 100 µL. The samples were then mixed with 10% RBC and 1% PBS buffer and incubated at 37°C for 1 h. Then the supernatant was transferred into a sterilized flat bottom 96-well microtiter plate after spinning the tubes at 3000 rpm for 5 min. After that, the absorbencies were measured at 540 nm using a MultiSkan EX, UV-Vis spectrophotometer (Thermo Scientific, USA). SDS (1%) solution was used as standard. Finally, the percentage of hemolysis was calculated using the following formula:
Percentage (%) of hemolysis=(A_t-A_n)/(A_c-A_n )×100
where, Ac is the absorbance of the control (water solution), An is the absorbance of the control (saline solution), and At is the absorbance of the extract-treated sample.
Antioxidant activity test
The antioxidant activity test was done as described by Hasan et al. [28] with minor modification. To carry out this assay, at first 6 tubes were taken for each sample, and 0 µL, 25 µL, 50 µL, 100 µL, 150 µL, and 200 µL of crude aqueous and methanolic extracts were added into the tubes. Then absolute methanol was added to make the final volume 1000 µL. Subsequently, 1.5 mL of 0.1 mM 2,2-Diphenyl-1-picrylhydrazyl (DPPH) solution was added to each tube and the tubes were left at RT for 30 min in a dark place. Then, absorbance of each sample was measured at 517 nm using a spectrophotometer. Ascorbic acid (0.2 mg/mL) was used as the standard. Finally, the percentage (%) of DPPH radical scavenging was calculated according to the equation articulated below:
Percentage (%) of DPPH inhibition = [(Ac – At)/Ac] × 100
where, Ac is the absorbance of the control and At is the absorbance of the extract-treated sample.
Finally, 50% inhibition concentration (IC50) values were calculated by plotting the inhibition percentages against different concentrations on scatter graph for both standard and test samples.
PLA2 neutralization efficacy test
The PLA2 neutralization activity test was conducted according to the methods described by Cardoso et al. [29] with minor modification. For this assay, a snake venom stock solution was prepared by dissolving 1.0 mg of lyophilized Naja naja snake venom in 1.0 mL of ddH2O. Besides, the egg yolk suspension was also prepared by mixing an egg yolk in 250 mL saline water. Then, experimental extracts were taken into wells of flat bottom 96-well microtiter plate. Three different concentrations (low = 1.5 µL, medium = 3 µL, and high = 6 µL) of each plant sample were used to evaluate dose-dependent effect. Thereafter, 5 µL of snake venom solution and 85 µL of egg yolk suspension were added to each well. A required amount of tris-HCl (pH = 7.4) was added to each well to reach the final volume 100 µL. Finally, OD values were measured at 740 nm after 10 min incubation to evaluate the neutralization activity of the tested plant samples. The control for this experiment was a mixture of venom, egg yolk solution, and tris-HCl buffer. The percentage of neutralization of PLA2 enzyme by plant extracts as well as control was calculated using the following equation:
Percentage (%) of PLA2 neutralization = [(At – Ac)/At] × 100%
where, Ac is the absorbance of the control and At is the absorbance of the extract-treated sample.
Statistical analysis
All data are presented as mean±SD (standard deviation) of three independent experiments. Statistical difference among the groups was evaluated using GraphPad Prism (version 8) software. One-way ANOVA following Tukey’s multiple comparison test was done for calculating statistical difference. The bar and scattered line graphs were prepared using Microsoft Excel 2013.
RESULTS
Effect of extracts on hemolysis
A. racemosus (roots) showed the highest hemolytic activity for both aqueous and methanolic extracts (Figure 1, Figure 2). At 100 µL dose, the aqueous extract of A. racemosus (roots) showed the highest (91.411%) hemolytic activity, which is almost same as the standard solution (1% SDS, hemolytic activity 93.186%) (Figure 1); while the aqueous extract of B. alba (seeds) showed the lowest (3.651%) hemolytic activity. The hemolytic activity of the aqueous extract of A. racemosus (roots) was 87.218% at 25 µL, 89.462% at 50 µL, and 91.411% at 100 µL dose. The aqueous extract of B. alba (seeds) showed 1.968%, 2.575%, and 3.651% at 25 µL, 50 µL, and 100 µL dose, respectively. The aqueous extract of other plant samples showed less than 10% hemolytic activity at 100 µL dose (Figure 1).
On the other hand, the highest hemolytic activity for methanolic extract was shown in the case of roots extract of A. racemosus (92.561%) at 100 µL concentration, while the lowest activity was shown by the roots extract of W. somnifera (33.609%) in comparison to standard solution (Figure 2). The hemolytic activity of the methanolic extract of A. racemosus (roots) was 90.335% at 25 µL, 91.402% at 50 µL, and 92.561% at 100 µL dose. The methanolic extract of W. somnifera (roots) showed 2.382%, 8.910%, and 33.609% hemolysis at 25 µL, 50 µL, and 100 µL dose, respectively. The methanolic extract of B. alba (seeds) showed 87.236%, 87.770%, and 90.786% hemolytic activity at 25 µL, 50 µL, and 100 µL dose, respectively. All of the other methanolic extracts of selected plants showed more than 85% hemolytic activity, including the leaves of S. cumini (91.991%), leaves of P. guajava (88.405%), leaves of B. alba (91.788%), seeds of B. alba (90.786%), leaves of M. indica (89.959%), leaves of M. laevigata (88.754%), and the leaves of M. latifolia (90.933%) (Figure 2).
