In vivo antidiarrheal effect and phytochemical characterization of Plectranthus barbatus Andrews
Abstract
Diarrheal infections remain a public health problem. The mainstays of pharmacological therapy for infectious diarrhea include probiotics, antibacterials, and intestinal adsorbents. Nevertheless, these clinical treatments are not devoid of shortcomings including prohibitive costs and adverse effects. Medicinal plants including Plectranthus barbatus (P. barbatus) have folkloric remedies against diarrhea. However, there is paucity of knowledge to scientifically validate the efficacy of P. barbatus on diarrheal infections. The study was undertaken to ascertain antidiarrheal efficacy, bioactive composition, and toxicity profiles of P. barbatus extracts. Antidiarrheal activity and acute toxicity were carried out on Swiss albino mice. Quantitative phytocompound analysis was evaluated using liquid chromatography-mass spectrometry (LCMS). Results indicated that P. barbatus extract has antidiarrheal activity whereby it prolonged the start of diarrhea, causing a decline in the occurrence of wet feces and intestinal transit significantly. Additionally, the extract reduced the accumulation of intraluminal fluid resulting in decrease in distension, intestinal overload, and water content in the fecal drops. Also, acute toxicity assay on mice showed that P. barbatus extract was non-toxic at the dosage level of 2000mg/kgbw. In addition, LCMS analysis detected presence of phytocompounds associated with antidiarrheal effects including forskolin, gallic, kaempferol, and quercetin. Findings from the current study offer a scientific validation for the folkloric utilization of P. barbatus in the management of diarrhea.
INTRODUCTION
Diarrheal diseases are defined as the movement of unformed or watery stool more than three times a day [1]. They are characterized by elevated gastrointestinal secretion and motility as well as a reduction in the absorption of fluid. The severity and frequency of diarrheal infections are aggravated by poor sanitation, hygiene practices, and inaccessibility to clean water [2]. Globally, diarrheal infections remain a public health problem, especially in children. With nearly 1.7 billion episodes and 1.3 million fatalities reported annually, diarrhea is one of causes of death in under-five children [1]. Furthermore, estimates reveal that diarrhea accounts for 15% of all child mortality that occurs each day [3].
A range of viral, bacterial, and parasitic species cause diarrheal diseases in humans. The disease can spread through the fecal-oral route, tainted food and water, and person-to-person transmission [4]. According to Breurec et al. [5], the most frequent etiological causes of diarrhea in children under five include rotavirus, adenovirus, and Escherichia coli. The pathogens induce a variety of diarrheal episodes, including acute watery diarrhea, which can lead to varying degrees of dehydration, and chronic diarrhea, which can cause nutritional losses, malabsorption, bloody diarrhea, and even mortality [6, 7].
The mainstays of pharmacological therapy for infectious diarrhea include, probiotics, antibacterials, and intestinal adsorbents [8]. Nevertheless, these clinical treatments are not devoid of shortcomings, including prohibitive costs, drug-drug interactions, and adverse effects. Furthermore, some bacteria causing diarrhea, including E. coli, are gaining resistance to conventional antibiotic drugs [9]. In view of the complexity associated with conventional treatments, there is a need to explore affordable, safe, and efficacious antidiarrheal drugs [10].
Medicinal plants are considered an alternative in the treatment of diarrhea. The anti-diarrheal efficacy of herbal plants is attributable to the diversity of their chemical properties and minimal toxicity [11]. Moreover, they are arguably available and affordable, and a large population relies on medicinal plants, especially in developing countries [12]. Several studies depict that in Sub-Saharan Africa, communities rely on the therapeutic benefits of medicinal plants as remedies for infectious diarrhea, including Lantana camara, Moringa stenopetala, Casuarina equisetifolia, and Lepidium sativamum [12, 13].
P. barbatus which belongs to the family Lamiaceae, has a wide variety of traditional medicinal uses among African communities. Traditionally, the plant is used to manage different ailments, including respiratory disorders, constipation, stomachaches and diarrheal infections [14, 15]. Nonetheless, there is a scarcity of knowledge to scientifically validate the efficacy of P. barbatus against diarrheal infections. Therefore, this study sought to determine the antidiarrheal efficacy, safety profile, and phytochemical composition of P. barbatus aqueous leaf extracts.
MATERIALS AND METHODS
Plant sample collection
Fresh leaves of P. barbatus were collected from Seme Sub-County in Kisumu County, Kenya. An accredited taxonomist authenticated the specimen and was accorded specimen voucher number ECI/202.
Plant preparation and extraction
Materials were cleaned before being dried for four weeks at ambient temperature and in shade. Pulverization of the dried plant samples was performed using a Gibson electrical miller. In 1000 mL of double-distilled water, 200g of fine powder plant materials were soaked. For 120 minutes, the extraction was performed in a water bath (Memmert, D-91126 Schwabach FRG, Germany) at 60°C for 120 minutes, followed by decantation and re-soaking for a further 2 h. The sample was sieved, and the resulting supernatant was freeze-dried (Operon, Korea Vacuum Limited, Korea) and kept at 4°C.
Ethical approval
Approval for the study was obtained from the Kenyatta University Animal Care and Use Committee (approval number: PKUA/2707/11831). Additionally, license number 402104 was granted from the National Commission for Science, Technology, and Innovation Research (NACOSTI).
Determination of in vivo antidiarrheal activity
Swiss Albino mice weighing approximately 25g and aged 6 weeks were housed in polypropylene cages and accessed food and water ad libitum in 12 h dark and light cycles. They were given one week to acclimate before the experiment began. In the selection and care of laboratory animals, the 2018 National Committee for Research Ethics in Science and Technology (NENT) guidelines were followed [16].
An aliquot of 0.5mL castor oil (Faholo Chemical LTD, Kenya) was orally administered to the mice to induce diarrhea.
Thirty-six mice of either sex were chosen at random and grouped into six groups, each having six animals. Normal control (Group I) was administered distilled water (dH2O) (vehicle). Group II (negative control) animals obtained distilled water and were induced with diarrhea 1 h later. The positive control (Group III) animals were given Loperamide (3 mg/kgbw) (Imodium ® Janssen-Cilag, Val-de-Reuil, France) and then diarrhea induced 1 h later. The test groups, groups IV, V, and VI, received orally P. barbatus extract at varying doses of 100, 200, and 400 mg/kgbw, respectively; diarrhea was induced 1 h later. Table 1 shows the summarized protocol.
Table 1. Experimental protocol for determination of antidiarrheal activity.
The mice were individually housed in polypropylene cages lined with permeable paper on the floor. These white papers were changed hourly until the 4th h. Within the observation period, the start of defecation, stool regularity, and the mass of the paper for every animal were recorded. After a 24-h storage period, each adsorbent paper was air-dried. The difference in weight between the white paper and that which contained dried stool was obtained [1, 17]. Inhibition of diarrheal and fecal output weights was computed by a formula expressed by Teferi et al. [18].
Where, WFC represents wet stool in the control group and WFT represents wet stool in the experimental group.
