Pathological investigation and molecular detection of bacterial zoonotic diseases of slaughtered cattle in Bangladesh
Abstract
The research was undertaken to investigate the important bacterial zoonotic diseases in slaughtered cattle in Mymensingh district of Bangladesh. The targeted diseases were tuberculosis (TB), leptospirosis, listeriosis, and brucellosis. Samples (mesenteric lymph nodes, lungs, and liver) were collected from 50 slaughtered cattle from different slaughterhouses in Mymensingh district during the periods from October 2019 to November 2021. The diagnosis was made based on gross pathological findings and histopathology by hematoxylin and eosin staining and acid-fast staining. The confirmatory diagnosis was done by polymerase chain reaction using disease-specific primers. Grossly, calcifications and caseation of mesenteric lymph nodes, caseous nodule formation in the liver and lungs, and enlarged mesenteric lymph nodes were the predominant lesions seen. Histopathologically, caseous necrosis and calcification surrounded by fibrous connective tissues in the mesenteric lymph nodes, and granuloma mixed with acid-fast bacteria in the liver were seen as suggestive of infectivity due to TB. Marked lymphoid depletion was seen in listeriosis suspected cases. The PCR amplified Mycobacterium tuberculosis complex (372 bp/16S rRNA), M. bovis (MPB83/600 bp), Leptospira interrogans serovar Hardjoprajitno (HP/323 bp) and Listeria monocytogenes (InlC/517 bp) species-specific amplicons in 09, 09, 05, and 05 cattle, respectively. Brucellosis was not identified in any cases in this study. In conclusion, deadly zoonotic diseases (TB, leptospirosis, and listeriosis) are present in slaughtered cattle having public health importance. Therefore, more extensive monitoring and epidemiological surveys are necessary for the effective prevention and control of zoonotic diseases of cattle.
INTRODUCTION
In Bangladesh, the meat market is a neglected area. Slaughter animals receive minimal attention from a sanitary standpoint, and there is no ante-mortem examination of animals prior to slaughtering, as well as the facilities provided in the slaughterhouse are very poor. As a result, humans are frequently exposed to zoonotic infections from slaughterhouses [1]. Farmers, livestock producers, abattoir employees, and the general public are all at risk of infection due to close relationships with animals and to a lack of knowledge about meat-borne zoonoses [2]. Zoonoses are those infectious diseases that are spread from animals to humans (or, in certain cases, vertebrates). The majority of newly emerging diseases in humans are zoonotic and can originate in animals [3]. They can be transmitted to people by the handling of diseased meat during preparation and consumption, or through intimate contact with animals, such as when hunting, slaughtering, or herding animals [4]. Zoonoses are widespread all over the world, and the public health threat of evolving, reemerging, and neglected zoonoses has been documented in the developed world [5], but they pose a major threat to human health in developing countries like Bangladesh. The important bacterial diseases such as tuberculosis (TB), brucellosis, leptospirosis, and listeriosis are the major burdens on the development of the livestock sector as well as public health importance [6]. Prevention and control of these zoonotic pathogens originated from livestock is very important component to ensure safe food production. In Bangladesh, cattle, goat, and sheep are important livestock animals that contribute to human health by supplying nutrition, employment generation as well as poverty alleviation. Humans can be easily affected by zoonotic diseases from these animals due to our close relationship with animals in agriculture. Still there is no correct data about the transmission of livestock zoonotic pathogens, particularly meat producing animals. In this circumstances, proper diagnosis of zoonotic diseases is very essential in the developing country like Bangladesh. Therefore, this research work was designed to apply technologies to bring about a proper diagnosis of bacterial zoonotic diseases in slaughtered cattle from the Mymensingh district of Bangladesh, which will provide valuable insight regarding preventive and control strategies for zoonotic diseases in cattle.
MATERIALS AND METHODS
Ethical approval
The ethical standards committee of the Bangladesh Agricultural University Research System (BAURES) approved the research work with the reference number BAURES//ESRC/VET/05 dated 11.05.2019.
Collection of samples and gross pathological study
A schematic diagram of the study was represented in Figure 1. A total (n = 50) slaughtered cattle were investigated from different slaughterhouses in Mymensingh district during the periods from October 2019 to November 2021. The detailed history including age and sex of slaughtered cattle were not able to collect due to lack of proper ante-mortem examination in slaughterhouses in Bangladesh. However, a detailed post-mortem examination was carried out at slaughter, and the gross changes were observed and recorded carefully. Samples were collected from the affected organs, mainly lungs, liver, and mesenteric lymph nodes. Portion of tissue samples were immediately fixed in 10% neutral buffered formalin (NBF) and transported to the Department of Pathology, Bangladesh Agricultural University, Mymensingh for histopathological examination. Small pieces of tissues from the lungs, liver, and mesenteric lymph nodes were also collected in sterile falcon tubes and stored at -20°C for molecular detection of specific diseases by Polymerase Chain Reaction (PCR).
