Biochemical and histopathological alterations of liver and kidney of Japanese quails due to lead toxicity and effects of vitamin C
Abstract
Lead (Pb) is the most dangerous xenobiotic causing variety of negative impacts on human and animal health. Thus, the current study intended to investigate the effect of different doses of Pb on biochemical and histological abnormalities and the beneficial effects of vitamin C in the kidney and liver of quail. A total of 72 male Japanese quails, aged 12 days were allotted in four groups with four replications. Control group (T0) received normal diet, group T1 and T2 received Pb at a dose of 70 mg and 700 mg/kg body weight (bw), respectively for 21 days, while group T3 received 700 mg Pb /kg bw for 11 days followed by only vitamin C supplementation at a dose of 70 mg/kg bw without any Pb for next 10 days. After end of treatment, blood serum was collected, and the kidney and liver were taken for a histoarchitecture study. As expected, Pb-induced significant elevations of biochemical markers of liver and kidney injury were observed in different periods of treatment (group T3 at day 21) and decreased (except creatinine) following vitamin C supplementation in group T3 at day 21. Also, Pb treatment induced congestion, necrotic foci in different organs, reactive cells in central vein, degeneration of hepatocytes, necrosis in the portal area as well as renal tubular degeneration, all of which were slightly improved by vitamin C in group T3. Therefore, the findings suggest that the use of vitamin C could be a potential preventive agent against Pb-induced toxicity.
INTRODUCTION
Lead (Pb) is heavy metal, naturally present in the soil, but as a result of global industrialization it is being incorporated into the environment and become hazardous to health [1]. It is now ubiquitous, a big concern to the public health [2]. Animals and humans are exposed to Pb through ingestion and /or inhalation from contaminated sources with the consequence of multisystemic health disorders including hemopoietic, reproductive and cardiovascular disorders [1]. The underlying mechanisms include reactive oxygen species (ROS)-mediated oxidative stress, genotoxicity, and apoptosis [3] and also enhanced encephalopathy [4] and increased lipid peroxidation in brain [5]. Since the previous few decades, environmental pollution has increased [6] and industrial areas are posing risks to all living systems, including birds [7]. Birds are exposed to Pb from a number of different sources, including the general environment, industrial pollution, and agricultural processing-related contamination of water, soil, and food [8]. When taken orally, Pb is absorbed in small amounts but is slowly eliminated, therefore exposure to small amounts over extended periods of time may result in detrimental effects [9]. When Pb reaches the bloodstream, it is absorbed, with some of it becoming attached to erythrocytes and some of it is conjugated in the liver before passing to the kidney. The remainder remains in plasma to be dispersed throughout the body, where it accumulates in various organs and impairs their functions. Animals have been known to perform poorly and even die after ingesting Pb [10]. In case of poultry it causes muscle paralysis of the digestive tract, weight loss, and even starvation [11]. Additionally, Pb displays certain immunotoxin effects as stated [12]. Birds can sustain high levels of metals in a variety of tissues, including the kidney, liver, meat, and eggs [13, 14]. Such residues can easily be dispatched to human and other organisms through food chain resulting in a wide range of biochemical [14, 15] and physiological abnormalities in different systems [16]. The kidney and liver are dynamic structure in both fitness and illness. A high creatinine level in the Pb treatment indicates the impaired renal function and glomerular filtration [17] while a higher alkaline transaminase (ALT), aspartate aminotransferase (AST), and degenerated hepatocyte also indicate the hepatic malfunction [18]. Chronic nephropathy can result from long-term exposure to Pb in the environment [19]. Further, Pb toxicity has been associated with cancers of the gastrointestinal tract, female genital, renal, respiratory, myeloma, and all forms of leukemia [20].
A few research have concentrated on amelioration of Pb toxicity. Antioxidants have reportedly been shown to significantly reduce Pb toxicity [2]. Scar tissue production and wound healing are both aided by vitamin C. Through the action of electron donation or transfer, vitamin C functions as a free radical scavenger, protecting the cell from oxidative stress caused by ROS [21]. The convenient, safe, and effective dietary administration of vitamins in a wide range of doses makes them the perfect antioxidants and boosts tissue protection against oxidative stress [22]. Beside, the quail’s meat with its special qualities such as rapid digestion, minimal lipid and high in protein, is very suitable for infant, older and patients with high blood pressure [23]. Although the Pb induced effects in humans and various animals have been focused, its biochemical and histopathological impact in quail has not been fully elucidated. On the other hand, the potential of vitamin C in regard to revive the oxidative damage of liver and kidney in the quail has not yet known. Thus, the current study aimed to evaluate the properties of Pb on these macro-anatomical changes, biochemical parameters and detect the histological architectural variations in hepatic and renal sample as a result of Pb poisoning, along with the ability to antioxidative nature of vitamin C to reverse the detrimental effect of Pb.