Determination of gastrointestinal motility
To ascertain the effects of the extract on gastrointestinal tract (GIT) passage, 36 animals were randomly sorted into six groups (6 mice each) and fasted for 18 h, though water was made available. Group I animals were given dH2O orally (vehicle) only. Group II animals were given dH2O orally (10 mL/kgbw) and diarrhea induced after 1 h, and orally given 1 mL of 5% activated charcoal suspension after 1 h. Group III (positive control) animals were given the reference drug, Loperamide (3 mg/kgbw). 1 h later, diarrhea was induced, and 1mL activated charcoal suspension was administered orally. Group IV- VI animals were orally given 100-400 mg/kgbw of the extract, respectively, followed by the induction of diarrhea after 1 h. The animals were then orally administered with 1 mL of 5% activated charcoal suspension (marker) (ULAX®, West-Coast Pharmaceutical Works Ltd. Gujarat) after 1 h. Table 2 summarizes the protocol.
Table 2. Experimental protocol for determination of gastrointestinal motility.
The mice were euthanized after half an hour with diethyl ether and dissected, whereby the small bowel the was cut up from pylorus to the cecum and the distance covered by the charcoal was measured [18]. The formula described by Abdela [17] was used in calculating percentage inhibition.
Where, Dc represents the average distance covered by the marker in the negative control group and Dt represents the average distance covered by the marker in the experimental group.
Determination of enteropooling activity
Thirty-six animals were randomly sorted into six groups (6 mice each) and fasted for 18 h, though they had free access to H2O. Group I received dH2O orally. Mice in Group II (negative control) received distilled water, followed by the induction of diarrhea 1 h later. Group III (positive control) animals were given the reference drug, Loperamide (3 mg/kgbw) and induced to have diarrhea after 1 h. Mice in the test groups received the extract orally at different concentrations and were then induced to have diarrhea 1 h later. This protocol is summarized in Table 3.
Table 3. Experimental protocol for determination of enteropooling activity.
The animals were euthanized after an hour and dissected, with the ileum taken out and weighed. The intestines’ contents were then unloaded into test tubes, and the volume was taken. The mass of the ileum after milking the contents was recorded. Using the formula depicted by Tadesse et al. [13], the difference in weight of the intestines before and after milking their contents was determined in order to calculate the percentage inhibition.
Where, MVICC is the mean capacity of intestinal contents in group II and MVICT is the average capacity of intestinal content in III, IV, V and VI groups.
Calculation of antidiarrheal index
Antidiarrheal index (ADI) of the extract was carried out using the formula by Ferede et al. [12] using a combination of the variables obtained in the previous sections (anti-diarrheal activity, gastrointestinal motility, and enteropooling activity).
Anti-diarrheal index =
Where, Pfreq- is the number of wet stool reduction (in group II), Dfreq - delay in the onset of defecation (in group II), and Gmeq –is the reduction of gut meal travel (in group II)
Dfreq
Determination of acute oral toxicity
The Organization for Economic Cooperation and Development (OECD) Guidelines 425 were followed when testing the acute oral toxicity of P. barbatus [19]. The extract was administered orally to five healthy female Swiss albino mice weighing approximately 25g. One mouse was subjected to fasting for 4 h with the provision of water before receiving the extract at dose of 2000mg/kgbw. It was then fasted for a further 2 h while strict observations were made for the basic signs of toxicity for a day. If no death was observed within the set time, the other four mice were subjected to the same procedure and closely monitored for 4 h at half-hour intervals for signs of toxicity, as described by Ferede et al. [12]. The animals were then placed in cages for observation for 14 days, with their weights taken on days 7 and 14. After 14 days of treatment, the mice were euthanized with ketamine (50 mg/mL) for organ harvesting. They were then dissected, and the liver, heart, spleen, kidney, and brain were removed for weight measurement and calculation of relative organ weights.
Determination of quantitative phytochemical profile
This was done using the liquid chromatography-mass spectrometry technique as follows: one gram of the extract was weighed with a Sartorius analytical balance, reconstituted using 1 mL of LC-MS-grade water and subjected to centrifugation at 15,000 revolutions per minute for 300 seconds. Using a glass pipette, the reconstituted extract was transferred into small, clean 1.5 mL vials. Centrifugation was undertaken to remove all particulates from the extracts, thus preventing the possibility of column blockages. From the extract, a stock solution of 10 mg/10 mL was prepared and 100 µL of the stock solution was placed into a High-Performance Liquid Chromatography (HPLC) vial (Pyrex), and 20 µL was injected into an LC-MS-Electrospray Ionization (ESI) vial.
The analysis was done using the C18 column (Agilent 6475 model, Torrance, CA). The beginning temperature was 45oC. The mobile phase was composed of water and acetonitrile with a flow rate of 0.4 mL/min. The gradient program started at 5%, B, whereas the final was 100% for 25 minutes. The mass spectrometer parameter included a dry gas flow rate of 400 L/h with a desolvation temperature of 380oC, and the spectra were achieved in positive mode with a range of 100 to 1500 m/z. Analyte peaks were all quantified using the same method, with a perfect spectrum-intensity vs. chemical formula based on the existing National Institute of Standards and Technology (NIST) chemical library.
Statistical analysis
The data was inventoried using a Microsoft Excel spreadsheet and then analyzed using Minitab software version 19. The data were descriptively analyzed and expressed as means ± standard error of the mean (SEM). For inferential statistics, one-way analysis of variance (ANOVA) and Tukey's post hoc test were performed for pairwise comparison of means. An unpaired T-test was done to compare organ weights, normal and treated. The significance level was set at 95%. Tables and graphs were used to present the data.
RESULTS
Effects of P. barbatus on castor oil-induced diarrhea in mice
In this study, mice treated with different dosage levels of P. barbatus extracts experienced significantly prolonged commencement of diarrhea in a dose-dependent trend (p< 0.05; Table 4). The longest delay in initiation of diarrhea was observed upon administration of the extract at 400 mg/kgbw (139.33±1.96), which was significant to the activity of loperamide (p > 0.05; Table 4). On the contrary, the mice in the negative control group had a significantly lower latency time of diarrheal onset as compared with those receiving the test sample (p < 0.05; Table 4).
From the study, P. barbatus extracts showed potent efficacy on the incidences of defecation in mice induced with diarrhea. Further, it was observed that there was a significant decline in the frequency of defecation in mice compared to the negative control group (Table 4; p<0.05). The highest decrease in wet fecal output was observed at the dose of 400 mg/kgbw (27.451±0.78%), followed by the doses of 200 (32.52±1.28%) and 100 mg/kgbw (46.41±0.84). In addition, the total fecal output in animals treated with an aqueous leaf extract of P. barbatus was significantly lower compared to that in the untreated group (p<0.05, see Table 4). Nevertheless, the potency of the extract dose of 200 mg/kgbw on the wet and total fecal output was similar to that of loperamide (p > 0.05; Table 4). The effect of the P. barbatus extract dose of 100 mg/kgbw on the total fecal weight was statistically lower than other dosages (p<0.05; Table 4). The study revealed further that P. barbatus extract exhibited diarrhea inhibition activity. The percentage inhibition was dose-dependent with extract doses of 100, 200, and 400 mg/kgbw showing percentage inhibitions of 49.98±1.61, 66.12±2.17, and 75.80±2.16% respectively. Furthermore, as shown in Table 4, there was no significant variation in percentage inhibition of diarrhea at the dose of 200 mg/kgbw and the positive control (p<0.05).
Table 4. Effect of P. barbatus on castor oil-induced diarrhea in mice.