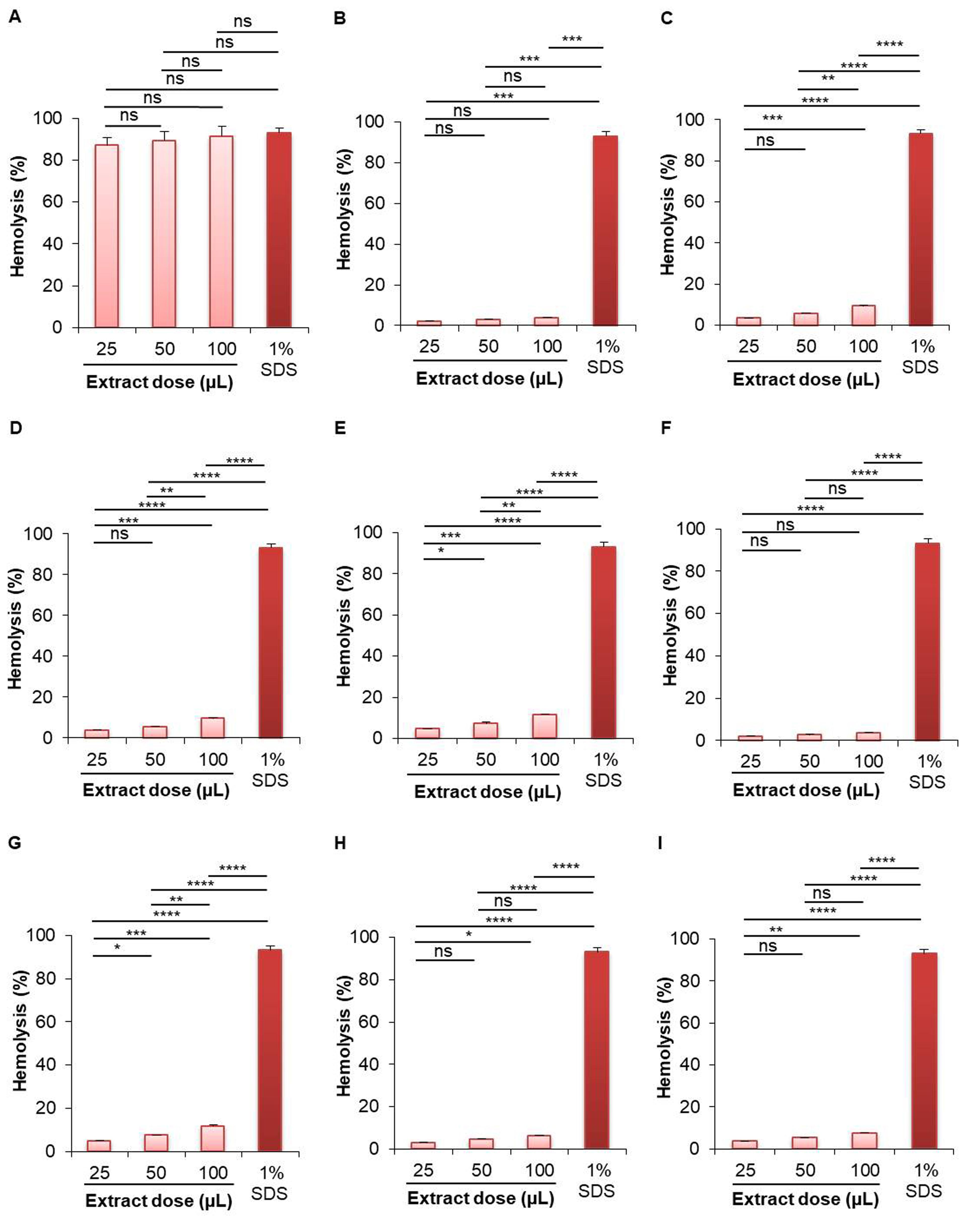
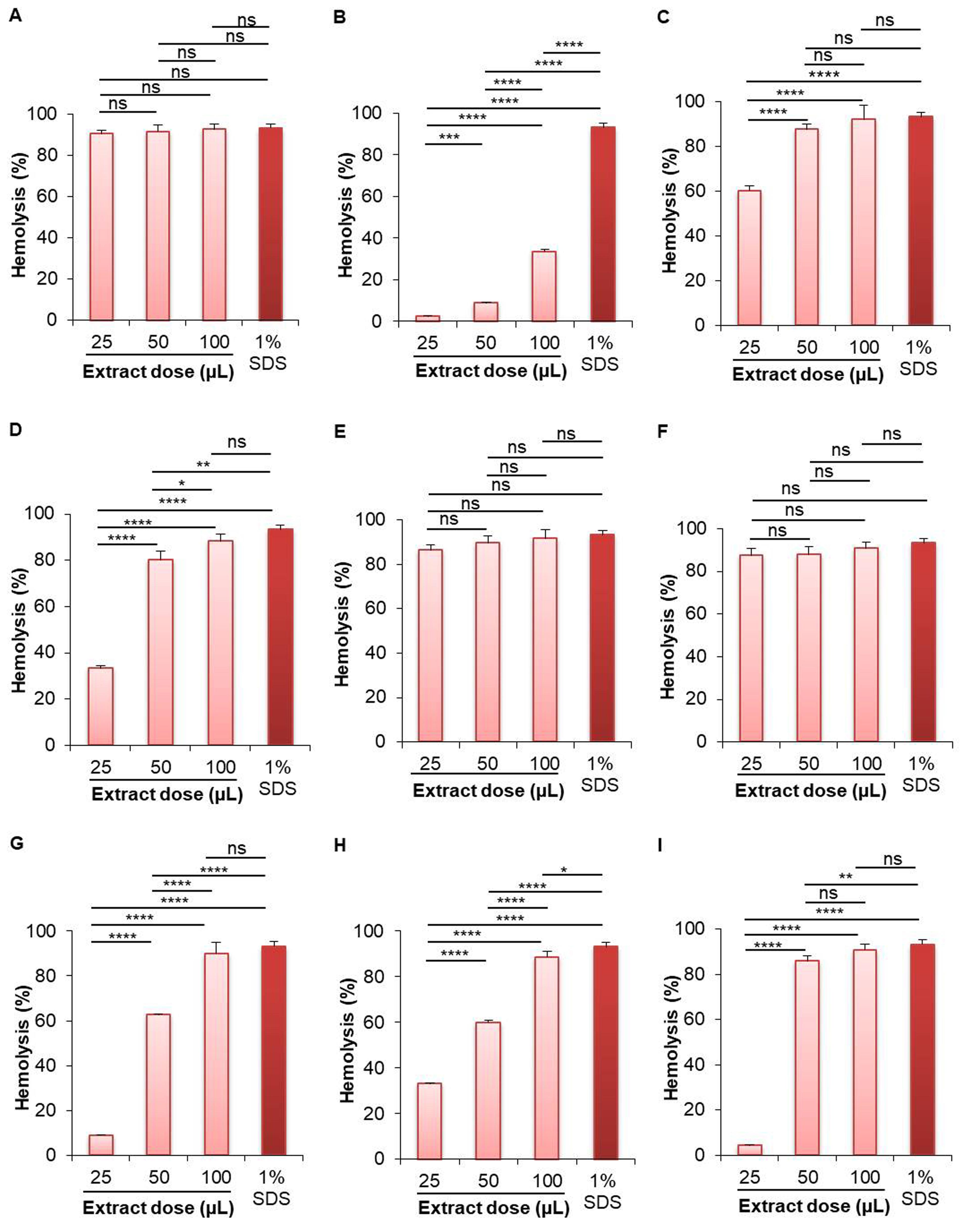
Effect of extracts on DPPH free radical inhibition
According to the results of the antioxidant activity test, the 50% DPPH radical inhibition concentration (IC50) values of aqueous extract for nine samples were calculated as roots of A. racemosus (167.556 µL), W. somnifera (138.821 µL), leaves of S. cumini (188.788 µL), P. guajava (162.196 µL), B. alba (278.599 µL), seeds of B. alba (135.387 µL), leaves of M. indica (157.203 µL), M. laevigata (142.553 µL) and M. latifolia (91.714 µL) (Figure 3).
