Glutathione: A key frontier of heavy-metal detoxification and tolerance in plants
Abstract
Heavy metal (HMs) toxicity is one of the major critical threats to agricultural plant production and global food security. In this current scenario, eco-friendly and hazardous-free sustainable crop production strategies for detoxifying and tolerating HMs are imperative. This updated study provides the impact of HMs on agricultural plants' growth, physiology, and yield. Further, this study explores the significance of glutathione (GSH) and phytochelatins (PCs) and a series of candidate genes that showed potential role in HMs stress tolerance in diverse plant species. This updated study encourages plant breeders or farmers to develop HMs stress-tolerant plant production through a breeding program. The overall study findings open new avenues of multiomics-assisted plant improvement, and it will help phytoremediation, a clean environment, and smart agriculture for sustainable plant production.
INTRODUCTION
The contamination of agricultural soils with heavy metals (HMs) is one of the serious limitations for plant life, food safety, and human health in the world [1]. The rapid industrialization and urbanization processes are gradually increasing the load of metal and metalloids such as Cd, Pb, Cr, As, Hg, Ni, Cu, Zn, and Co to agricultural soils [2]. In addition, the application of phosphate fertilizers, excess industrial chemical waste, and sewage sludge are actively involved in increasing HMs-toxicity to plants, soils, and environments [3, 4]. HMs toxicity severely inhibit plant growth and alters a series of physiological and molecular changes in different plant species [5-8]. These limitations in plants ultimately lead to a decline in plant biomass yield and productivity. Therefore, updated understating with eco-friendly and cost-effective strategies are highly demandable for sustainable plant production, agricultural soil health, and ensuring toxicant-free global food security.
HMs induce excess reactive oxygen (ROS) species that lead to oxidative stress-induced cellular toxicity, and lipid peroxidation in plant cells [7]. The excess production of ROS and subsequent induction of oxidative stress are sequential consequences in plants. Plants have evolved several mechanisms to combat these toxic pollutants through antioxidant defense systems [9, 10]. In this defense system, plant induces several enzymatic and non-enzymatic antioxidants, which play key roles in detoxifying and/or mitigating tolerance in HMs in plants [6, 10]. Plants produce also low molecular weight thiols, which possess a high affinity to HMs [10]. One of the key frontiers of thiols is glutathione (GSH) [2]. The synthesis of GSH occurs by the consecutive involvement of enzymes γ-glutamylcysteine synthetase and glutathione synthetase [2]. The GSH (gamma-glu-cys-gly) presents with a high level in living cells and is a major source of non-protein-reduced sulfur (S). The GSH accelerates bio-reductive reactions that lead to enhanced defense against HMs or any other foreign toxic chemicals [11]. The regulation of the S assimilation process is crucial for the adequate supply of S-compounds important for HMs-detoxification and tolerance in plants [10]. S presence in several components including amino acids cysteine, methionine, oligopeptides (GSH, phytochelatins), vitamins, and secondary products [12]. The thiols cysteine and GSH are involved in the redox process through a disulfide conversion system that leads to the homeostasis of oxidative stress in plants [13]. The particular GSH plays a key role in detoxifying HMs through coordination with the −SH group that is mediated by GSH-S-transferase. In addition, the phytochelatins (PCs) are a polymerized version of GSH involved in HMs detoxification by functioning as chelating ligands [6].
This current review explores the toxic impact of HMs in plants and highlights the recent progress of GSH potential in HMs detoxification and tolerance in diverse plant species. This study further suggests the involvement of various candidate genes in HMs tolerance processes and their prospects in developing HMs-tolerant genotypes through breeding programs.
GLUTATHIONE BIOSYNTHESIS IN PLANTS
The chloroplast, mitochondria, plastid, and cytosol are the key locations for GSH synthesis [14]. GSH is synthesized through two consecutive enzymatic reactions whereas ATP is dependent. In the first step, enzyme γ-ECS catalyzes a bond between γ-COOH of Glu and the α-NH2 group of Cys (Figure 1). In the second step, the GS leads the reaction to bond formation cysteinyl-COOH of γ-GC and α-NH2 group of Gly, subsequently, the GSH is synthesized from γ-GGC (Figure 1). Hence, the number of ATP is reduced in every reaction, synthesis of γ-GC can act as a rate-limiting factor for GSH synthesis, and the γ-ECS activity depends on Cys-availability. Interestingly, the addition of glutathione S-transferase (GST) to GSH leads to the conjugation process of toxic metals/xenobiotics and transports those metals into the vacuole (Figure 1).