Effect of P. barbatus on gastrointestinal motility in mice
Findings from this study revealed that P. barbatus extract elicited a reduction in gastrointestinal motility in mice induced with diarrhea. At all doses, it was evident that the extract induced a significant decrease in the transit of charcoal meal in the gastrointestinal tract compared with the negative control (Figure 1; p<0.05). Moreover, as shown in Figure 1, it was observed that the reduction in intestinal transit of the charcoal meal by the extract dose of 200 mg/kgbw and loperamide (positive control) was statistically comparable (p > 0.05). Further, the dose of 400 mg/kgbw prevented the passage of the meal through the bowel and significantly (p<0.05) reduced the peristaltic index to 11.46±0.53 in comparison to the negative control (50.46±2.07).
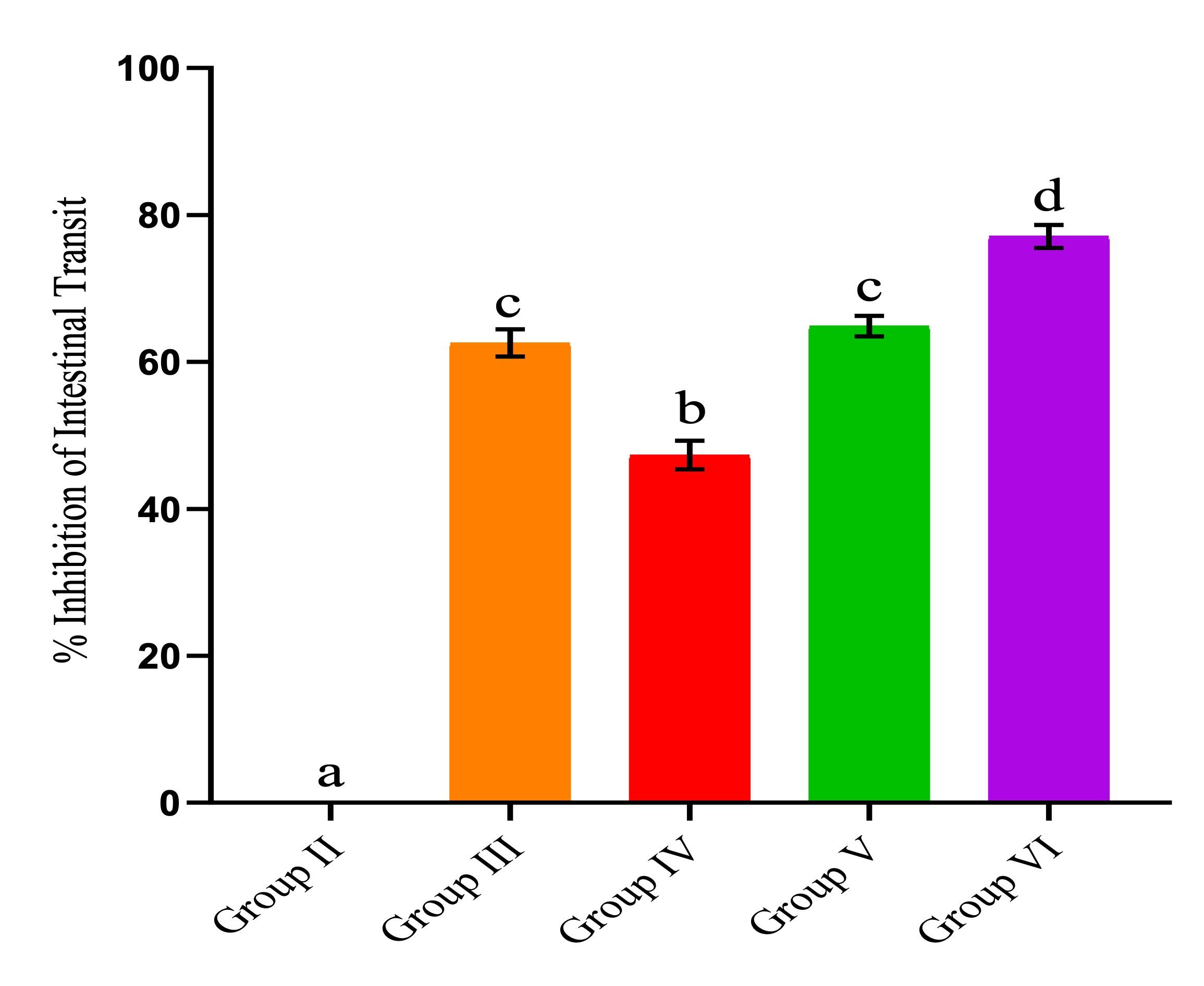
Effect of P. barbatus on enteropooling in mice
The extract hindered the buildup of castor oil-induced gastrointestinal fluid. The highest extract dosage (400 mg/kgbw) produced the highest percentage reduction. However, as depicted in Figure 2, it was evident that the effects of the extract dose of 200 mg/kgbw were significantly similar to those of loperamide (p > 0.05; Figure 2).
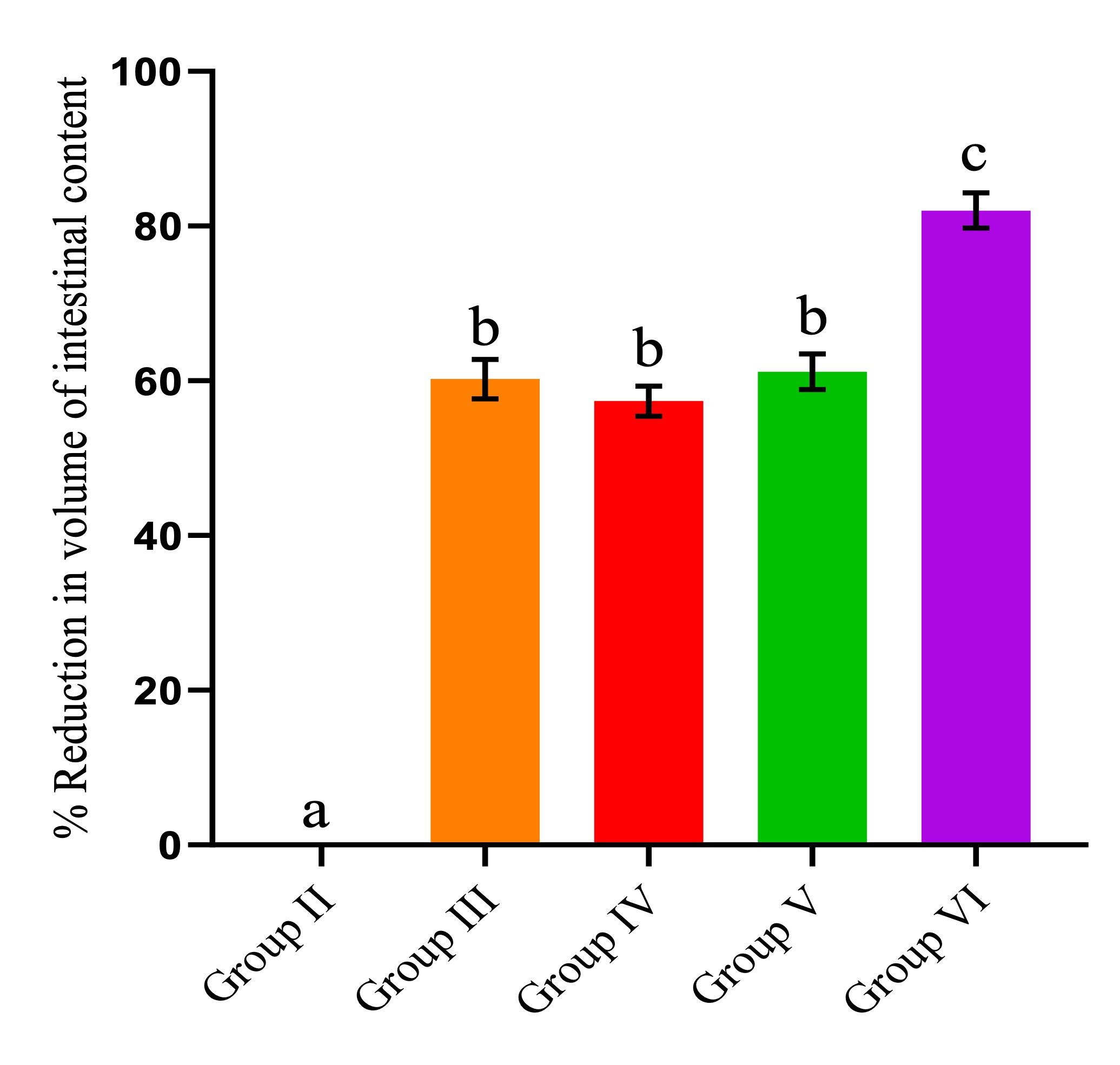
Effects of P. barbatus on anti-diarrheal index in mice
The anti-diarrheal effects of P. barbatus extract were also quantified in terms of the anti-diarrheal index (ADI). In vivo ADI parameters included delay in defecation, percentage inhibition of wet feces, and reduction of ileum contents. The extract demonstrated a remarkable dose-dependent increase in the ADI value of aqueous leaf extract of P. barbatus, with the maximum effect being detected at the highest concentration of the extract (p<0.05; Figure 3). The higher the ADI, the more effective the sample is at exerting antidiarrheal effects.