MATERIALS AND METHODS
Chemicals
Lead acetate (II) trihydrate (316512, ≥99.999% purity) and vitamin C (A4544, 98% purity) were purchased from Sigma-Aldrich, St. Louis, MI, USA, unless otherwise stated.
Animals
Total 72 quails (12 days of old male quail) were obtained from the Dinajpur Quail Farm, Birol, Dinajpur, Bangladesh. The temperature of the farm was kept at 28±2ºC with a relative humidity of 40–70% and provided the birds with complete access to feed and water. The quail were housed under-regulated lighting (12-hour light/dark cycle). They were kept in an animal house with plenty of ventilation. For seven days, under lab conditions, birds were cared for and adapted. Following the acclimation phase, birds were randomly chosen to form 4 groups, each containing 18 birds. Animals in all groups were also weighed at intervals of three days and then divided into four treatment groups with three replicates.
All the experiments were conducted by ensuing the ethics and guidelines, including animal care followed by the Department of Anatomy and Histology of Hajee Mohammad Danesh Science and Technology University (HSTU), Dinajpur-5200, Bangladesh. The approval number was HSTU/VAS/ANH-1070 dated: 01-06-2020 (Resolution No: 10).
Experimental design
There were four experimental groups such as T0, T1, T2, and T3. The control group (T0), which received a basal diet and distilled water. Pb was administered orally to second group T1 for 21 days at a dose rate of 70 mg/kg body weight (bw) with basal diet and adlibitum distilled water. The third group (T2) received Pb orally for 21 days at a rate of 700 mg /kg bw. The last group, T3 had 700 mg Pb /kg bw for 11 days followed by only vitamin C supplementation at a dose of 70 mg/kg bw without any Pb for next 10 days (Table 1).
Table 1. Experimental treatment.
Collection of samples
Blood collection was performed from randomly selected three birds from each group for 3 time periods such as at 0 day (d0), 10th day (d10), and 21st day (d21). The jugular vein was suitable for taking blood from quail. After the end of the experimental period (21 days) and 12 h of circumvention of diet, quails from each treatment group were sacrificed to obtain viscera (Hepatic and renal organs, muscular stomach, intestinal tract, and lungs) as well as to determine the macroscopic and histological alterations of those organs. Blood was then preserved in a lithium heparinized collecting tube (BD 366667; Thermo Fisher, Waltham, Massachusetts, USA, clot activator tube) for biochemical examinations.
Histopathological analysis
The samples were maintained for fixation in 10% formalin for a 24 h period to conduct a histopathological analysis. The tissues were then left in each grade of alcohol (70, 80, 90, 95, 100%, and 100%) for 1 h to dry. The tissues were then submerged for 90 minutes each in xylene-1 and xylene-2 (214736., Sigma-Aldrich, St. Louis, MI, USA). The procedure was then repeated with the samples submerged in melted paraffin at 55-60 o C for 90 minutes. Paraffin blocks were made after the tissues had been embedded in paraffin (76242., Sigma-Aldrich, St. Louis, MI, USA). Using a microtome machine, the paraffin blocks were sliced at a thickness of 5–6 micrometers. (Leica RM2135 Manual Rotary Microtome, Germany). Sectioning was followed by floating the paraffin block's slices in warm water in a water bath. After cutting the paraffin block into slices, the slices were floated in warm water and heated to 45°C to lengthen. Hematoxylin and Eosin's (H & E) stain (H3136 and 45260., Sigma-Aldrich, St. Louis, MI, USA) was used to color the slices on glass slides for general histology study. With a light microscope, the slides were examined, and pictures were taken with Camera headed microscope (AmScope B100-E 1000X Digital Compound Binocular Microscope, USA).