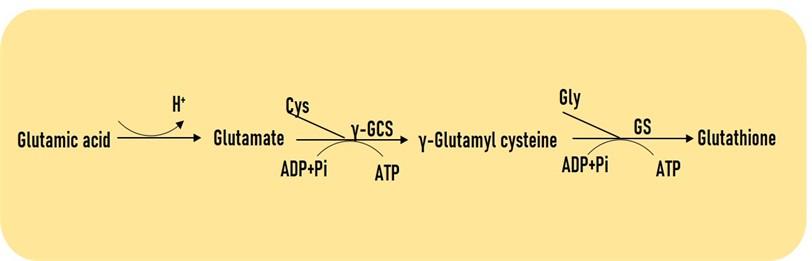
IMPACT OF HEAVY METAL TOXICITY IN PLANTS
Several metals/metalloids such as Cd, Pb, Cr, As, Hg, Ni, Cu, and Zn cause toxicity in plants [15]. Among them, Cd, Pb, As, Hg, and Cr show highly toxic effects on plant growth, development, and productivity [5, 6, 10, 15]. These toxic elements are involved in regulating a series of morpho-physiological and molecular alterations in several plant species. In the following sections, we discuss the updated findings on how different HMs negatively impact on different crop plants (Figure 2 and Table 1).
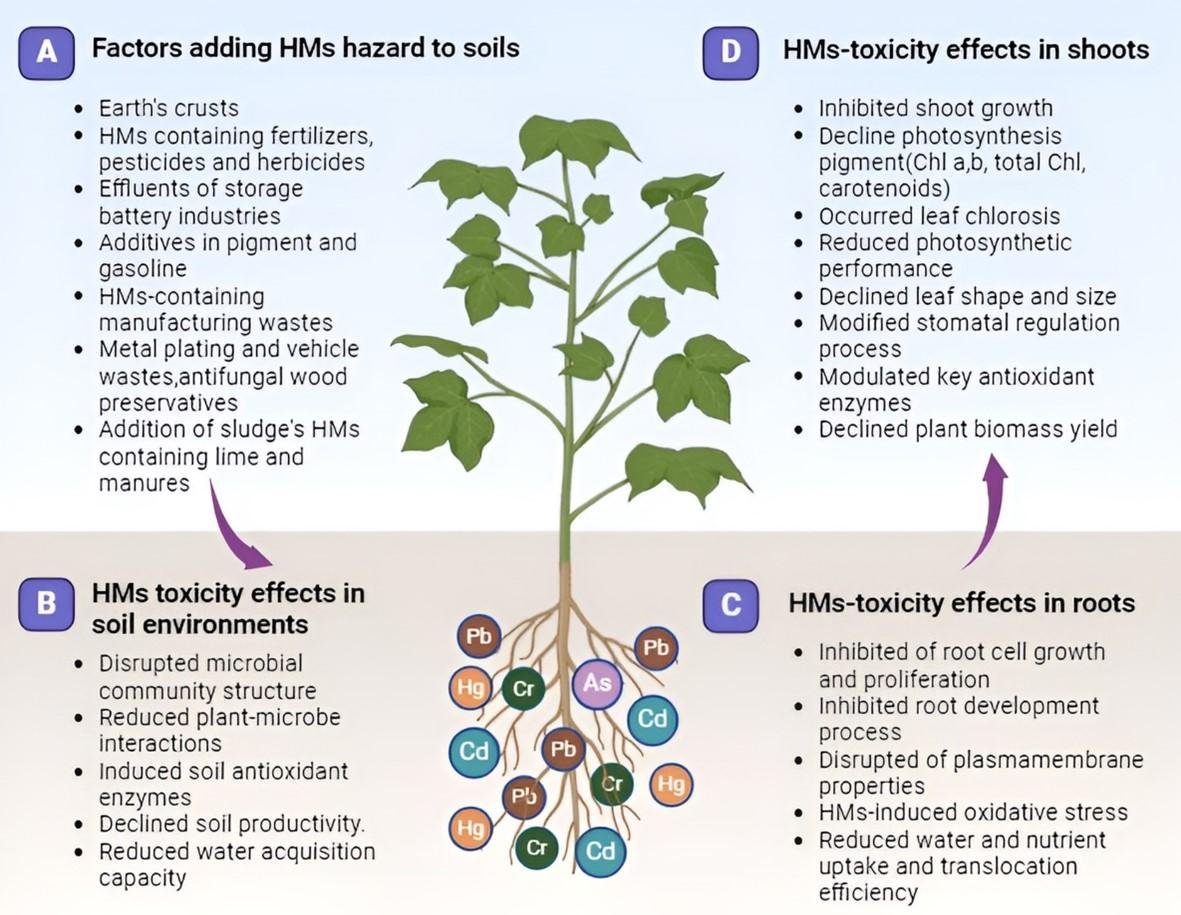
Table 1. Effects of HMs-toxicity on plant traits.