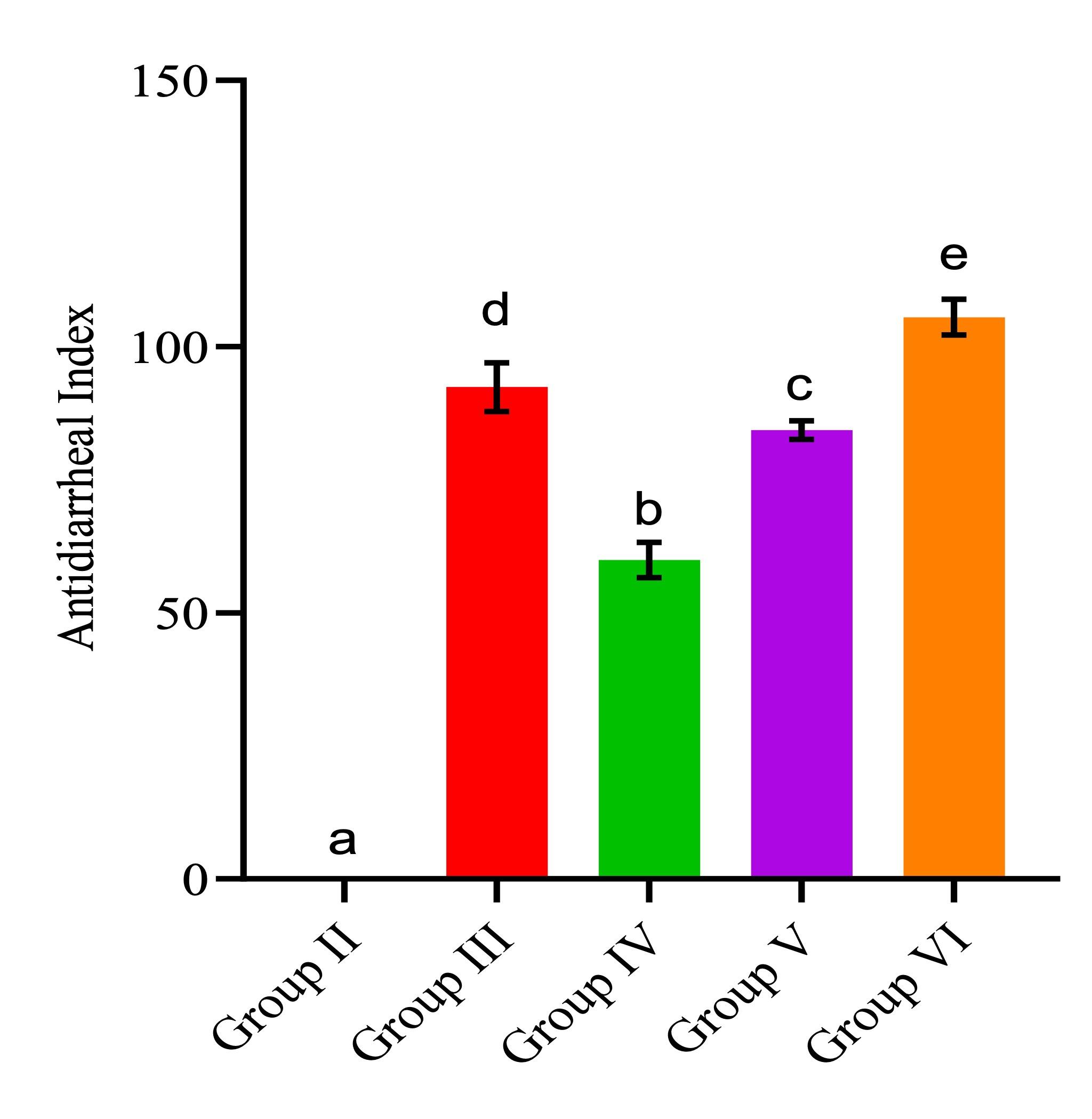
Effects of P. barbatus on acute oral toxicity in mice
The study included an acute oral toxicity test. There were no signs of evident toxicity or fatalities after 14 days of oral administration of P. barbatus extract. There were no changes in the mice's behavioral activities, movement frequency and nature, autonomic, neurological, or physical aspects. There were no observable signs of anxiety, coma, polyuria, seizures, skin and hair changes, ocular and mucous membrane alterations, salivation, piloerection, bradycardia, or tachycardia. Furthermore, there were no symptoms of vasodilation in the animals, such as redness of the skin, tongue, ear, or foot pad. The mean absolute organ weight and organ-body weight ratios for the treatment group (2000 mg/kgbw) and the normal control were calculated and compared. Orally administered P. barbatus aqueous leaf extracts at a dose of 2000 mg/kgbw did not reveal significant activity on the changes of body weight or organs to body weights compared to normal control mice, as shown in Figure 4.