Biochemical analysis
Blood samples were given a minimum of 30 minutes to clot (maximum of 60 min). Following clotting, the samples were centrifuged for 10 minutes at 6000 rpm. Then the serum was taken by pipette and mixed with available reagents (RANDOX, Crumlin, Country Antrim, United Kingdom) for analyzing ALT and AST by using biochemical analyzer (18200; HUMAN, Wiesbaden, Germany) and renal function marker (Serum Creatinine) in the blood serum were determined using a modified version of Larsen's kinetic Jaffé reaction.
Statistical analysis
Experiments were carried out at least three times. The results of biochemical parameters analyzed as a ratio against control and Pb exposed group were expressed as Mean ± Standard Error of Mean (SEM). Single factor analysis of variance (ANOVA) was done by IBM SPSS version 25 software to analyze the statistical differences between the groups and t-test was done for differences between the period of treatment. Differences were considered significant at the level of p<0.05.
RESULTS
Postmortem findings of quails with lead toxicity
Pb-treated birds showed mucosal erosion of the gizzard (Figure 1A), intestinal gas buildup (Figure 1B), change in the color, and caecal extension (Figure 1C) and pinpoint hemorrhage in the caecal tonsil (Figure 1D). Pb toxicity induced hepatomegaly (Figure 1E), as well as renal congestion and hemorrhage (Figure 1F) in quails. In addition, hemorrhage was found in lung of Pb-intoxicated quails (Figure 1F).
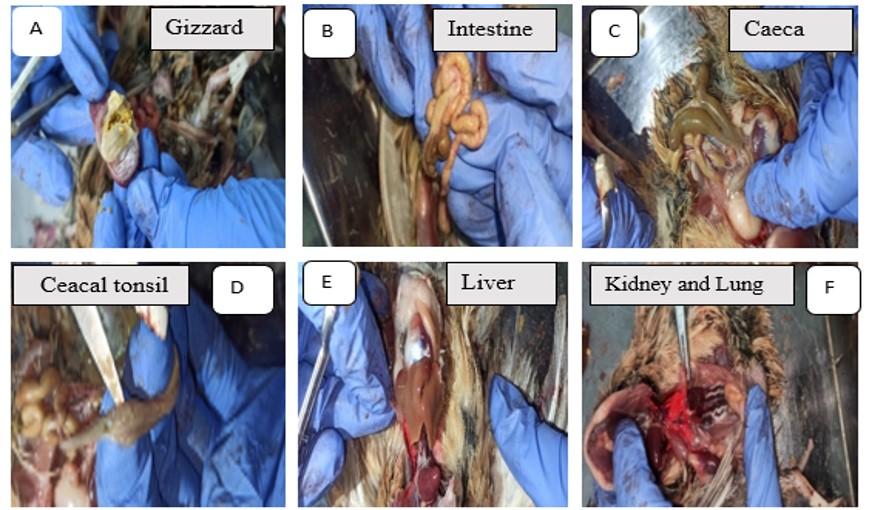
Effect of vitamin C on histopathological changes in liver of Pb-intoxicated quails
In the present study, the liver was found with normal histological architecture in the control group T0 of quails (Figure 2A). The liver of the Pb-treated quail showed somewhat congestion in the central vein (CV), increased lymphocytic infiltration, and increased von Kupper cells in Group T1 (Figure 2B). Whereas necrosis (black arrow) was found in both Group T2 and T3 of Pb-treated quail (Figure 2C-D). Also, reactive cells were found in both Group T2 and T3 (Figure 2C-D) but the amount was less in case of Group T3 (Figure 2D), and tubule was observed in Group T3 (Figure 2D).