Cadmium toxicity
Cadmium (Cd) is one of the most toxic hazards exposed in industrial workplaces, and agricultural plants and soils due to frequent use of Cd-containing fertilizers, pesticides, and herbicides (Figure 2) [16]. The threshold level of Cd toxicity for plants ranges from 3–30 mg kg−1 Cd concentration in plant tissue exceeds [17]. The Cd toxicity causes chlorosis, growth and photosynthetic inhibition, and biomass yield reduction in alfalfa [6]. Haque et al found Cd-toxicity triggers plant growth retardation, chlorosis, low photosynthesis performance, and low Fe, S, and Zn concentrations in sugar beets, which were related to decline FCR activity and BvIRT1 gene expression, suggesting the negative effect of Cd-toxicity on Fe acquisition process in sugar beet [7]. In this same study, the candidate genes BvHMA3 and BvNRAMP3 showed upregulation patterns in response to Cd-toxicity, suggesting that these candidate genes are involved in Cd uptake processes. Mittra et al explored Cd-induced alterations physiological and proteomic alterations in Brassica, where Cd-severely declined plant growth, occurred leaf chlorosis and induced a series of candidate proteins involved in antioxidant defense system and sulfur assimilation-involving Cd-detoxification processes in Brassica [5]. Cd-toxicity not only changes the morpho-physiological and molecular attributes but also these impairments lead to a decline in biomass yield and productivity in plants (Table 1). For instance, Kabir et al stated that Cd (10 μM CdSO4) significantly declines elemental concentration, photosynthetic performance, and biomass yield in sugar beet [9]. Cd-toxicity (1 mg L−1) severely impairs mineral acquisition and yield traits in wheat at the seedling stage, exploring the toxic consequence of Cd at different developmental stages in plants [18]. Recently, Sana et al found genotypic differences in Chili plants in response to several doses of treatment Cd (3-5 mg Cd kg− 1) in soils, where Cd-caused growth retardation, reduced sugars, proteins, and amino acids concentrations, along with biomass yield reduction [16]. The above investigations suggest that Cd-toxicity can vary based on Cd-doses and/or Cd load to the exposed plants, wherein a very low concentration of Cd can be toxic and detrimentally influence plant developmental processes and productivity.
Lead toxicity
Lead (Pb) toxicity is considered as the second most hazardous element after As, which comprises 0.002% of Earth's crust and it does not show any potential benefit in biological systems [19]. The additives in pigment and gasoline, effluents of storage battery industries, melting and manufacturing factories, activities related to metal plating, and vehicle wastes are the potential sources for adding Pb to soils and environments (Figure 2) [19]. Pb toxicity causes cellular injury and damage in plants from growing to mature stages. A series of morphological, physiological, biochemical, and molecular alterations occur in plants under Pb toxicity [20]. Pb-toxicity considerably changes the photosynthetic apparatus and declines chlorophyll pigment content leading to a decline in carbon metabolism efficiency in plants [19]. The crop yield component and productivity significantly declined due to Pb toxicity in different rice genotypes [21], where yield reduction was 46.27% to 69.12%. Weekly 25 μg kg−1 Pb exposure with human body weight is considered the threshold level [22]. Pb has no beneficial significance in plants, soils, and environments. Recently, Pb toxicity severely exposed to environments from the effluents of storage batteries. The translocation of toxic Pb from the environment to crop grains [19], indicates that Pb toxicity rapidly spreads from the environment to food chains. Pb toxicity is not only harmful to plants and soils but also significantly impairs soil microbial community structures [23].