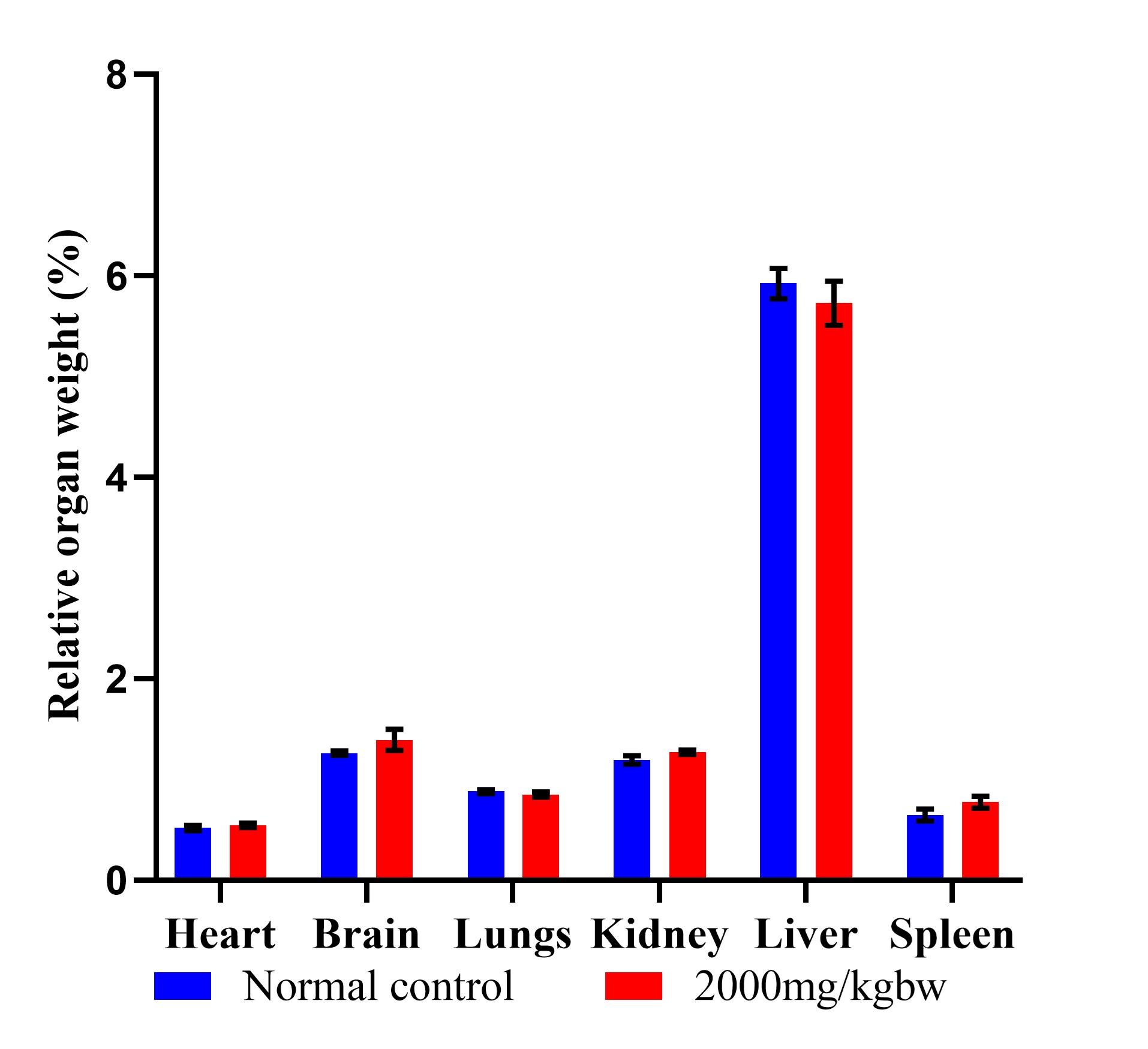
Quantitative phytochemical profile of P. barbatus
In this study, LC-MS analysis of P. barbatus extract identified 23 phytocompounds, viz., flavonoids, phenolics, and diterpenes (Table 5). The most abundant flavonoid was methoxy-kaempferol-3-glucuronide with a concentration of 1.34µg/mg, followed by luteolin-O-glucuronide (0.71µg/mg), methoxy-kaempferol-3-glucuronide (0.51µg/mg), and 4′-Methoxy-quercetin-3-O-glucuronide (0.31µg/mg). Vicenin-2 was the least abundant compound, with a concentration of 0.16 µg/mg.
The most abundant phenolic was rosmarinic acid (0.45µg/mg), followed by gallic acid with a concentration of 0.36µg/mg (Table 5). Several diterpenes were detected, including 3,6,12-Trihydroxy-2-acetyl-8,12-abietadien-7,11,14-trione (1.70µg/mg), 3,6,7,12,16-Pentahydroxy-2-acetyl-5,8,12-abietatrien-11,14-dione (0.43µg/mg), and forskolin (0.37µg/mg). The LC-MS spectrum of P. barbatus extract revealed detected phytocompounds with varying retention times, as depicted in Figure 5.
Table 5. Quantitative phytochemical profile of P. barbatus extract.
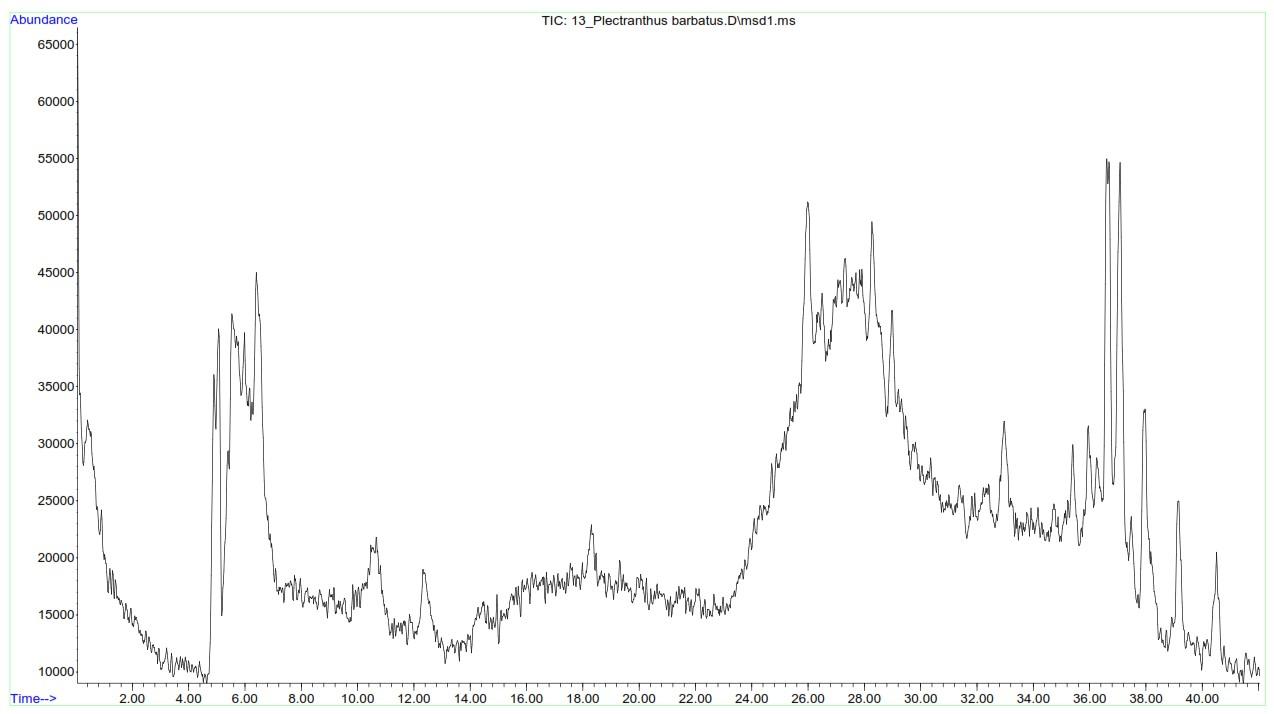
DISCUSSION
Diarrheal diseases remain a global health problem, and the mainstay conventional therapeutic strategies in use, despite showing effectiveness, are linked with several drawbacks. Herbal medicines are considered promising sources of novel therapeutic drugs owing to their affordability, minimal toxicity, and efficacy. This necessitates the need to pursue medicinal plants as potential antidiarrheal agents.