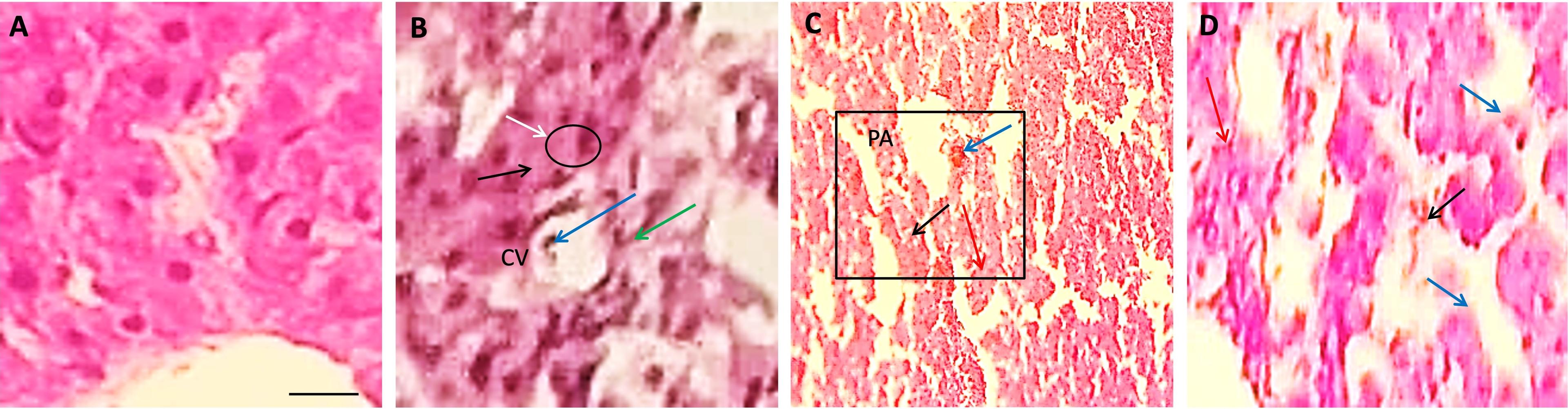
Effect of vitamin C on histopathological changes in kidney of Pb-intoxicated quails
In the present study, kidney was found normal morphological appearance such as regular glomerulus was observed in Group T0 (Figure 3A). Screening reactive cell infiltration and necrosis on renal tubules, shrinkage of glomerulus was discovered in Group T2 (Figure 3C) and increased reactive cell infiltration and necrosis on renal tubules were detected in Group T1 (Figure 3B). Less reactive cell infiltration and less necrosis on renal tubule was observed in Group T3 (Figure 3D).
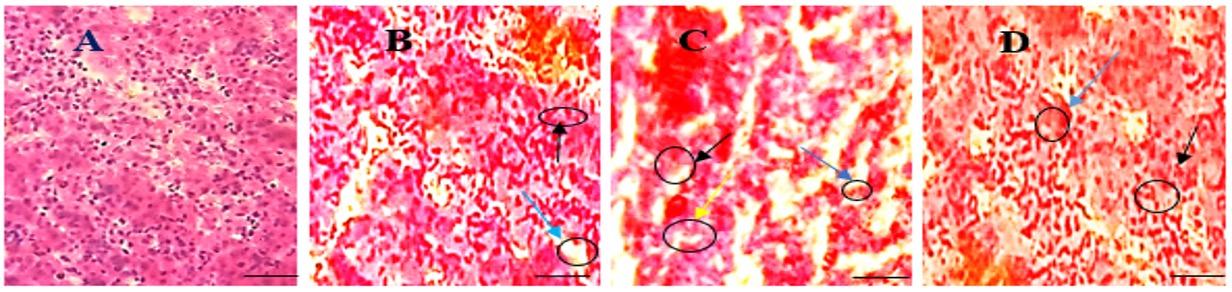
Effect of vitamin C on liver and kideny injury biomarkers in Pb-intoxicated quails
There was a significant difference in concentration of ALT (Figure 4A) and AST (Figure 4B) between d10 and d21 treatment periods in Pb-treated groups (T1, T2, and T3). Interestingly, d21 period of group T3 showed significant lower levels of ALT than d10 period which caused due to the supplementaion of vitamin C in T3 group (Figure 4A). Thus, vitamin C-treated period (from day 11 to day 21) showed significant lower ALT level than non vitamin C treated period at d10 of T3 group (Figure 4A).
In case of AST, there was no significant difference among all Pb-treated groups (Figure 4B). Further, d10 period of group T3 showed statistically higher levels of AST than the period d0. Interestingly, there was significant lower AST levels in d21 period as compared to d10 period of T3 group beacuse of vitamin C treatment in d21 (Figure 4B).
There was an increased trend of creatinine levels in all Pb-treated groups but there was no significant difference among all Pb-treated groups. Interestingly, there was remarkable significance difference in creatinine levels between the period of treatment (d0 and d10) of Pb-treated group. In addition, the value of creatinine at d21 period of T1 and T2 group shown the non-significantly increasing trend it might be due to the sex, body weight, physiological, and environmental factor. Interestingly, lower value of creatinine was due to vitamin C supplementation in T3 group (d21) as shown in Figure 4C.