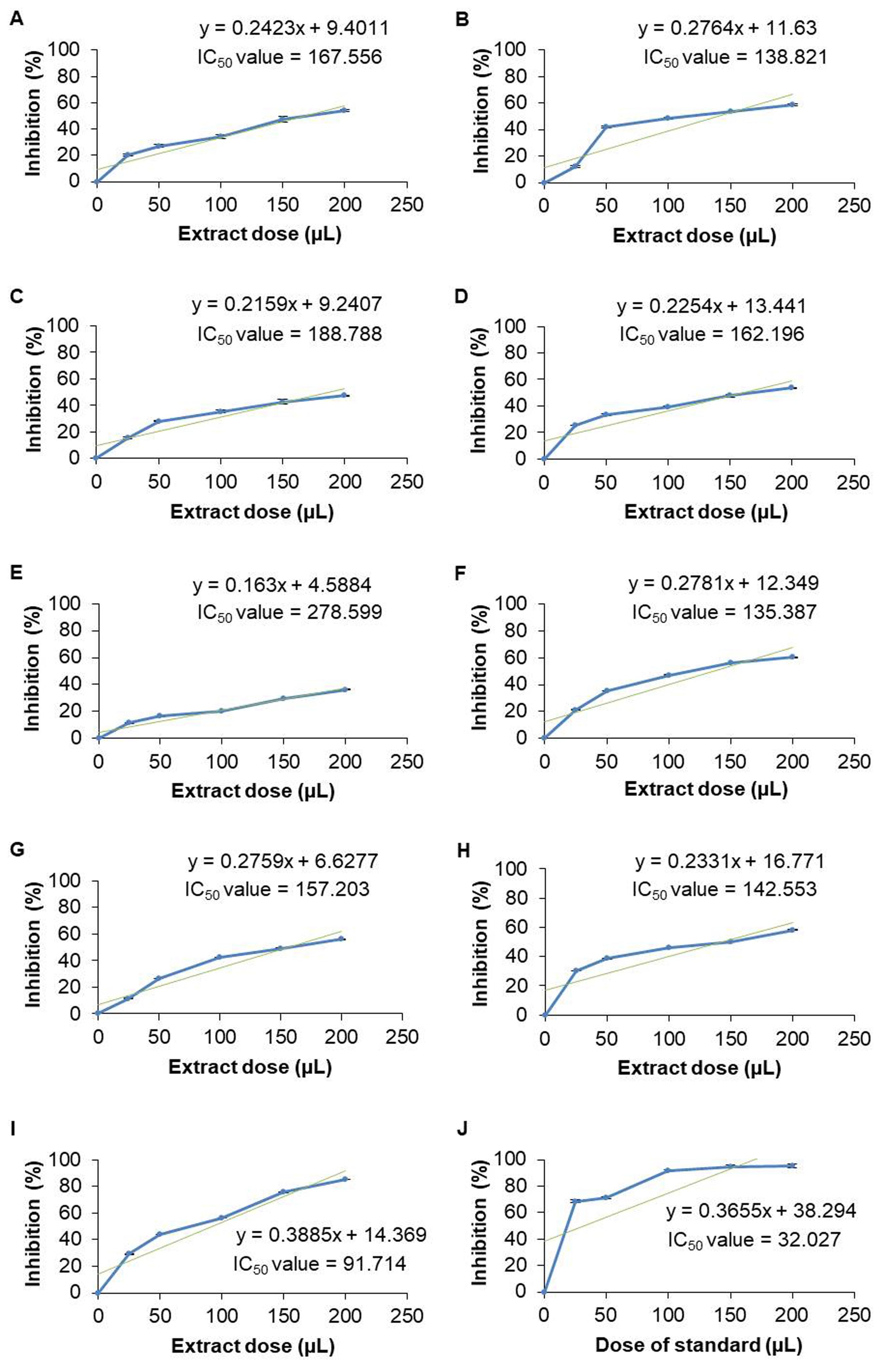
In contrast, the IC50 values of methanolic extracts were determined as follows- roots of A. racemosus (53.338 µL) and W. somnifera (61.064 µL), leaves of S. cumini (78.04 µL), P. guajava (50.547 µL), and B. alba (143.074 µL), seeds of B. alba (71.285 µL), leaves of M. indica (80.981 µL), M. laevigata (49.897 µL), and M. latifolia (32.598 µL) (Figure 4). But the IC50 value of the standard solution (ascorbic acid) was 32.027 µL.
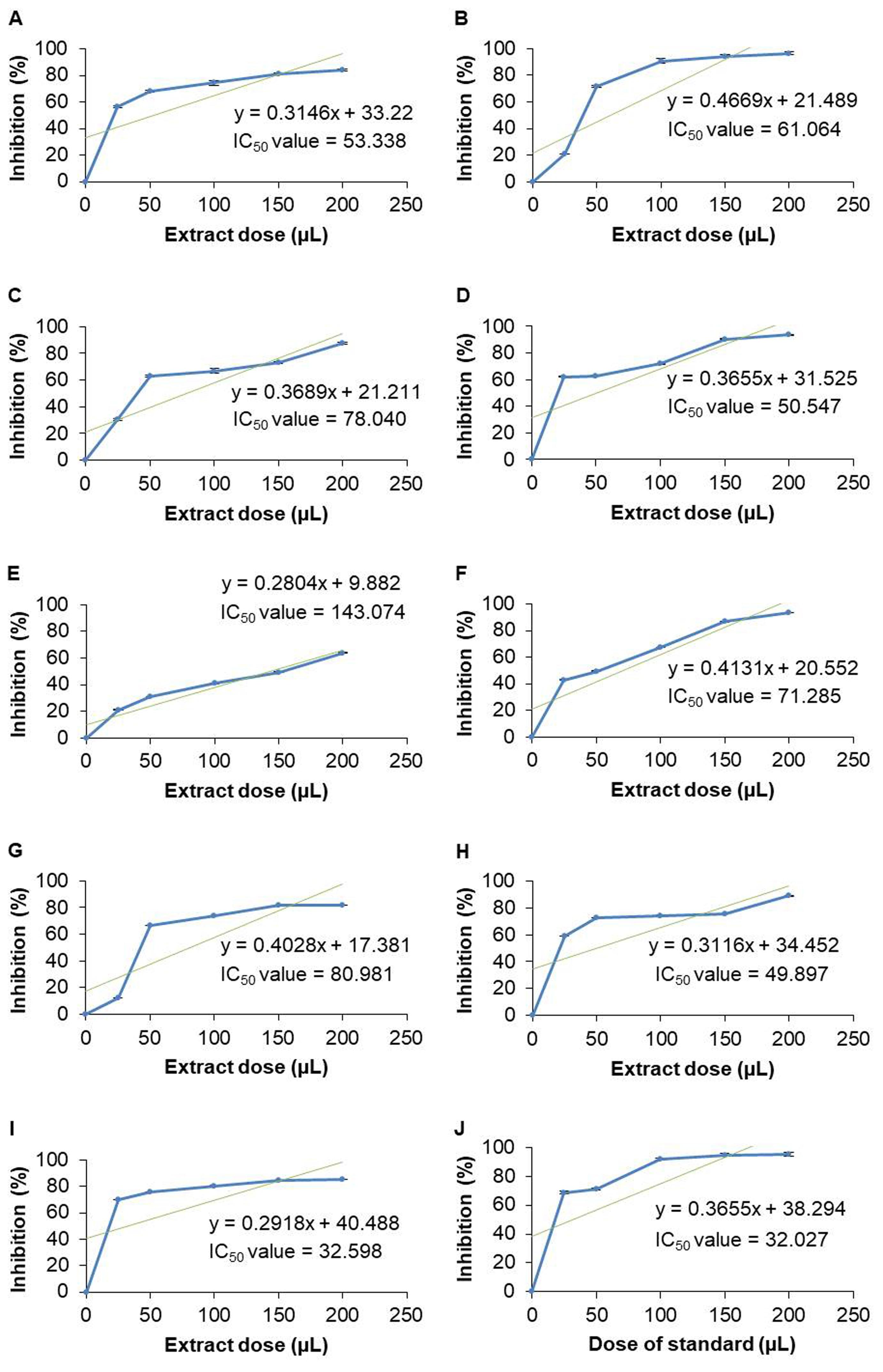
Effect of extracts on PLA2 enzyme neutralization
The results of the PLA2 neutralization activity test presented that the aqueous extract of leaves of S. cumini (88.814%) and leaves of P. guajava (88.662%) showed the best activity, while the roots of W. somnifera (25.207%) showed the lowest activity at high dose compared to their respective control (5.525%) (Figure 5). The aqueous extract of leaves of S. cumini showed 84.305%, 87.823%, and 88.814% PLA2 neutralization at low, medium, and high dose, respectively. The aqueous extract of leaves of P. guajava showed 84.260%, 87.891%, and 88.662% PLA2 neutralization at low, medium, and high dose, respectively. The roots of W. somnifera neutralized 18.598%, 19.958%, and 25.207% PLA2 at low, medium, and high dose, respectively. Besides, the aqueous extract of leaves of B. alba (71.370%), M. indica (69.582%), M. laevigata (52.614%), and M. latifolia (68.271%) showed more than 50% PLA2 neutralization activity, whereas the roots of A. racemosus (41.535%) and seeds of B. alba (28.687%) showed less than 50% PLA2 neutralization activity (Figure 5).