Arsenic toxicity
Arsenic (As) is naturally producing metalloids that are nonessential for the plant developmental process. As is toxic for plants, humans, and the environment. Soil and water are the major sources of As hazard. As is a naturally occurring poison universally present in the Earth's crust, and is used in antifungal wood preservation processes, as an element of industrial manufacture, pesticides, and fireworks materials (Figure 2) [24]. The root is the first sensing organ that is initially affected by As exposure [25]. As uptake and transportation several plant organs significantly inhibit plant growth, development, and total biomass yield [26]. As toxicity alters the plant metabolism process it leads to inhibition of cell proliferation and even plant death [27]. The process of symbiosis is crucial for biological ‘N2’ fixation and is an alternative source of ‘N2’ source in plants. Unfortunately, toxicity negatively affects rhizobium bacteria, root nodule formation, and the ultimate naturally plant-microbe-based N supply system [25]. Recently, it has been reported that As toxicity declines grain yields up to 39% in rice.
Mercury toxicity
Mercury (Hg) is a toxic element. Hg hazard added to the environment through volcanic eruption, fuels or raw materials of batteries, electric materials, sludges, fertilizers, lime, and manures (Figure 2) [28, 29]. Hg exposure levels even at low concentrations (1.0 and 1.5 mg/L) can be toxic for plants that lead to disruption of cellular function, plant growth, and development [30]. Though the specific role of Hg in plant biological functions is still unclear [31], but it can cause trouble in plant physiological processes. El-Shehawi et al found that Hg causes sulfur (S) and iron (Fe) deficiency which induces oxidative stress in alfalfa [4]. The Hg toxicity (1.0 and 2.5 mg L−1) declined root biomass yield by 50%, while shoot biomass declined by 50% at 0.5 mg L−1 Hg level [32].
Chromium toxicity
Chromium (Cr) is a non-essential toxic metal. Cr is added to the environment from the waste of leather factories, electroplating, paints, and dye industries, municipal trash, and uses of Cr-containing fertilizer [33]. Plants uptake Cr from soil contaminants that lead to a series of physiological and molecular alterations in plants [34]. Basit et al. [35] reported that excess accumulation of Cr significantly declines seed germination rate and plant growth which leads to plant biomass yield. Some other consecutive studies suggest that Cr toxicity considerately reduces photosynthetic pigments (Chl a, b, and total chl) content, which significantly reduces plant photosynthesis efficiency [33]. Excess accumulation of Cr negatively influences the activity of the cell cycle and causes an imbalance of elemental concentration, enzyme activity, and other metabolic processes, which leads to the induced generation of reactive oxygen species and oxidative stress in several plant species [36]. Recently, Saud et al [37], found that Cr toxicity declines seed germination rate, photosynthesis, plant growth, and yield. The shoot (38%) and root (37%) length of green gram declined in Cr toxicity treated Vigna radiate compared to control plants [38].
GLUTATHIONE-INVOLVING HEAVY METAL DETOXIFICATION AND TOLERANCE IN PLANTS
Glutathione (GSH) is an excellent source of thiol in cells that are located in several cellular organelles such as vacuole (VC), mitochondria (Mt), chloroplast (ChP), and endoplasmic reticulum (EPR). The thiol group of GSH forms a bond with HMs. GSH is involved in the HMs detoxification process by mitigating the H2O2 level in the cells. During the HMs-induced ROS scavenging system the GSH is changed to its oxidized form (GSSG) which is one of the key processes of the redox signaling process (Figure 3). GSH plays as role of antioxidant and is involved in ROS homeostasis and detoxification processes foreign toxic metals (xenobiotics including major HMs) that lead to enhanced HMs tolerance in plant cells (Figure 3 and Table 2). The toxic metal and xenobiotic conjugate with GSH by the activity of glutathione S-transferase (GST), and subsequently transport metals to VC. Another process is chelation, where GSH plays a role as a precursor of phytochelatins (PCs) synthesis. In another process enzyme PCS coordinates the binding process of thiol group and PCs.
GSH supplementation enhances HM's stress tolerance through the regulation of antioxidant systems [44]. Several studies have indicated that exogenous supplementation of GSH enhances antioxidant enzyme activity that helps to enhance HMs toxicity tolerance in plants. Daud et al. [45], found GSH supplementation (50 μM) declines leaf Cd content and increases SOD, APX, POD, and GR activity in Mexican cotton. Khan et al. [46], reported that exogenous application of GSH (50 μM) mitigates Pb-induced physiological and metabolic impairments by reducing oxidative stress markers (MDA and H2O2) levels and increasing SOD, CAT, POD, APX, and GR activity in Gossypium hirsutum.