Findings from the study revealed that P. barbatus extract exhibited remarkable antidiarrheal effects. Additionally, the extract was found to be safe upon an acute oral toxicity test. Antidiarrheal activity of plant extracts has extensively been studied in previous studies; for instance, extracts of Sapium ellipticum, Plectranthus aegypticus, P. otostegiodes, P. lanuginose, Clutia abyssinica, and Clerodendrum myricoides have been documented to have potent antidiarrheal activity [20-23]. Additionally, preceding studies have established the antidiarrheal potency of aqueous plant extracts. For example, Lantana camara displayed antidiarrheal potential in castor oil-induced diarrhea in mice [13]. The antidiarrheal efficacy of the stem bark extract of Sapium ellipticum has also been reported [24]. Additionally, aqueous leaf extracts of Labisia pumila demonstrated growth inhibitory activity against selected microorganisms such as P. aeruginosa and E. coli [25]. Further, Hibiscus sabdariffa and Rosamarinus officinalis showed potent bactericidal effects on B. subtilis, S. aureus, and E. coli [26].
The antidiarrheal effect of P. barbatus was assessed through a castor oil-induced diarrheal model. The utilization of castor oil as a diarrheagenic agent has been documented [12, 27]. Castor oil induction of diarrhea has been shown to mimic the natural pathophysiology of diarrhea due to its laxative effect [23]. The effect is mediated by ricinoleic acid, a metabolite secreted by intestinal lipase. Additionally, the metabolite causes local inflammation and irritation of the stomach lining, which leads to the release of diarrheagenic mediators, including prostaglandins. Prostaglandins reduce intestinal fluid absorption by inhibiting Na+/K+-ATPase activity. It also increases the net secretion of intestinal electrolytes and water, gastrointestinal motility, and nitric oxide activation [10, 28]. Loperamide, the positive control used in this study, antagonizes the effects of ricinoleic acid, thereby reducing diarrhea [12].
An in vivo antidiarrheal assay was assessed using different parameters, including the commencement of diarrhea, frequency, and mass of wet feces in addition to the mass of total feces. Previous studies have validated the use of these parameters [12, 23]. Findings showed that an aqueous extract of P. barbatus delayed the onset of diarrhea and elicited a decrease in the rate of recurrence, mass of wet feces, and total fecal output in a concentration-dependent manner. The dose dependency of these parameters suggests that high doses of the extract are required to effectively inhibit diarrhea [10].
This study's findings are consistent with those previously reported on the methanol and aqueous leaf extracts of Cordia africana and Lantana camara Linn, respectively [12, 13]. Previous research has reported that medicinal plants with antidiarrheal effects prolonged diarrhea onset and reduced the frequency and mass of wet feces [23]. Root extracts of Clutia abyssinia and leaf extracts of Berssama abyssinica and Bixa orellana have been reported to have remarkable activity on the impediment of the onset of diarrhea and the reduction of its frequency. In addition, the extracts reduced the mass and frequency of wet feces [1, 22, 29].
P. barbatus extract was subjected to enteropooling and motility tests to further ascertain the mode of action [30]. The P. barbatus extract was found to reduce the accumulation of intraluminal fluid in the enteropooling assay. This could reduce distension, intestinal overload, and water content in fecal drops, resulting in less frequent defecation and diarrheal drops [31]. This could be attributed to ricinoleic acid's inhibition of prostaglandin E2 receptors, a mechanism consistent with loperamide's action [30]. Therefore, P. barbatus extract could have inhibited gastrointestinal enteropooling and hypersecretion by increasing facilitated electrolyte and intestinal water absorption [21].
Furthermore, P. barbatus extract inhibited the propulsion of the charcoal meal, thereby reducing intestinal transit and, eventually, diarrhea. The study’s finding implies that the P. barbatus extract can affect peristaltic movement in the lumen, indicating an antimotility effect [12, 32]. Protracted transit through the intestines also increases fluid absorption from feces. This causes the stool to become dry as it moves through the intestines [33]. In addition, the antimotility activities of P. barbatus extract could be related to mechanisms that influence inhibition of serotonergic and cholinergic activities, which are associated with gut motility [31].
In this regard, extracts of Clutia abyssinica and Myrtus communis have been shown to induce antidiarrheal effects in Swiss Albino mice by reducing gastrointestinal motility and stimulating the reabsorption of intestinal water and electrolytes [21]. Similarly, antimotility and anti-enteropooling activities of Hagenia abyssinica, Withania somnifera, and Cordia africana have been reported [12, 23, 31]. This study further revealed a high antidiarrheal index of the extract, an indication of its effectiveness to treat diarrhea [21, 34]. The findings are in concordance with those of Tadesse et al.[13], Degu et al. [21] and Desta et al. [23].
The antidiarrheal activities of P. barbatus can be attributed to a range of phytocompounds in the extract. In this study, flavonoids including 4-methoxy-quercetin-3-O-glucuronide, methoxy-kaempferol-3-glucuronide, 3,4-dimethoxy-luteolin-7-glucuronide, and luteolin-O-glucuronide were detected in the extract. Quercetin, as well as its derivatives, have been reported to reduce capillary permeability in the abdominal cavity and intestinal movement [35]. Additionally, quercetin inhibits the growth of pathogens that affect the gastrointestinal system, including E. coli. The bioactivity was achieved through various mechanisms, including disruption of the integrity of the bacterial cell membrane [36]. The antidiarrheic effect of quercetin could also be attributed to its antihistamine activities, which are related to inhibition of prostaglandin biosynthesis [37].
Additionally, methoxy-kaempferol-3-glucuronide and methoxy-kaempferol-7-glucuronide have been shown to have antimicrobial, anti-inflammatory, and antioxidant properties, which are associated with antidiarrheal effects [38]. Through their ability to complex bacterial cell walls, as well as soluble and extracellular proteins, these compounds have been shown to interfere with the growth of diarrhea-causing agents, including S. aureus [39].
The anti-inflammatory activities of the compounds aid in the inhibition of cyclooxygenase enzymes, which are required in the synthesis of prostaglandins [40, 41]. Additionally, previous studies show that flavonoids, including vicenin-2, reduce COX-2 expression by hindering the NF-ꓗB pathway. These activities stimulate protein kinase (AMPK), which suppresses pro-inflammatory signaling pathways, thereby increasing the absorption of electrolytes [41]. Moreover, flavonoids have been shown to confer antidiarrheal activities through their ability to hinder intestinal mucosa and hydro-electrolytic secretions [35, 42].
Phenolic compounds, including rosmarinic acid and gallic acid, exert anti-diarrheal activities through different mechanisms. The compounds have been demonstrated to reduce transit and intestinal secretion [10]. The compounds are also endowed with anti-inflammatory and analgesic properties associated with antidiarrheal activities [43].