On the contrary, in the case of methanolic extract of selected plant samples, the highest PLA2 neutralization activity was found by the leaves of P. guajava (89.421%) and the lowest activity was found by the roots of W. somnifera (30.861%) at even high concentration in comparison to their corresponding controls (5.525%) (Figure 6). The methanolic extract of P. guajava leaves neutralized 87.589%, 88.354%, and 89.421% PLA2 at low, medium, and high dose, respectively. The methanolic extract of leaves of S. cumini showed 86.341%, 88.668%, and 89.399% PLA2 neutralization activity at low, medium, and high dose, respectively. The methanolic extract of the roots of W. somnifera inhibited 23.984%, 27.741%, and 30.861% PLA2 activity at low, medium, and high dose, respectively. Other methanolic extracts of selected plant samples showed more than 50% PLA2 neutralization activity, including the leaves of S. cumini (89.399%), leaves of B. alba (78.765%), seeds of B. alba (56.373%), leaves of M. indica (77.919%), M. laevigata (64.593%), and M. latifolia (69.820%) (Figure 6).
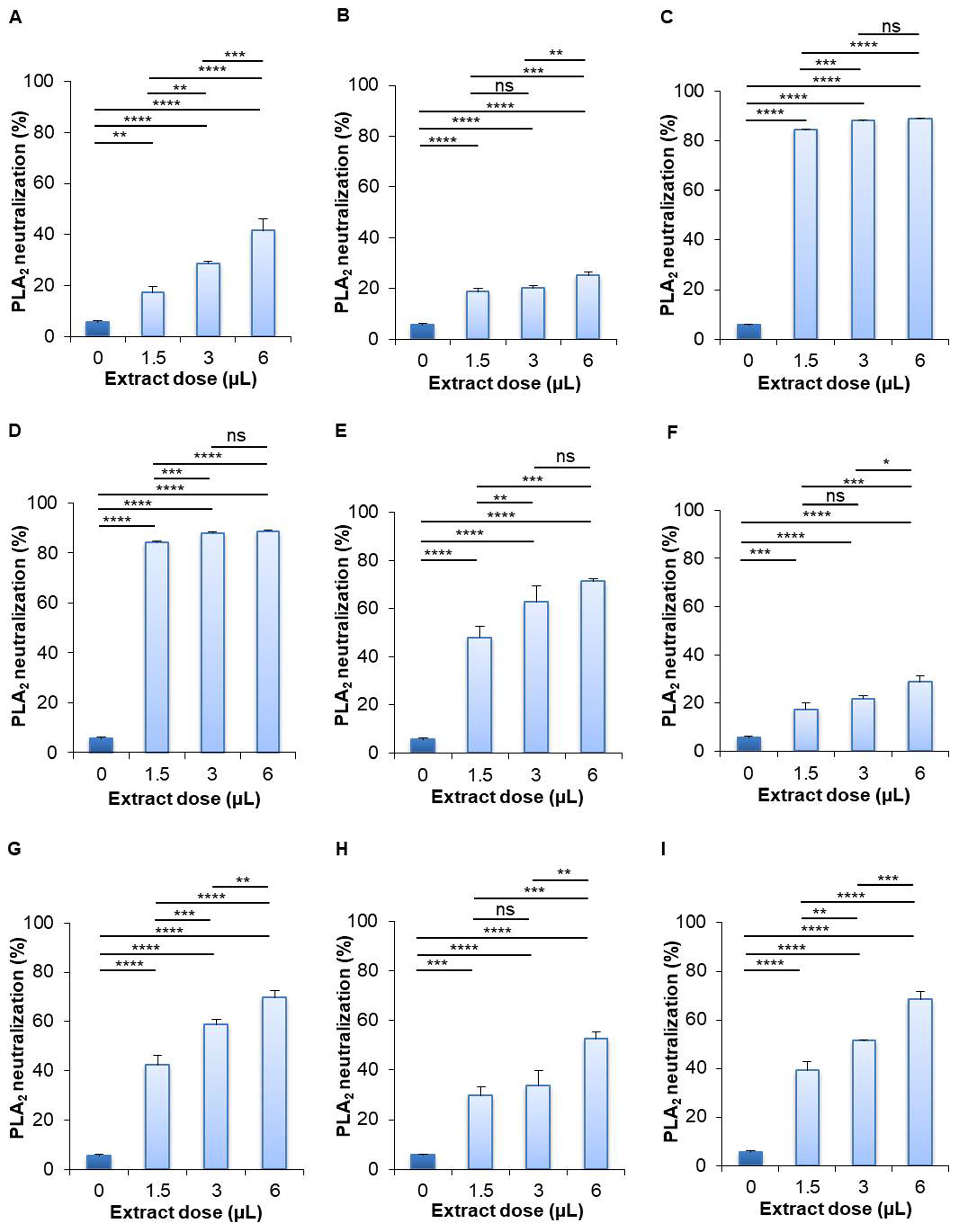
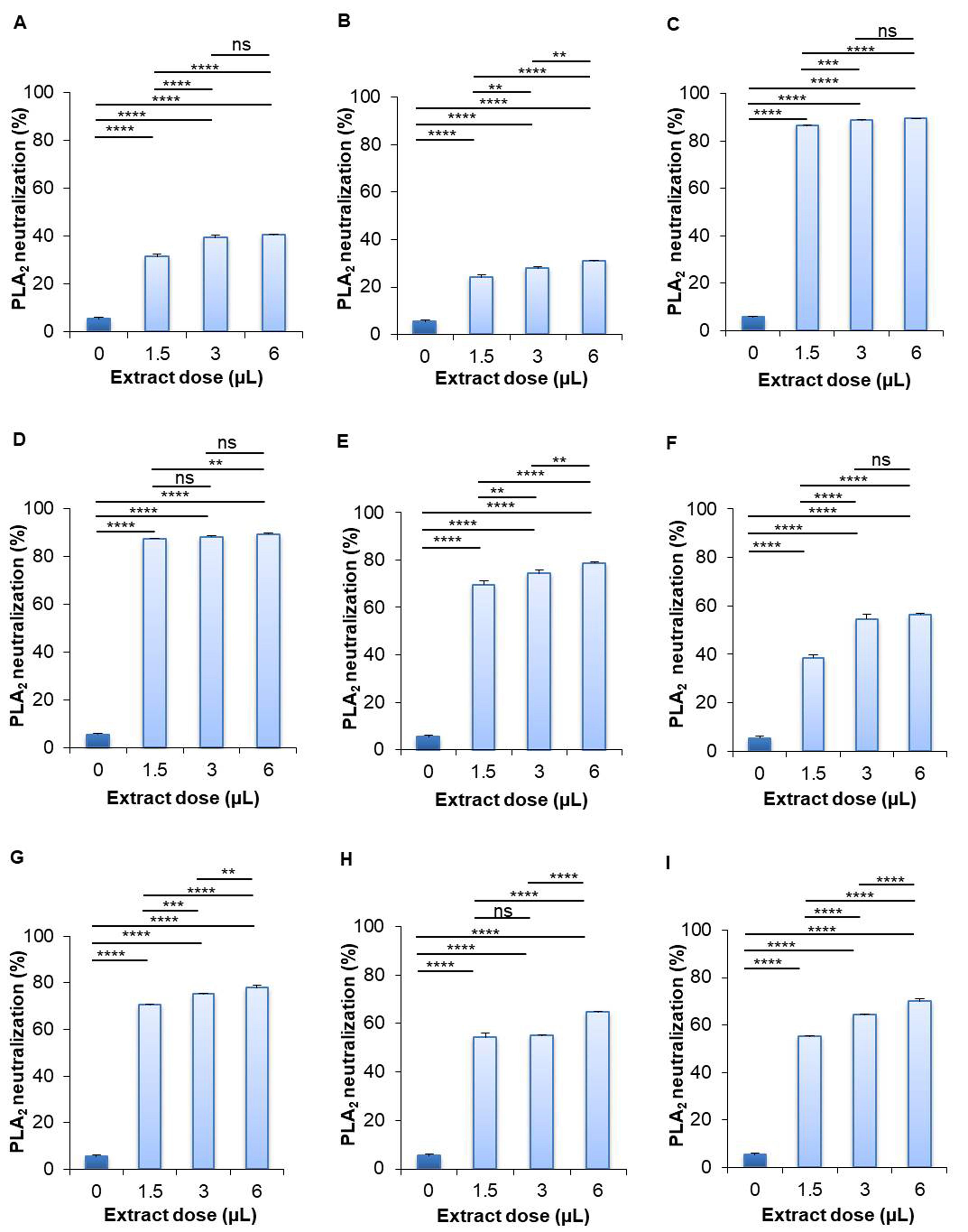
DISCUSSION
Researchers have been interested in herbal phytomedicines due to their possible biological activity. Since the beginning of time, there have been many investigations carried out. The red blood cell (RBC) model has been extensively utilized because it provides a direct indication of toxicity and is readily available. The demise of RBC is known as hemolysis and is caused by the lysis of the membrane lipid bilayer of RBC. This hemolysis relates to concentration, potency, and the chemical composition of plant extracts [30]. The present study evaluated the hemolytic activity of both aqueous and methanolic extracts and the extracts induced hemolysis to different extent. Based on percentage values of hemolytic activity, aqueous extracts of A. racemosus roots exhibited the maximum hemolytic activity without showing any significant difference among groups and compared to the SDS, while aqueous extracts of B. alba seeds exhibited the lowest (3.651%) hemolysis even at high dose extract treatment and there was no statistical difference among extract treated groups. B. alba leaf aqueous extract also has ignorable hemolytic effect. Previous research showed protective effect of B. alba leaf aqueous extract against osmotic fragility of RBC and suggested regular consumption as a protectant against hemolytic anemia [31]. In our study, aqueous extract of W. somnifera exhibited 3.807% hemolysis at even high dose. Previous studies reported that aqueous extract of W. somnifera has no hemolytic activity [32, 33]. To the best of knowledge, the current study is the first evaluating hemolytic activity of M. indica, M. laevigata, and M. latifolia leaf extract. Before us, a group showed that flavonoids from Morus sp. leaves