The potential of GSH-derived PCS and its biosynthesis-related genes have a pivotal role in HM stress tolerance in a series of plant species. The PCs are synthesized by the phytochelatins synthase (PCS) gene that responds under HMs stress. Overexpression of BnPCS1 lines showed better growth and biomass yield, along with exhibiting higher Cd accumulation and translocation compared to WT, which led to Cd stress tolerance [47]. The PCS genes are well distributed in diverse plant species and AtPCS1, OsPCS1, TaPCS1, and BjPCS1 are well characterized in Arabidopsis, rice, and wheat [2]. These genes are involved in diverse metal toxicity tolerance (Table 2). Exogenous supplementation of sulfur was reported to enhance GSH concentration along with their corresponding genes MsGS and MsPCS1 in roots, which leads to Cd toxicity tolerance in alfalfa plants [6]. Recently, Rahman et al. [10], found that GSH triggers the PC accumulation, and Hg responsive upregulation of the MsPCS1 gene helps to enhance Cd tolerance in alfalfa. With the above advancement of GSH-related research in HMs toxicity stress it is clear that GSH is potentially involved in HMs detoxification and tolerance processes, which lead to enhanced endogenous GSH and PC biosynthesis and plant fitness under diverse HMs stress.
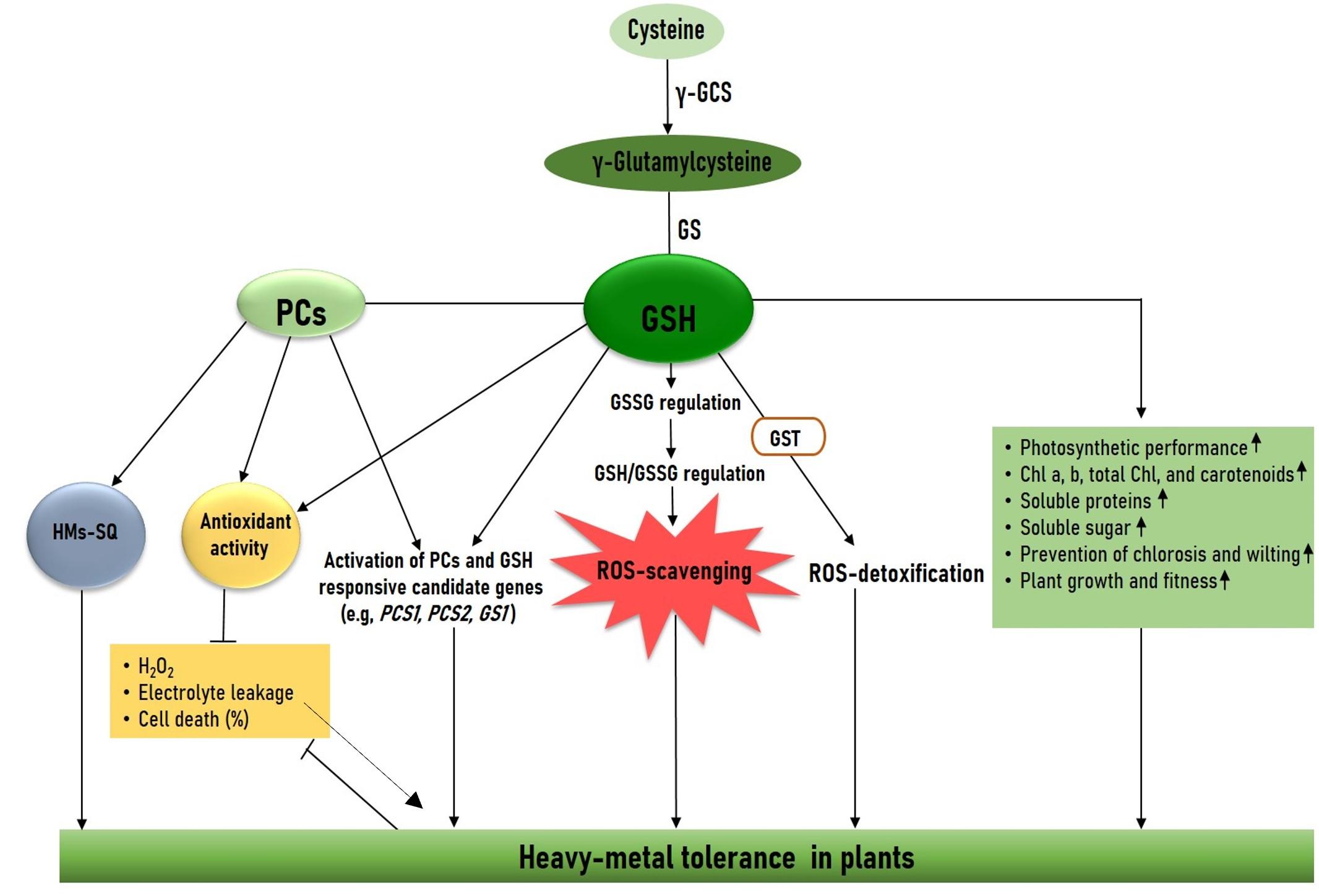
Table 2. Glutathione and phytochelatins responsive key genes involved in HMs detoxification and tolerance in plants.