Considering the data above, it is stated that Pb significantly increased the serum ALT, AST, and serum creatinine levels in different periods of treatment except d21 period of T3 group. Treatment of Pb-exposed birds with vitamin C showed marked improvement of biochemical findings at d21 period of T3.
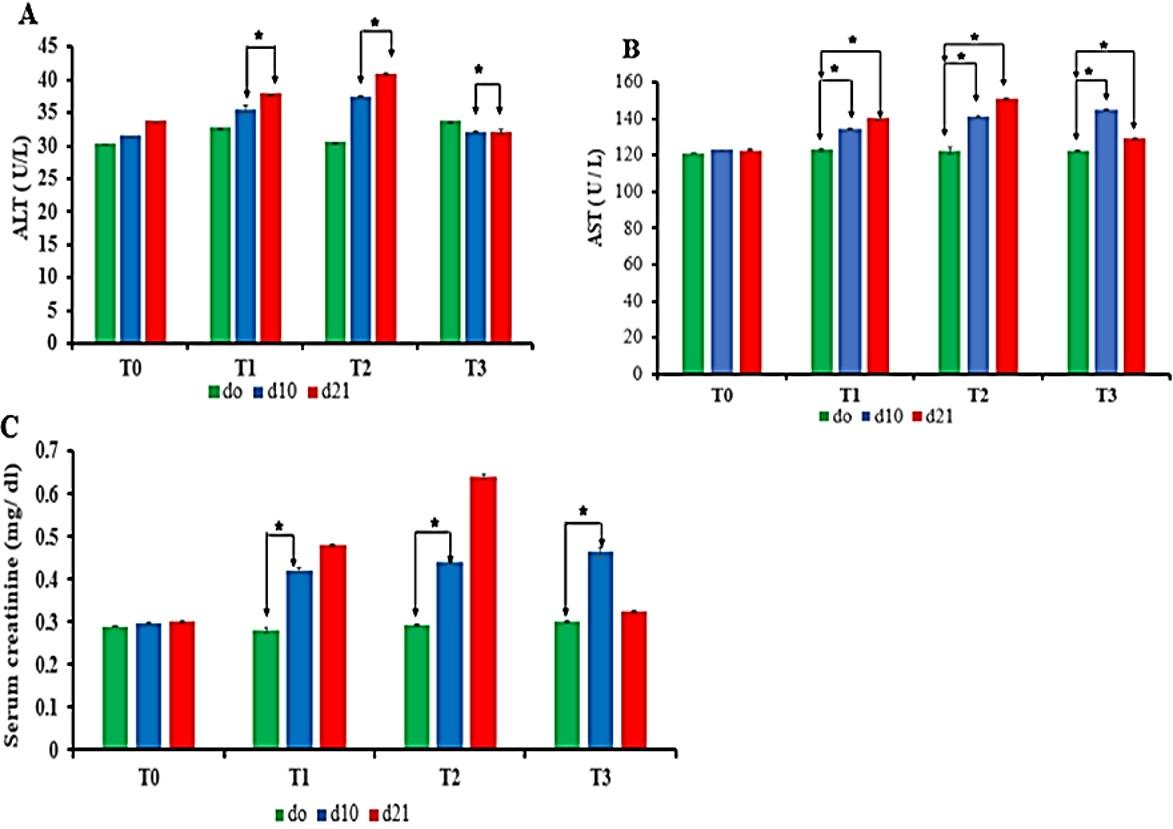
DISCUSSION
Birds treated with Pb causes different gastrointestinal, renal, and hepatic changes as well as significantly alters the hematological parameters [1]. Pb is a hazardous substances which may cause renal failure and hepatic damage [23]. It has been reported that short term exposure to Pb causes hemorrhages in the tonsils of the caecum, liver hypertrophy [24] and accumulation of gas in intestine [25]. In addition, Pb toxicity causes condense movement of the proximal gastrointestinal tract and it is associated with hematological dysfunction [26].
Repeated exposure of Pb causes significant elevation of ALT and AST, which is consistent with a previous finding [27]. Also, Pb-induced elevations in serum ALT and AST were observed in different periods of treatment that was agreed with previous findings [28]. In the present study, the ALT and AST levels were found significantly lower in vitamin C treated group (T3 group) at d21. Because vitamin C prevent or lessen the oxidative stress [22]. Additionally, Pb exposure increased the level of creatinine which was effectively decreased after vitamin C supplementation. This was little bit contradict with another group of researchers [29]. It might be due to huge tissue damage by Pb that could not revive fully by vitamin C supplementation and also may be dose factor. It is also reported that the elevation of creatinine values caused by severe renal parenchymal injury, which may have prevented glomerular infiltration [30]. However, this damage was not significantly reduced when the birds were given a vitamin C that was not in agreement with previous report [31].