protect from 2, 2 0-azobis (2-amidinopropane) dihy-drochloride (AAPH)-induced hemolysis in a dose-dependent manner but considered harmless [34]. In opposition to the aqueous extract, methanolic extracts of all plant samples, except W. somnifera roots (33.609%), showed approximately 90% hemolytic activity at high dose. Based on percentage values of hemolytic activity of methanolic extract, A. racemosus root exhibited the maximum (92.561%) hemolytic activity at high dose. In the case of A. racemosus, extract at all doses showed dose-dependent but statistically insignificant hemolysis. In addition to that, there was no statistical difference between the extract-treated groups and the 1% SDS standard group, which is indicative to elevated enrichment of compounds having hemolytic potential in methanolic extract of A. racemosus root. Though there is no prior report regarding hemolysis induction by W. somnifera and A. racemosus, methanolic extract of these two plants have thrombolytic potential [35]. According to the comparative analysis of hemolytic activity between aqueous and methalonic extracts, methanolic extracts of S. cumini (leaf), P. guajava (leaf), B. alba (seed), B. alba (leaf), M. indica (leaf), M. laevigata (leaf), and M. latifolia (leaf) showed higher hemolytic activity than their aqueous counterpart. To the best of our knowledge, there is no prior report regarding hemolytic effect of S. cumini (leaf), B. alba (seed), B. alba (leaf), M. indica (leaf), M. laevigata (leaf), and M. latifolia (leaf) methanolic extract. However, prior research showed that the methanolic extract of P. guajava leaf induced 50% hemolysis at 10.26 mg/mL dose [36]. Our data regarding hemolysis by methanolic extract of P. guajava leaf is not comparable with previous report [36] because of difference in extract preparation procedure but is corroborated by data of Purba et al. [36]. According to the in vitro hemolytic experiment conducted on isolated rat erythrocytes, the seed extract of Nigella sativa and Lepidium sativum induced 53% and ~33% hemolysis, respectively at 100 mg/mL dose [37]. On the other hand, aqueous extract of Putoria calabrica (leaf) is able to protect erythrocytes from damage caused by oxidation [38]. The hemolytic effect of Cymbopogon citratus and Azadirachta indica aqueous and ethanolic extract was <5% and found to be non-toxic [39]. Plant samples having >10% hemolytic effect should be avoided for medicinal purpuse [39], therefore, the aqueous extract of A. racemosus root at any amount may affect the RBC and should be aware of its medicinal formulation. Besides, methanolic extracts of all plant sample also are the matter of concern in case of traditional medicinal formulation. However, the methanolic extract of W. somnifera (root), M. indica (leaf), and M. latifolia (leaf) may retain the possibilities of their use in medicinal formulation at low dose.