CONCLUSION AND FURTHER PROSPECTS
HMs toxicity is now a global problem for agricultural plants, soils, the environment, and human health. Therefore, eco-friendly, and cost-effective strategies of HMs toxicity mitigation and stress tolerance are highly demandable. This study provides an updated understanding of major heavy metal toxicity impacts on agricultural plants. Further, this study suggests the potential role of GSH and PCs and their corresponding candidate genes which help to mitigate and tolerate distinct heavy metals in plants. A glutathione-based heavy metal mitigation approach proves effective by harnessing the natural detoxification properties of glutathione. By cultivating crops rich in glutathione or its precursors, such as certain members of the Brassicaceae family, the plants actively absorb heavy metals from the soil. Glutathione, present within the plant cells, binds to the heavy metals, facilitating their sequestration and subsequent transport to vacuoles for storage or root exudation. Additionally, fostering plant-microbe interactions, particularly with mycorrhizal fungi, enhances glutathione production, contributing to an increased capacity for heavy metal detoxification. This update study might be helpful to plant breeders or farmers for enhancing HMs stress tolerance in diverse crop plants under toxic environments. However, with the progress of the study GSH-induced regulation of candidate genes, proteins, and metabolites have not been well established. Therefore, multiomics-assisted agriculture crop improvement would be a promising area of research along will open a new avenue of HMs-tolerant crop production for smart and sustainable plant production for global food security.
ACKNOWLEDGEMENT
None.
AUTHORS CONTRIBUTION
SKR conceived the research plan and wrote the manuscript. MN and FR supported to figure drawing and edited the manuscript. All the authors approved the final version of the manuscript.
CONFLICTS OF INTEREST
There is no conflict of interest among the authors.
References
- [1]Zhou H, Chen Y, et al. Identification and hazard analysis of heavy metal sources in agricultural soils in ancient mining areas: A quantitative method based on the receptor model and risk assessment. J Hazard Mater. 2023;445:130528.
- [2]Yadav SK. Heavy metals toxicity in plants: An overview on the role of glutathione and phytochelatins in heavy metal stress tolerance of plants. S Afr J Bot. 2010;76:167-79.
- [3]Mbarki S, Talbi O, et al. Comparison of grain sorghum and alfalfa for providing heavy metal remediation of sandy soil with different soil amendments and salt stress. Front Environ Sci. 2022;10.
- [4]El-Shehawi AM, Rahman MA, et al. Mercury toxicity causes iron and sulfur deficiencies along with oxidative injuries in alfalfa (medicago sativa). Plant Biosyst. 2022;156:284-91.
- [5]Mittra PK, Roy SK, et al. Proteome insights of citric acid-mediated cadmium toxicity tolerance in brassica napus l. Environ Sci Pollut Res. 2023.
- [6]Das U, Rahman MA, et al. Sulfur triggers glutathione and phytochelatin accumulation causing excess cd bound to the cell wall of roots in alleviating cd-toxicity in alfalfa. Chemosphere. 2021;262:128361.
- [7]Haque AFMM, Tasnim J, et al. The cd-induced morphological and photosynthetic disruption is related to the reduced fe status and increased oxidative injuries in sugar beet. Plant Physiol Biochem. 2021;166:448-58.
- [8]Haque AFMM, Rahman MA, et al. Changes in physiological responses and mtp (metal tolerance protein) transcripts in soybean (glycine max) exposed to differential iron availability. Plant Physiology and Biochemistry. 2022;179:1-9.
- [9]Kabir AH, Das U, et al. Silicon induces metallochaperone-driven cadmium binding to the cell wall and restores redox status through elevated glutathione in cd-stressed sugar beet. Physiol Plant. 2021;173:352-68.