An increase in lymphatic infiltration and Kupffer cells in the liver of Pb-treated group was observed. Likewise, liver necrosis was found in the Pb-treated group, but fewer Kupffer cells, less reactive cell infiltration, and less necrosis in liver of vitamin C-treated group were detected, which is consistent with previous results [32]. Several histopathological conditions and increased ALT and AST level indicate the damaging of hepatic cells that was similar with other report [33]. Consistently, reduced histopathological conditions and decreased ALT and AST level indicate the antioxidative effect of vitamin C [34]. According to the presence of responsive cells in the liver caused by connections between amino acid and stimulant of the interstitial tissue of liver and these were inhibited by the antioxidative defense mechanism [35, 36]. According to the another group of researchers [37], the higher AST and ALT levels may be due to liver injury, as evidenced by different histological alterations [38] as well as an increase in the number of Kupffer cells [39]. Recent research showed that the variations in enzyme activity are linked to the result of hepatotoxicity [40, 41]. These variations are linked to liver damage caused by metallothionein, a protein generated within the liver with a high affinity for heavy metals resulting in hepatotoxicity [42]. Pb accumulated in the kidney of quails in a dose dependent way [43, 44]. Consequently, Pb exposure increased biochemical markers, which may have been responsible for the structural, functional, and metabolic alterations by Pb toxicity in the kidney and liver [45, 46]. Thus, our findings demonstrated that vitamin C supplementation partially mitigated the harmful effects of lead on the kidney and liver of quail by suppressing Pb-induced the activity of free radicals, and the presence of Pb, glutathione, superoxide dismutase, and catalase enzyme form a complex with the Pb ,and they are important to execute normal antioxidant functions causing irreversible damage to cell membranes leading to cell death [23, 43, 47, 48].
CONCLUSIONS
In conclusion, different doses of Pb cause biochemical and histopathological alterations in liver and kidney. These alterations are due to the oxidative stress caused by ROS. Interestingly, the supplementation of vitamin C in Pb-induced toxicity in quail mitigates oxidative stress as well as the histopathological and biochemical alterations in the liver and kidney. Due to the high doses of Pb and for a short time of experiment, the vitamin C couldn’t fully reverse the anatomical, and histopathological alterations in the liver and kidney. Significant histological changes were observed in vitamin C-treated group, suggesting vitamin C as a potential preventive agent against Pb-induced toxicity. Further experimentation on low doses and long-term use of Pb and vitamin C with more population, bioaccumulation rate of Pb on different organs and transgenerational effect of Pb will be needed to get for better result of vitamin C against Pb intoxication.
ACKNOWLEDGEMENTS
This research was supported by research and development project (R&D project), Ministry of Science and Technology, Government of the People’s Republic of Bangladesh, Bangladesh Secretariat, Dhaka-1000, Bangladesh (Grant number: 44).
AUTHOR CONTRIBUTIONS
Conceptualization and design of the research, MAH and MTH; methodology, MAH; experimental investigation, MAH, MTH, NHP, and KAF; sample resources, MAH; writing and original draft preparation, MAH, SS and KAF; writing-review and editing, MAH, MTH, NHP, and KAF; supervision, MAH; project administration, MAH. All authors have read and agreed to the published version of the manuscript.
CONFLICTS OF INTEREST
There is no conflict of interest among the authors.
References
- [1]Assi MA, Hezmee MN, et al. The detrimental effects of lead on human and animal health. Vet World. 2016;9:660-71.
- [2]Gurer H, Ercal N. Can antioxidants be beneficial in the treatment of lead poisoning? Free Radical Biology and Medicine. 2000;29:927-45.
- [3]Pulido MD, Parrish AR. Metal-induced apoptosis: Mechanisms. Mutation Research/Fundamental and Molecular Mechanisms of Mutagenesis. 2003;533:227-41.
- [4]Douglas-Stroebel E, Hoffman DJ, et al. Effects of lead-contaminated sediment and nutrition on mallard duckling brain growth and biochemistry. Environ Pollut. 2004;131:215-22.