A number of methods are used to determine the free radical scavenging activity of antioxidant compounds, and the DPPH free radical scavenging assay is a widely used method because it is a fast, easy, and reliable technique that does not require special and sophisticated devices [40, 41]. The therapeutic effects of methanolic extract of two mangrove species, Bruguiera gymnorrhiza and Heritiera littoralis, were studied [5] and high radical scavenging ability against DPPH, H2O2, and Fe2+ radicals was found in both species. B. gymnorrhiza and H. littoralis extracts prevented H2O2-induced lysis of human RBC and their reductive capacity rose dose-dependently [5]. The Iranian people utilize 13 historically popular plant species in folk treatment because of their excellent DPPH radical scavenging capacity with IC50 value ranging from 1.03 μg/mL by Salix sp. to 187.88 μg/mL by Allium hirtifolium, the lowest and the highest, respectively among all species [42]. Interestingly, the DPPH scavenging capacity of Salix sp., Mentha spicata, Salvia hydrangea, Zataria multiflora, Achillea tenuifolia, and Polypodium vulgare was lower than that of quercetin (IC50 value = 5.22 μg/mL), a strong and well used natural antioxidant [42]. Bruguiera gymnorrhiza is a rich source of compounds with DPPH scavenging activity with the lowest IC50 value by ethanol extract of leaf and bark compared to methanol and chloroform extracts [43]. In our experiment, we evaluated the antioxidant activity of extracts utilizing DPPH radical scavenging assay by calculating the IC50 values using the regression equations. According to the results of the antioxidant bioassay, all of the aqueous extracts, except the leaves of M. latifolia (IC50 value = 91.714) showed IC50 values greater than 100 µL; while all of the methanolic extracts, except the leaves of B. alba (IC50 value = 143.074) showed IC50 values less than 100 µL. According to an earlier study, A. racemosus root ethanolic extract's IC50 value was 468.57±3.002 µg/mL [44]. The methanolic extract of S. cumini leaves scavenged 50% DPPH at 125.39±7.55 µg/mL concentration [45], while the IC50 value of of S. cumini fruit methanolic extract was 81.4±0.6 µg/mL [46]. The P. guajava leaf methanolic extract’s IC50 value was 85.7±1.8 µg/mL [47]. The antioxidant activity of B. alba leaf and seed was determined with an IC50 value of 112.96±4.87 µg/mL and 145.34±4.38 µg/mL, respectively [22]. M. leavigata leaf aqueous extract showed antioxidant property with an IC50 value 186.76 µg/mL [24]. Another study reported the IC50 value 104.86 µg/mL of M. laevigata methanol extract [48]. M. indica leaves methanol extract scavenged 50% DPPH at 79.53 ± 0.87 µg/mL concentration [49]. The IC50 value of M. latifolia leaf methanolic extracts was 98.58 ± 7.54 µg/mL [25]. However, in our study, the standard solution (ascorbic acid) showed IC50 values of 32.027 µL. The comparison of IC50 values between methanolic extracts and standard solution showed that the methanolic extracts of roots of W. somnifera, leaves of S. cumini, leaves of B. alba, seeds of B. alba, and leaves of M. indica showed noticeable higher IC50 values than standard solution which indicated that they have less antioxidant activity. But the methanolic extracts of roots of A. racemosus, leaves of P. guajava, leaves of M. laevigata, and leaves of M. latifolia showed IC50 values that were almost close to the IC50 value of the standard solution which is indicative to high antioxidant capacity of these extracts. However, the IC50 values of aqueous extracts are significantly higher than that of methanolic extracts, which represents less antioxidant potential of the aqueous extracts.
Plants as well as plant parts are used as traditional medicine to treat the fatal effects of venomous snake bites. Pharmacological studies have reported anti-inflammatory, anti-viral, and anti-venom properties of plant extracts by neutralizing different toxic proteins [50]. Rosmarinic acid is an excellent plant-derived compound that can neutralize PLA2 enzyme in snake venom [50]. Seventeen extracts from Pakistani medicinal plants were examined for evaluating their ability to inhibit PLA2 activity in Echis carinatus venom [51]. Though all plant extracts were able to neutralize PLA2, Curcuma longa, Citrullus colocynthis, and Rubia cordifolia showed the highest (78%) inhibitory aptitude [51]. Another study evaluated venom-antagonizing characteristics of aqueous extracts of five plants including Sapindus laurifolius, Spondias pinnata, Plumeria lutea, Woodfordia fruticosa, and Croton roxburghii and found entire PLA2 and acetylcholinesterase neutralization by Spondias pinnata and W. fruticosa [52]. In our current experiment, we evaluated the efficacy of neutralizing PLA2 protein of N. naja venom. Interestingly, all plant extracts showed dramatic PLA2 neutralizing efficacy. Both of the aqueous and methanolic extracts of S. cumini (leaves) and P. guajava (leaves) showed the highest (>80%) PLA2 neutralization capacity at all experimental doses. Prior research showed that methanolic extract of S. cumini L. neutralized 50% PLA2 in the venom of Pakistani Echis carinatus [39, 51]. Extract from leaves of guava cultivars including Paluma, Pedro Sato, and Roxa inhibited PLA2 produced by B. alternatus venom [53]. The maximum inhibition was 63.16% by the Paluma cultivar, followed by 57.89% inhibition by Pedro Sato, and 52.63% by Roxa [53]. W. somnifera inhibited 30% of the PLA2 in N. naja venom [54]. However, comparative PLA2 neutralization activity between aqueous and methanolic extracts revealed better PLA2 neutralization activity by the methanolic extracts.
Altogether, the study found extreme hemolytic activity by the aqueous extract of A. racemosus (root). Consumption of fresh root of A. racemosus as traditional medicine is common and this might be a contributing factor to hemolytic anemia. Therefore, keeping this effect in consideration during eating fresh A. racemosus (root) or herbal medicine formulation is suggested. However, it found dramatic PLA2 neutralization by both aqueous and methanolic extracts of P. guajava (leaf) and S. cumini (leaf). Chewing fresh leaves of P. guajava and S. cumini after snake biting may exert protection against the fatal effects of PLA2 enzyme in snake venom. In addition to that, the aqueous extract of P. guajava (leaf) and S. cumini (leaf) can be considered safe in terms of hemolysis since the percentage of hemolysis by these two extracts is less than 10% at even high dose [39]. Moreover, the methanolic extract of W. somnifera scavenged 50% DPPH at 61.064 µL dose. But the methanolic extract of W. somnifera at medium dose (50 µL) induced <10% hemolysis and considered less toxic compared to other methanolic extracts. This ignorable hemolytic effect of W. somnifera methanolic extract might be the result of high antioxidant capacity of this extract [5, 39]. However, the limitation of this study is that the concentration (weight/volume) of extracts was not optimized after drying the filtrate.