- [10]Rahman MA, Kabir AH, et al. Glutathione restores hg-induced morpho-physiological retardations by inducing phytochelatin and oxidative defense in alfalfa. Biology 2020;9.
- [11]Mendoza-Cózatl D, Loza-Tavera H, et al. Sulfur assimilation and glutathione metabolism under cadmium stress in yeast, protists and plants. FEMS Microbiol Rev. 2005;29:653-71.
- [12]Brosnan JT, Brosnan ME. The sulfur-containing amino acids: An overview. J Nutr. 2006;136:1636s-40s.
- [13]Saito K. Sulfur assimilatory metabolism. The long and smelling road. Plant Physiol. 2004;136:2443-50.
- [14]Gasperl A, Zellnig G, et al. Organelle-specific localization of glutathione in plants grown under different light intensities and spectra. Histochem Cell Biol 2022;158:213-27.
- [15]Rashid A, Schutte BJ, et al. Heavy metal contamination in agricultural soil: Environmental pollutants affecting crop health. Agronomy2023.
- [16]Sana S, Ramzan M, et al. Differential responses of chili varieties grown under cadmium stress. BMC Plant Biol. 2024;24:7.
- [17]Ismael MA, Elyamine AM, et al. Cadmium in plants: Uptake, toxicity, and its interactions with selenium fertilizers. Metallomics. 2019;11:255-77.
- [18]Zhang G, Fukami M, et al. Influence of cadmium on mineral concentrations and yield components in wheat genotypes differing in cd tolerance at seedling stage. Field Crops Res 2002;77:93-8.
- [19]Zulfiqar U, Farooq M, et al. Lead toxicity in plants: Impacts and remediation. J Environ Manage. 2019;250:109557.
- [20]Sharma P, Dubey RS. Lead toxicity in plants. Braz J Plant Physiol. 2005;17.
- [21]Ashraf U, Kanu AS, et al. Lead (pb) toxicity; physio-biochemical mechanisms, grain yield, quality, and pb distribution proportions in scented rice. Frontiers in plant science. 2017;8:259.
- [22]Fang Y, Sun X, et al. Concentrations and health risks of lead, cadmium, arsenic, and mercury in rice and edible mushrooms in china. Food Chem Toxicol. 2014;147:147-51.
- [23]Akmal M, Jianming XJ. Microbial biomass and bacterial community changes by pb contamination in acidic soil. J aagric biol sci. 2009;1.
- [24]Chung JY, Yu SD, et al. Environmental source of arsenic exposure. Journal of preventive medicine and public health = Yebang Uihakhoe chi. 2014;47:253-7.
- [25]Shukla SK, Mishra RK, et al. Land reformation using plant growth–promoting rhizobacteria in the context of heavy metal contamination. In: Ahmad P, editor. Plant metal interaction: Elsevier; 2016. p. 499-529.
- [26]Srivastava S, Sharma YK. Impact of arsenic toxicity on black gram and its amelioration using phosphate. ISRN toxicology. 2013;2013:340925.
- [27]Finnegan PM, Chen W. Arsenic toxicity: The effects on plant metabolism. Frontiers in physiology. 2012;3:182.
- [28]Edwards BA, Kushner DS, et al. Fifty years of volcanic mercury emission research: Knowledge gaps and future directions. Sci Total Environ. 2021;757:143800.
- [29]Azevedo R, Rodriguez E. Phytotoxicity of mercury in plants: A review. J Bot. 2012;2012:848614.
- [30]Kalinhoff C, Calderón NT. Mercury phytotoxicity and tolerance in three wild plants during germination and seedling development. Plants 2022;11.
- [31]Durand A, Maillard F, et al. Interactions between hg and soil microbes: Microbial diversity and mechanisms, with an emphasis on fungal processes. Applied microbiology and biotechnology. 2020;104:9855-76.
- [32]Du X, Zhu YG, et al. Uptake of mercury (hg) by seedlings of rice (oryza sativa l.) grown in solution culture and interactions with arsenate uptake. Environ Exp Bot. 2005;54:1-7.
- [33]Ali S, Mir RA, et al. Chromium toxicity in plants: Signaling, mitigation, and future perspectives. Plants (Basel, Switzerland). 2023;12.
- [34]Sharma A, Kapoor D, et al. Chromium bioaccumulation and its impacts on plants: An overview. Plants 2020;9.