As effective antimicrobial agents, phenolics inhibit the growth of pathogens associated with diarrheal diseases, including E. coli [44]. Phenolics, including gallic acid, have been demonstrated to exert their bactericidal activities by interrupting the integrity of the cell membrane [44]. Moreover, the compounds bind to bacterial DNA, subsequently inhibiting cellular functions such as replication, transcription, and expression, ultimately causing cell death. Their antioxidant activities have been demonstrated to protect the intestinal mucosa against castor oil [45]. In addition, antioxidant properties are arguably responsible for the observed inhibitory activities. Rosmarinic acid exerts its antibacterial effect by interfering with the membrane of the cytoplasm, thereby coagulating the cell contents. Kruk et al. [46] also suggested that these phenolic compounds interact with proteins, therefore generating a redox imbalance.
Diterpenoids, including forskolin, isolated from P. barbatus have been demonstrated to exhibit strong antibacterial and anti-spasmodic properties, pointing to their potential application in the care of digestive problems [47]. Previous studies have shown that forskolin stimulates adenylate cyclase, which increases the amount of the second messenger cyclic AMP in cells, thereby reducing histamine release, which in turn reduces inflammation and, ultimately, diarrheal episodes. Additionally, forskolin's antibacterial action was previously shown to be demonstrated by its inhibitory effects on the bacterial galactose-H+ transport protein, which interfered with the survival and pathogenicity of E. coli [48].
In this study, P. barbatus extract was found to be safe upon an acute oral toxicity test. Findings revealed no changes in the mice's behavioral activities or movement frequency. In addition, there were no clinical signs of anxiety, coma, polyuria, seizures, skin and hair changes, ocular and mucous membrane alterations, salivation, or piloerection. Moreover, oral administration of P. barbatus to mice had no effect on the weights of the body and organs. The present findings agree with those of Ezeonwumelu et al. [49], who revealed that the aqueous extracts of P. barbatus were safe up to a dosage level of 10,000 mg/kg. Similarly, Mwitari et al. [50] and Cordeiro et al. [51] demonstrated that P. barbatus extracts exhibited no toxicity to normal cells.
CONCLUSIONS
Findings from the current study revealed that the aqueous leaf extract of P. barbatus has a remarkable antidiarrheal effect attributable to the presence of bioactive compounds. In addition, the extracts were considered safe at the dosage level of 2,000 mg/kg. The study, therefore, offers scientific validation for the folklore utilization of P. barbatus in the management of diarrhea. However, further studies should be conducted to explore the mechanistic approach to the reduction of diarrhea and the comprehensive chronic toxicological effects on biochemical and hematological parameters.
ACKNOWLEDGEMENT
We acknowledge Kenyatta University and Uzima University for providing the resources and support for this research.
AUTHOR CONTRIBUTIONS
EA conceived, presented the idea and carried out the study. MP designed the study, supervised data analysis and verified the findings of this study. GO supervised and verified the findings of the study. CM analyzed the data and contributed to the drafting of the manuscript. All authors discussed the results and contributed to the final manuscript.
CONFLICTS OF INTEREST
There is no conflict of interest among the authors.
References
- [1]Ayalew M, Bekele A, et al. Evaluation of the antidiarrheal activity of 80% methanol extract and solvent fractions of the leaf of Bersama abyssinica fresen (Melianthaceae) in mice. BMC Complementary Medicine and Therapies. 2022;2(1):1–9.
- [2]Abdul- Mutalib NN, Sakai K, An overview of foodborne illness and food safety in Malaysia. International Food Research Journal.2015; 22(3):896.
- [3]Zhang SX, Zhou YM, et al. Impact of Coinfections with Enteric pathogens on Children Suffering from Acute Diarrhea in Southwest China. Infect. Dis. Poverty. 2016; 5(1):64.
- [4]Ashbolt NJ, Microbial contamination of drinking water and human health from community water systems. Current Environment Health Report. 2015; 2(1):95-106
- [5]Breurec S, Vanel N, et al. Etiology and Epidemiology of Diarrhea in Hospitalized Children from Low Income Country: A Matched Case-Control Study in Central African Republic. PLoS Neglected Tropical Diseases.2016;10(1):1–8.
- [6]Iijima Y, Oundo JO, et al. High Prevalence of Diarrheagenic Escherichia coli among Children with Diarrhea in Kenya. Japanese Journal of Infectious Diseases.2017; 70(1), 80–83.
- [7]World Health Organization (WHO) (2019). Drinking water 2019.
- [8]Li XY, Xia ST, et al. Gut microbiota and diarrhea: an updated review,”. Frontiers in Cellular and Infection Microbiology. 2021;11.
- [9]Silverman M, Konnikova L, et al. Impact of Antibiotics on Necrotizing Enterocolitis and Antibiotic-Associated Diarrhea. Gastroenterology Clinics of North America. 2017; 46(1):61–76.
- [10]Sisay M, Engidawork E, et al. Evaluation of the Antidiarrheal Activity of the Leaf Extracts of Myrtus Communis Linn (Myrtaceae) in Mice Model. BMC Complementary and Alternative Medicine. 2017;17(1):1–11.
- [11]Shifah F,Tareq A, et al. Antidiarrheal, Cytotoxic and Thrombolytic activities of methanolic extract of Hedychium coccineum leaves. J.Adv Biotechnol Exp Ther. 2020; 3(1): 77-78.
- [12]Ferede YA, Zewdu WS, et al. Evaluation of Antidiarrheal Activity of 80 % Methanolic Extract of the Leaves of Cordia africana (Lamiaceae) in Mice. Evidence-Based Complementary and Alternative Medicine. 2021;1–10.
- [13]Tadesse E, Engidawork E, et al. Evaluation of the Antidiarrheal Activity of the Aqueous Stem Extract of Lantana Camara Linn (Verbenaceae) in Mice. BMC Complementary and Alternative Medicine. 2017;17(190):1–8.
- [14]Alasbahi RH, Melzig MF, Plectranthus barbatus: A review of phytochemistry, ethnobotanical uses and pharmacology part 1. Planta Medica. 2010;76(7):653–659.
- [15]Lukhoba CW, Simmonds MSJ, et al. Plectranthus: A review of ethnobotanical uses. Journal of Ethnopharmacology.2006; 103:1–24.
- [16]NENT (2018). National Committee for Research Ethics in Science and Technology. National Ethics Research Committee
- [17]Abdela J, Evaluation of In Vivo Antidiarrheal Activities of Hydroalcoholic Leaf Extract of Dodonaea viscosa L .( Sapindaceae ) in Swiss Albino Mice. Journal of Evidence-Based Integrative Medicine.2019;24:1–10.
- [18]Teferi MY, Abdulwuhab M, et al. Evaluation of In Vivo Antidiarrheal Activity of 80% Methanolic Leaf Extract of Osyris quadripartita Decne (Santalaceae) in Swiss Albino Mice. Journal of Evidence-Based Integrative Medicine.2019;24.
- [19]OECD (2008). Guidelines for the Testing of Chemicals.
- [20]Musila FM, Nguta JM, et al. Antibacterial and antifungal activities of 10 Kenyan Plectranthus species in the Coleus clade. Journal of Pharmacy Research. 2017;11(8):1–7.