- [5]Bokara KK, Brown E, et al. Lead-induced increase in antioxidant enzymes and lipid peroxidation products in developing rat brain. Biometals. 2008;21:9-16.
- [6]Gaston KJ, Blackburn TM, et al. Habitat conversion and global avian biodiversity loss. Proc Biol Sci. 2003;270:1293-300.
- [7]Klaminder J, Bindler R, et al. Estimating the mean residence time of lead in the organic horizon of boreal forest soils using 210-lead, stable lead and a soil chronosequence. Biogeochemistry. 2006;78:31-49.
- [8]Berglund AM, Ingvarsson PK, et al. Lead exposure and biological effects in pied flycatchers (ficedula hypoleuca) before and after the closure of a lead mine in northern sweden. Environ Pollut. 2010;158:1368-75.
- [9]Madhavi D, Devil KR, et al. Modulating effect of phyllanthus fruit extract against lead genotoxicity in germ cells of mice. J Environ Biol. 2007;28:115-7.
- [10]Hoshiari E, Pourkhabbaz A. A study of heavy metal (pb, zn, cd) accumulation in different tissues of sebria gull (larus heuglini) from hara biosphere reserve. Environmental Sciences. 2011;9:-.
- [11]Handbook of ecotoxicology, second edition. 2nd ed. Boca Raton, FL: Lewis Publishers; 2003.
- [12]Ohsawa M. [heavy metal-induced immunotoxicity and its mechanisms]. Yakugaku Zasshi. 2009;129:305-19.
- [13]Farahani S, Eshghi N, et al. Determination of heavy metals in albumen of hen eggs from the markazi province (iran) using icp-oes technique. Toxin Reviews. 2015;34:96-100.
- [14]Humayun Y, Aslam A, et al. Toxic effects of lead on biochemical and histological features and their amelioration by vitamin e in japanese quails (coturnix coturnix japonica). J Avian Res. 2015;1:4-9.
- [15]Elayat W, Bakheetf M. Effects of chronic lead toxicity on liver and kidney functions. J Med Lab Sci. 2010;1:29-36.
- [16]Ghorbe F, Boujelbene M, et al. Effect of chronic lead exposure on kidney function in male and female rats: Determination of a lead exposure biomarker. Arch Physiol Biochem. 2001;109:457-63.
- [17]Hamidipour F, Pourkhabbaz H, et al. Bioaccumulation of lead in the tissues of japanese quails and its effects on blood biochemical factors. Iranian Journal of Toxicology. 2016;10:13-21.
- [18]Shaban El-Neweshy M, Said El-Sayed Y. Influence of vitamin c supplementation on lead-induced histopathological alterations in male rats. Exp Toxicol Pathol. 2011;63:221-7.
- [19]Wynne J, Stringfield C. Treatment of lead toxicity and crop stasis in a california condor (gymnogyps californianus). J Zoo Wildl Med. 2007;38:588-90.
- [20]Bhuloka Reddy S, John Charles M, et al. Trace elemental analysis of cancer-afflicted intestine by pixe technique. Biological Trace Element Research. 2004;102:265-81.
- [21]Mumtaz S, Ali S, et al. Therapeutic role of garlic and vitamins c and e against toxicity induced by lead on various organs. Environ Sci Pollut Res Int. 2020;27:8953-64.
- [22]Janisch KM, Milde J, et al. Vitamin c, vitamin e and flavonoids. Dev Ophthalmol. 2005;38:59-69.
- [23]Heriba S, Alhemmali E, et al. Toxic impact of lead acetate on histopathological alterations of kidney in the local quail (coturnix sp.) and the ameliorative role of vitamin c supplementation. J Vet Mar Sci. 2020;3:132-5.
- [24]Wani AL, Ara A, et al. Lead toxicity: A review. Interdiscip Toxicol. 2015;8:55-64.
- [25]Kelly TR, Poppenga RH, et al. Causes of mortality and unintentional poisoning in predatory and scavenging birds in california. Vet Rec Open. 2014;1:e000028.
- [26]Rodrigo L, Hernández AF, et al. Immunohistochemical evidence for the expression and induction of paraoxonase in rat liver, kidney, lung and brain tissue. Implications for its physiological role. Chemico-biological interactions. 2001;137:123-37.
- [27]Firoozichahak A, Rahimnejad S, et al. Effect of occupational exposure to lead on serum levels of lipid profile and liver enzymes: An occupational cohort study. Toxicol Rep. 2022;9:269-75.