CONCLUSION
The present study revealed the ex vivo hemolytic activity, in vitro antioxidant activity, and in vitro PLA2 enzyme neutralization efficacy of A. racemosus (root), W. somnifera (root), S. cumini (leaf), P. guajava (leaf), B. alba (leaf), B. alba (seed), M. indica (leaf), M. laevigata (leaf), and M. latifolia (leaf). The overall results of this study present P. guajava (leaf) and S. cumini (leaf) as potent sources of anti-venom drug candidates which could be used in traditional medicine to combat against snake-biting. The severe hemolytic effect of A. racemosus (root) aqueous extract represents it toxic and using it directly in folk medicine may become a cause of hemolytic anemia. On the other hand, the methanolic extract of W. somnifera may become an attractive source of antioxidant molecules.
ACKNOWLEDGMENTS
The authors are very grateful to Dr. Ariful Haque (Associate Professor, Molecular Pathology Laboratory, Institute of Biological Sciences, University of Rajshahi, Bangladesh) for providing us lab facilities to carry out this experiment. The authors are also thankful to the director of the Botanical Garden (University of Rajshahi, Bangladesh), the Regional Fruit Research Station (Rajshahi, Bangladesh), and the Bangladesh Sericulture Research and Training Institute (Rajshahi, Bangladesh).
AUTHOR CONTRIBUTIONS
Conceptualization: ZF, MAR, and FAJ; Data curation: FAJ and MMH; Formal analysis: FAJ and MMH; Investigation: FAJ, MMH, MAR, and ZF; Methodology: FAJ and MMH; Project administration: ZF and MAR; Resources: MAR and ZF; Supervision: ZF and MAR; Validation: ZF and MAR; Visualization: FAJ and MMH; Writing - original draft: FAJ, MSRS, and MJA; Writing – review & editing: ZF and MMH. All authors have read and approved the final version of the manuscript.
CONFLICTS OF INTEREST
There is no conflict of interest among the authors.
References
- [1]Anjum N, Hossain J, et al. Potential In vitro and In vivo bioactivities of Schleichera oleosa (lour.) oken: A traditionally important medicinal plant of Bangladesh. Res J Pharm Technol. 2022; 15 (1): 113-121.
- [2]Ghalloo BA, Khan KU, et al. Phytochemical Profiling, In Vitro Biological Activities, and In Silico Molecular Docking Studies of Dracaena reflexa. Molecules. 2022; 27(3): 913.
- [3]Savych A, Mazur O. Antioxidant activity in vitro of antidiabetic herbal mixtures. PhOL. 2021; 2: 17-24.
- [4]Karim M, Islam M, et al. Evaluation of antioxidant, anti-hemolytic, cytotoxic effects and anti-bacterial activity of selected mangrove plants (Bruguiera gymnorrhiza and Heritiera littoralis) in Bangladesh. Clinic Phytosci. 2020; 6(1): 1-12.
- [5]Akther Y, Nabi J, et al. Comprehensive Overview of Some Edible Medicinal Plants from Kashmir Valley: Cultural, Economic, and Pharmacological Importance. In: Masoodi MH, Rehman MU (eds). Edible Plants in Health and Diseases: Springer, Singapore, 2022, pp 137–159.
- [6]Hasan MM, Islam MS, et al. Effect of Citrus macroptera Fruit Pulp Juice on Alteration of Caspase Pathway Rendering Anti-Proliferative Activity against Ehrlich's Ascites Carcinoma in Mice. Toxicol Res. 2019; 35(3): 271–277.
- [7]Tabassum S, Ahmad S, et al. Phytochemical Profiling, Antioxidant, Anti-Inflammatory, Thrombolytic, Hemolytic Activity In Vitro and In Silico Potential of Portulacaria afra. Molecules. 2022; 27(8): 2377.
- [8]Amraoui N, Laroui H, et al. Antioxidant, Anti-Hemolytic and Anti-Inflammatory Activities of Aqueous Extract of Aerial Parts of Rosmarinus Tournefortii. Ann Rom Soc Cell Biol. 2022; 26(01): 2912-2923.
- [9]Aziz M, Ahmad S, et al. Phytochemical, pharmacological, and In-silico molecular docking studies of Strobilanthes glutinosus Nees: An unexplored source of bioactive compounds. S Afr J Bot. 2022; 147: 618-627.
- [10]Joty FA, Hasan MM, et al. Evaluation of antibacterial and antioxidant activity of three plant species from Morus genus. Int J Biosci. 2019; 14: 183-189.
- [11]Sen S, Chakraborty R, et al. Free radicals, antioxidants, diseases and phytomedicines: current status and future prospect. Int J Pharm Sci Rev Res. 2010; 3(1): 91-100.
- [12]Sivaraman T, Sreedevi NS, et al. Neutralizing potential of Rauvolfia serpentina root extract against Naja naja venom. Brazilian J Pharm Sci. 2020; 56: 1.
- [13]Singh P, Yasir M, et al. Green synthesis of silver nanoparticles using Indian male fern (Dryopteris Cochleata), operational parameters, characterization and bioactivity on Naja naja venom neutralization. Toxicol Res (Camb). 2020; 9(5): 706-713.
- [14]Omara T, Nakiguli CK, et al. Medicinal plants used as snake venom antidotes in east African community: Review and assessment of scientific evidences. J Med Chem Sci. 2021; 4:107-144.
- [15]Pach S, Le Geyt J, et al. Paediatric snakebite envenoming: the world's most neglected 'Neglected Tropical Disease'? Arch Dis Child. 2020; 105(12): 1135-1139.
- [16]Parwani N, Ganapathy J, et al. Antivenom Activity of Ethnobotanical Plants. Arch Food Sci Nutr Res. 2021; 1(1): 1004.
- [17]Sharma R, Jaitak V. Asparagus racemosus (Shatavari) targeting estrogen receptor α: - An in-vitro and in-silico mechanistic study. Nat Prod Res. 2020; 34(11): 1571-1574.
- [18]Bhatnagar M, Sisodia SS, et al. Antiulcer and antioxidant activity of Asparagus racemosus Willd and Withania somnifera Dunal in rats. Ann N Y Acad Sci. 2005; 1056: 261-278.
- [19]Ayyanar M, Subash-Babu P, et al. Syzygium cumini (L.) Skeels., a novel therapeutic agent for diabetes: folk medicinal and pharmacological evidences. Complement Ther Med. 2013; 21(3): 232-243.
- [20]Gutiérrez RM, Mitchell S, et al. Psidium guajava: a review of its traditional uses, phytochemistry and pharmacology. J Ethnopharmacol. 2008; 117(1): 1-27.
- [21]Islam MS, Rahi MS, et al. Deciphering the molecular pathways of apoptosis using purified fractions from leaf extract of Basella alba through studying the regulation of apoptosis related genes. Mol Biol Rep. 2021; 48(1): 85-96.
- [22]Islam MS, Rahi MS, et al. In Vivo Anticancer Activity of Basella alba Leaf and Seed Extracts against Ehrlich's Ascites Carcinoma (EAC) Cell Line. Evid Based Complement Alternat Med. 2018; 2018: 1537896.