- [21]Degu A, Kefale B, et al. Evaluation of the Antidiarrheal Activity of Hydromethanol Crude Extracts of Ruta chalepensis and Vernonia amygdalina in Mice. Evidence-Based Complementary and Alternative Medicine. 2020;67-75.
- [22]Zayede D, Mulaw T, et al. Antidiarrheal Activity of Hydromethanolic Root Extract and Solvent Fractions of Clutia abyssinica Jaub. & Spach. (Euphorbiaceae) in Mice. Evidence Based Complementary and Alternative Medicine.2020;45-53.
- [23]Desta GT, Adela Alemu M, et al. Antidiarrheal Effect of 80% Methanol Extract and Fractions of Clerodendrum myricoides (Hochst.) Vatke (Lamiaceae) Leaf in Swiss Albino Mice. Evidence-Based Complementary and Alternative Medicine. 2021;1-6.
- [24]Wansi SL, Nguelefack-Mbuyo EP, et al. Antidiarrheal Activity of Aqueous Extract of the Stem Bark of Sapium ellipticum (Euphorbiaceae). Tropical Journal of Pharmaceutical Research. 2014;13(6):929–935.
- [25]Karimi E, Ze Jaafar H, et al. Fatty acid composition, antioxidant and antibacterial properties of the microwave aqueous extract of three varieties of Labisia pumila Benth. Biological Research. 2015;48:1–6.
- [26]Gonelimali FD, Lin J, et al. Antimicrobial properties and mechanism of action of some plant extracts against food pathogens and spoilage microorganisms. Frontiers in Microbiology. 2018;9:1–9.
- [27]Ahmadu AA, Zezi AU, et al. Anti-diarrheal activity of the leaf extracts of Daniellia Oliveri hutch and Dalz (Fabaceae) and ficus sycomorus Miq (Moraceae). African Journal of Traditional, Complementary and Alternative Medicines. 2007;4(4):524–528.
- [28]Usman AM, Danjuma NM, et al. Antidiarrheal Potentials of Methanol Bark Extract of Hymenocardia Acida Tul (Euphorbiaceae) in Laboratory Animals. Bulletin of the National Research Centre. 2021;45(1):1–12.
- [29]Tagne M, Akaou H, et al. Effect of the Hydroethanolic Extract of Bixa Orellana Linn (Bixaceae) Leaves on Castor Oil-Induced Diarrhea in Swiss Albino Mice. Gastroenterology Research and Practice. 2019;1:1-10.
- [30]Mekonnen B, Asrie AB, et al. Antidiarrheal Activity of 80% Methanolic Leaf Extract of Justicia schimperiana. Evidence-Based Complementary and Alternative Medicine. 2018;3:1-12.
- [31]Amabeoku G, Antidiarrheal activity of Geranium incanum Burm. f. (Geraniaceae) leaf aqueous extract in mice. Journal of Ethnopharmacology.2009; 123(1): 190–193.
- [32]Asrie AB, Abdelwuhab M, et al. Antidiarrheal activity of methanolic extract of the root bark of Cordia africana. Journal of Experimental Pharmacology. 2016; 8: 53–59.
- [33]Kifle ZD, Kidanu BB, et al. Evaluation of in Vivo Antidiarrheal Activity of Solvent Fractions of Hagenia abyssinica (Rosaceae) in Swiss Albino Mice. Evidence-Based Complementary and Alternative Medicine. 2021;3:50-56.
- [34]Woldeyohannes MG, Eshete GT, et al. Antidiarrheal and Antisecretory Effect of 80% Hydromethanolic Leaf Extract of Moringa stenopetala Baker f . in Mice. Evidence Based and Complementary Medicine, 2022;450-457.
- [35]Xiao X, Shi D, et al. Quercetin Suppresses Cyclooxygenase-2-expression and Angiogenesis through Inactivation of P300 Signaling. PLOS ONE. 2011;6:8.
- [36]Nguyen TLA, Bhattacharya D, Antimicrobial Activity of Quercetin: An Approach to Its Mechanistic Principle. Molecules. 2022;27:8.
- [37]Yadav AK, Tangpu V, Anticestodal Activity of Adhatoda vasica Extract Against Hymenolepis diminuta Infections in Rats. Journal of Ethnopharmacology.2008;119(2): 322–324
- [38]Mbaveng AT, Zhao Q, et al. 20 - Harmful and Protective Effects of Phenolic Compounds from African Medicinal Plants. Toxicological Survey of African Medicinal Plants, Elsevier.2014;577–609.
- [39]Konaté K, Yomalan K, Sytar O, et al. Antidiarrheal and antimicrobial profiles extracts of the leaves from Trichilia emetica Vahl. (Meliaceae). Asian Pacific Journal of Tropical Biomedicine. 2015;5(3):242–248.
- [40]El Sayed AM, Basam SM, et al. LC–MS/MS and GC–MS profiling as well as the antimicrobial effect of leaves of selected Yucca species introduced to Egypt. Scientific Reports. 2020; 10(1):1–15.
- [41]Tutunchi H, Naeini F, et al. Naringenin, a Flavanone with Antiviral and Anti-inflammatory Effects: A Promising Treatment Strategy against COVID-19. Phytotherapy Research. 2020;3137–3147.
- [42]Alexander D, Arulmoli R, et al. Overview of Biological Importance of Quercetin: A Bioactive Flavonoid. Pharmacognosy Reviews. 2016;10(20):84.
- [43]Pei K, Ou J, et al. p-Coumaric acid and its conjugates: Dietary sources, pharmacokinetic properties and biological activities. Journal of the Science of Food and Agriculture. 2016; 96(9): 2952–2962.
- [44]Su X, Howell AB, et al. Antiviral Effects of Cranberry Juice and Cranberry Proanthocyanidins on Foodborne Viral Surrogates – A Time Dependence Study In Vitro. Elsevier-Food Microbiology.2010; 27(8): 985–991.
- [45]Lou Z, Wang H, et al. p-Coumaric acid kills bacteria through dual damage mechanisms. Food Control.2012;25(2):550–554.
- [46]Kruk J, Aboul-Enein BH, et al. Antioxidative properties of phenolic compounds and their effect on oxidative stress induced by severe physical exercise. J Physiol Sci. 2022; 72:19.
- [47]Patel M, Forskolin: A successful therapeutic phytomolecule. East and Central Africa Journal of Pharmaceutical Science.2010; 13: 25-32.
- [48]Salehi B, Staniak M, et al. The Therapeutic Potential of the Labdane Diterpenoid Forskolin. Applied Sciences.2019; 9(19):4089.
- [49]Ezeonwumelu J, Kawooya G, et al. Phytochemical Screening, Toxicity, Analgesic and Anti-pyretic Studies of Aqueous Leaf Extract of Plectranthus barbatus (Andrews. Engl.) in Rats. Pharmacology and Pharmacy.2019; 10: 205-2011.
- [50]Mwitari P, Ayeka P, et al. Antimicrobial Activity and Probable Mechanisms of Action of Medicinal Plants of Kenya: Withania somnifera, Warbugia ugandensis, Prunis Africana and Plecranthus barbatus. PLOS. 2013; 8 (6): 1-9.
- [51]Cordeiro MF, Nunes TRS, et al. Phytochemical characterization and biological activities of Plecranthus barbatus andrews. Brazilian Journal of Biology. 2022;82:1–12.