- [35]Basit F, Akhter Bhat J, et al. Screening of rice cultivars for cr-stress response by using the parameters of seed germination, morpho-physiological and antioxidant analysis. Saudi J Biol Sci. 2022;29:3918-28.
- [36]Patra DK, Pradhan C, et al. Chromium bioaccumulation, oxidative stress metabolism and oil content in lemon grass cymbopogon flexuosus (nees ex steud.) w. Watson grown in chromium rich over burden soil of sukinda chromite mine, india. Chemosphere. 2019;218:1082-8.
- [37]Saud S, Wang D, et al. The impact of chromium ion stress on plant growth, developmental physiology, and molecular regulation. Front Plant Sci. 2022;13:994785.
- [38]Dheeba B, Sampathkumar P, et al. Fertilizers and mixed crop cultivation of chromium tolerant and sensitive plants under chromium toxicity. Journal of toxicology. 2015;2015:367217.
- [39]Zhao H, Guan J, et al. Effects of cadmium stress on growth and physiological characteristics of sassafras seedlings. Scientific Rep. 2021;11:9913.
- [40]Saeed M, Masood Quraishi U, et al. Identification of arsenic-tolerant varieties and candidate genes of tolerance in spring wheat (triticum aestivum l.). Chemosphere. 2022;308:136380.
- [41]Yuan H, Liu Q, et al. Sulfur nanoparticles improved plant growth and reduced mercury toxicity via mitigating the oxidative stress in brassica napus l. J Clean Prod. 2021;318:128589.
- [42]Sahu GK, Upadhyay S, et al. Mercury induced phytotoxicity and oxidative stress in wheat (triticum aestivum l.) plants. Physiology and molecular biology of plants : an international journal of functional plant biology. 2012;18:21-31.
- [43]Sharmin SA, Alam I, et al. Chromium-induced physiological and proteomic alterations in roots of miscanthus sinensis. Plant Sci. 2012;187:113-26.
- [44]Jozefczak M, Remans T, et al. Glutathione is a key player in metal-induced oxidative stress defenses. Int J Mol Sci. 2012;13:3145-75.
- [45]Daud MK, Mei L, et al. Leaf-based physiological, metabolic, and ultrastructural changes in cultivated cotton cultivars under cadmium stress mediated by glutathione. Environmental science and pollution research international. 2016;23:15551-64.
- [46]Khan M, Daud MK, et al. Alleviation of lead-induced physiological, metabolic, and ultramorphological changes in leaves of upland cotton through glutathione. Environmental science and pollution research international. 2016;23:8431-40.
- [47]Zhu S, Shi W, et al. Overexpression of bnpcs1, a novel phytochelatin synthase gene from ramie (boehmeria nivea), enhanced cd tolerance, accumulation, and translocation in arabidopsis thaliana. Frontiers in plant science. 2021;12:639189.
- [48]Tang M, Li R, et al. Exogenous glutathione can alleviate chromium toxicity in kenaf by activating antioxidant system and regulating DNA methylation. Chemosphere. 2023;337:139305.
- [49]Yu G, Chen Q, et al. Glutathione promotes degradation and metabolism of residual fungicides by inducing udp-glycosyltransferase genes in tomato. Frontiers in plant science. 2022;13:893508.
- [50]Gasic K, Korban SS. Transgenic indian mustard (brassica juncea) plants expressing an arabidopsis phytochelatin synthase (atpcs1) exhibit enhanced as and cd tolerance. Plant Mol Biol. 2007;64:361-9.
- [51]Guo J, Dai X, et al. Overexpressing gsh1 and aspcs1 simultaneously increases the tolerance and accumulation of cadmium and arsenic in arabidopsis thaliana. Chemosphere. 2008;72:1020-6.
- [52]Yamazaki S, Ueda Y, et al. Rice phytochelatin synthases ospcs1 and ospcs2 make different contributions to cadmium and arsenic tolerance. Plant direct. 2018;2:e00034.
- [53]Liang Zhu Y, Pilon-Smits EAH, et al. Overexpression of glutathione synthetase in indian mustard enhances cadmium accumulation and tolerance. Plant Physiol. 1999;119:73-80.
- [54]Zhang X, Rui H, et al. Overexpression of a functional vicia sativa pcs1 homolog increases cadmium tolerance and phytochelatins synthesis in arabidopsis. Frontiers in plant science. 2018;9:107.