- [28]Shalan MG, Mostafa MS, et al. Amelioration of lead toxicity on rat liver with vitamin c and silymarin supplements. Toxicology. 2005;206:1-15.
- [29]Ghanwat G, Patil AJ, et al. Effect of vitamin c supplementation on blood lead level, oxidative stress and antioxidant status of battery manufacturing workers of western maharashtra, india. J Clin Diagn Res. 2016;10:Bc08-11.
- [30]Biagini G, Misciattelli ME, et al. [electron microscopy features of renal changes in chronic lead poisoning (author's transl)]. Lav Um. 1977;29:179-87.
- [31]Offor SJ, Mbagwu HO, et al. Lead induced hepato-renal damage in male albino rats and effects of activated charcoal. Front Pharmacol. 2017;8:107.
- [32]Jarrar BM, Taib NT. Histological and histochemical alterations in the liver induced by lead chronic toxicity. Saudi J Biol Sci. 2012;19:203-10.
- [33]Al-Mansour MI, Al-Otaibi NM, et al. Histological and histochemical alterations induced by lead in the liver of the quail coturnix coturnix. Toxicological and Environ Chemistry. 2009;91:1191-203.
- [34]Abd AL-Zahra Ali R, Rasmi Huwait AJ, et al. The histopathological and oxidative stress profiles in japanese quails (coturnix japonica) induced by dietary lead. Archives of Razi Institute. 2023;78:533-40.
- [35]Abbas MT. The protective effect of quercetin on diazinon-induced oxidative stress in rats. Iraqi Nat J Chem. 2014;53:96-122.
- [36]Ahmad T, Azmi S, et al. Ameliorative effect of vitamin-c on lead-induced biochemical alterations in the serum of broilers. Applied Biological Research. 2015;17:219-23.
- [37]Richardson J. Implications of toxic substances in clinical disorders. Clinical avian medicine. 2006;2:711-9.
- [38]Kou H, Ya J, et al. The effects of chronic lead exposure on the liver of female japanese quail (coturnix japonica): Histopathological damages, oxidative stress and amp-activated protein kinase based lipid metabolism disorder. Ecotoxicology and environmental safety. 2020;190:110055.
- [39]Patra R, Rautray AK, et al. Oxidative stress in lead and cadmium toxicity and its amelioration. Veterinary medicine international. 2011;2011.
- [40]Alijagic A, Islamagic E, et al. Effects of trivalent and hexavalent dietary chromium on blood biochemical profile in japanese quails. Bulgarian Journal of Veterinary Medicine. 2018;21.
- [41]Suljević D, Handžić N, et al. Lead exposure influences serum biomarkers, hepatocyte survival, bone marrow hematopoiesis, and the reproductive cycle in japanese quails. Biological Trace Element Research. 2021;199:1574-83.
- [42]Wong D, Merrifield-MacRae M, et al. Lead (ii) binding in metallothioneins. Met ions life sci. 2017.
- [43]Kandeel M, Abdelaziz I, et al. Nephrotoxicity and oxidative stress of single large dose or two divided doses of gentamicin in rats. Pakistan Journal of Biological Sciences: PJBS. 2011;14:627-33.
- [44]Zhang G, Zhang Y, et al. Lead exposure induced developmental nephrotoxicity in japanese quail (coturnix japonica) via oxidative stress-based pi3k/akt pathway inhibition and nf-κb pathway activation. Comparative Biochemistry and Physiology Part C: Toxicology & Pharmacology. 2023;268:109599.
- [45]Taib N, Jarrar B, et al. Ultrastructural alterations in hepatic tissues of white rats (rattus norvegicus) induced by lead experimental toxicity. Saudi J Biol Sci. 2004;11:11-20.
- [46]Ashour AERA, Yassin MM, et al. Blood, serum glucose and renal parameters in lead-loaded albino rats and treatment with some chelating agents and natural oils. Turkish journal of Biology. 2007;31:25-34.
- [47]Almansour MI, Al-Otaibi NM, et al. Histological and histochemical alterations induced by lead in the kidney of the quail coturnix coturnix. Saudi Journal of Biological Sciences. 2008;15:307-13.
- [48]Alkahtani S, Alarifi SA, et al. Detection of apoptotsis induced by gentamicin in rat hepatocytes. International journal of Zoological Research. 2009;5:161-70.