- [23]Kumar RP, Sujatha D, et al. Potential antidiabetic and antioxidant activities of Morus indica and Asystasia gangetica in alloxan-induced diabetes mellitus. J Exp Pharmacol. 2010; 2: 29-36.
- [24]Rahi MS, Islam MS, et al. Differential expression of Bax-Bcl-2 and PARP-1 confirms apoptosis of EAC cells in Swiss albino mice by Morus laevigata. J Food Biochem. 2020; 44(8): e13342.
- [25]Islam MS, Jahangir CA, et al. In-vivo antiproliferative activity of Morus latifolia leaf and bark extracts against Ehrlich's ascites carcinoma. Toxicol Res. 2019; 36(1): 79-88.
- [26]Hasan MM, Reza MA, et al. Detection and Quantification of Ascorbic Acid in Citrus macroptera Fruit Pulp Juice by High Performance Liquid Chromatography. Anal Chem Lett. 2021; 11(2): 284-288.
- [27]Yang ZG, Sun HX, et al. Haemolytic activities and adjuvant effect of Astragalus membranaceus saponins (AMS) on the immune responses to ovalbumin in mice. Vaccine. 2005; 23(44): 5196-5203.
- [28]Hasan MM, Tasmin MS, et al. R. vesicarius L. exerts nephroprotective effect against cisplatin-induced oxidative stress. BMC Complement Med Ther. 2021; 21(1): 225.
- [29]Cardoso FF, Gomes AAS, et al. Neutralization of a bothropic PLA2-like protein by caftaric acid, a novel potent inhibitor of ophidian myotoxicity. Biochimie. 2020; 170: 163-172.
- [30]Zohra M, Fawzia A. Hemolytic activity of different herbal extracts used in Algeria. Int J Pharm Sci Res. 2014; 5(8): 495-500.
- [31]Arokoyo DS, Seriki AL, et al. The In-Vitro Effect of Aqueous Leaf Extract of Basella Alba L. On Osmotic Fragility of Red Blood Cells in Hbss Subjects. European J Biotechnol and Biosci. 2015; 3(10): 5-10.
- [32]El-Boshy ME, Abdalla OM, et al. Effect of Withania somnifera extracts on some selective biochemical, hematological, and immunological parameters in guinea pigs experimental infected with E. coli. Int. Sch. Res. Notices. 2013; 153427.
- [33]Girish KS, Machiah KD, et al. Antimicrobial properties of a non‐toxic glycoprotein (WSG) from Withania somnifera (Ashwagandha). J. Basic Microbiol. 2006; 46(5): 365-74.
- [34]Zheng Q, Tan W, et al. Protective effect of flavonoids from mulberry leaf on AAPH-Induced Oxidative damage in sheep Erythrocytes. Molecules. 2022; 27(21): 7625.
- [35]Shahriar M, Sharmin FA, et al. Membrane stabilizing and anti-thrombolytic activities of four medicinal plants of Bangladesh. Experiment. 2012; 4: 265-270.
- [36]Purba RA, Paengkoum P. Farang (Psidium guajava L.) Dried Leaf Extracts: Phytochemical Profiles, Antioxidant, Anti-Diabetic, and Anti-Hemolytic Properties for Ruminant Health and Production. Molecules. 2022; 27(24): 8987.
- [37]Ouattar H, Zouirech O, et al. In Vitro Study of the Phytochemical Composition and Antioxidant, Immunostimulant, and Hemolytic Activities of Nigella sativa (Ranunculaceae) and Lepidium sativum Seeds. Molecules. 2022; 27(18): 5946.
- [38]Mekhoukh N, Chougui N, et al. Phytochemical Screening and In vitro Antioxidant, Antibacterial, and Antihemolytic Activities of Putoria calabrica Leaf Extracts. Curr Bioact Compd. 2023; 19(2): 37-48.
- [39]Hussain S, Javed W, et al. Synergistic Antibacterial Screening of Cymbopogon citratus and Azadirachta indica: Phytochemical Profiling and Antioxidant and Hemolytic Activities. ACS omega. 2023; 8(19): 16600-16611.
- [40]Kedare SB, Singh RP. Genesis and development of DPPH method of antioxidant assay. J Food Sci Technol. 2011; 48(4): 412-422.
- [41]Baliyan S, Mukherjee R, et al. Determination of Antioxidants by DPPH Radical Scavenging Activity and Quantitative Phytochemical Analysis of Ficus religiosa. Molecules. 2022; 27(4): 1326.
- [42]Souri E, Amin G, et al. Screening of thirteen medicinal plant extracts for antioxidant activity. Iran J Pharm Res. 2022; 7(2): 149-154.
- [43]Haq M, Sani W, et al. Total phenolic contents, antioxidant and antimicrobial activities of Bruguiera gymnorrhiza. J Med Plants Res. 2011; 5(17): 4112–8.
- [44]Karuna DS, Dey P, et al. In vitro antioxidant activities of root extract of Asparagus racemosus Linn. J. Tradit. Complement. Med. 2018; 8(1): 60-5.
- [45]Ruan ZP, Zhang LL, et al. Evaluation of the antioxidant activity of Syzygium cumini leaves. Molecules. 2008; 13(10): 2545-56.
- [46]Qamar M, Akhtar S, et al. Syzygium cumini (L.), Skeels fruit extracts: In vitro and in vivo anti-inflammatory properties. J. Ethnopharmacol. 2021; 271: 113805.
- [47]Zahin M, Ahmad I, et al. Antioxidant and antimutagenic potential of Psidium guajava leaf extracts. Drug. Chem. Toxicol. 2017; 40(2): 146-53.
- [48]Janakirama AR, Shivayogi SM, et al. Characterization of isolated compounds from Morus spp. and their biological activity as anticancer molecules. BioImpacts. 2021; 11(3): 187.
- [49]Arabshahi-Delouee S, Urooj A. Antioxidant properties of various solvent extracts of mulberry (Morus indica L.) leaves. Food Chem. 2007; 102(4): 1233-40.
- [50]Ticli FK, Hage LI, et al. Rosmarinic acid, a new snake venom phospholipase A2 inhibitor from Cordia verbenacea (Boraginaceae): antiserum action potentiation and molecular interaction. Toxicon. 2005; 46(3): 318-327.
- [51]Fatima S, Javed T, et al. Evaluation of different Pakistani medicinal plants for inhibitory potential against Echis carinatus induced phospholipase A2 toxicity. Pak J Pharm Sci. 2019; 32(5): 2269-2277.
- [52]Deshpande AM, Sastry KV, et al. Neutralization of Naja naja and Daboia russelii snake venoms by aqueous plant extracts. J Appl Pharm Sci. 2022; 12(9): 086-095.
- [53]Cesar PH, Trento MV, et al. Prospection of effects of guava leaves infusion: Antigenotoxic action and enzymatic inhibition. Nat. Prod. Commun. 2017; 12(6): 957-960.
- [54]Machiah DK, Gowda TV. Purification of a post-synaptic neurotoxic phospholipase A2 from Naja naja venom and its inhibition by a glycoprotein from Withania somnifera. Biochimie. 2006; 88(6): 701-710.