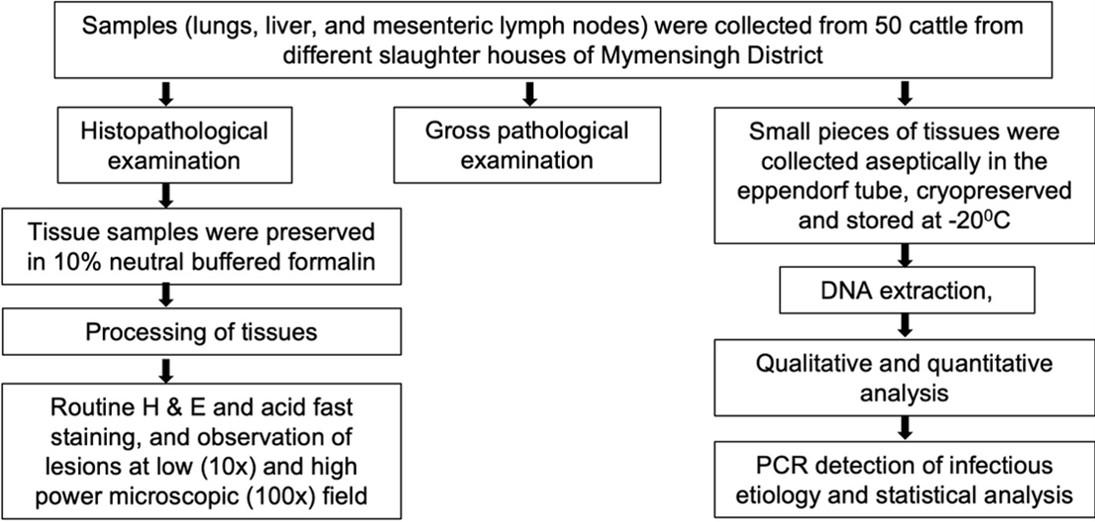
Hematoxylin and eosin (H & E) and acid-fast staining of tissue sections
Representative NBF-fixed tissue samples from affected organs and lymph nodes were processed routinely. Briefly, the tissues were dehydrated with ascending graded alcohols and embedded in paraffin. Then they were sectioned at a thickness of 6 µm. The sectioned tissues were stained with hematoxylin and eosin (H & E) staining [7]. Acid-fast staining [7] was also used to identify acid-fast bacteria in suspected TB-infected organs. The stained slides were mounted using DPX, air dried and examined under a microscope at low (10x) and high (40x and 100x) power objectives, and the images were taken in a microphotographic system (Cell Bioscience, Alphaimager HP, California, USA).
DNA extraction from tissue samples
Commercially available DNA extraction kit (Wizard Genomic DNA purification kit, Promega, USA) was used to extract the microbial DNA from the mesenteric lymph nodes, liver, and lungs for the PCR detection of Mycobacterium tuberculosis complex (MTBC), Mycobacterium bovis, Leptospira interrogans serovar Hardjoprajitno, Listeria monocytogenes, and Brucella abortus as per manufacturer’s instruction. The concentration and quality of the extracted DNA were further measured at 260 nm/ 280 nm using the Spectrophotometer NanodropTM spectrophotometer (IAEA, Scibersdoff, Vienna). The concentration of all the DNA samples obtains ranged between 250-300 ng/ μl and the quality was about 1.8. The DNA was then stored at -200C for molecular detection of diseases by PCR.
Molecular detection of diseases by PCR
PCR was used to detect MTBC, M. bovis, L. interrogans serovar Hardjoprajino, L. monocytogenes, and B. abortus by amplifying 372 bp, 600 bp, 323 bp, 517 bp, and 621 bp fragments of the 16S rRNA gene, MPB83 gene, HP gene, InlC gene, and alkB gene respectively by using specific primers. Primers were obtained from published sequences (Table 1) [8-12] and the PCR primers were synthesized from a commercial source (AIT Biotech, Singapore). The PCR reaction was run in an oil-free thermal cycler (Proplex gradient PCR, USA) in a 25 μl reaction volume using One Taq R Quick-Load R 2X Master Mix kit (New England Biolabs, USA). The reaction mixture consists of 2x PCR master mix, 20 pmol primer in each, 150-200 ng of DNA template, and nuclease free water to make a 25 µl reaction volume. Nuclease free H2O was used instead of template DNA in the reaction mixture.
The thermal profile of 35 cycles of PCR amplification was carried out with an initial denaturation at 94⁰C for 3 mins followed by denaturation at 94⁰C for 30 secs, annealing at 62⁰C for 2 mins (MTBC), 56⁰C for 1 min (M. bovis), 57⁰C for 1.5 mins (M. tuberculosis), 59.3⁰C for 1 min (B. abortus), 57.5⁰C for 1 min (L. interrogans serovar Hardjoprajitno), 55⁰C for 1 min (L. monocytogenes), extension at 68⁰C for 5 mins and final elongation was carried out at 68⁰C for 7 mins.
Table 1. Oligonucleotide primers used in PCR detection of zoonotic pathogens in slaughtered cattle.
Agarose gel electrophoresis
Electrophoresis (WSE-1710Submerge-Mini2322100, China) was performed in 1.5% agarose gel containing ethidium bromide (0.5 μg/ml), and images were captured in a transilluminator (Alpha Imager, USA). To evaluate the size of the amplicons, 100 bp DNA ladder (TackIT, Invitrogen, USA) was used in the agarose gels.
Statistical analysis
The Pearson chi-square test for independence relatedness of the variants and descriptive analysis of the data was carried out by using SPSS 22 (IBM corporation, United States); these enable to estimate the percentages of infection of different zoonotic diseases. A value of P ≤ 0.05 was considered significant at a 95% confidence interval.
RESULTS
Gross pathological observations
Grossly, the mesenteric lymph nodes were enlarged and swollen in 25 cattle (Figure 2A). After cross section of the lymph nodes, caseous materials and calcification were observed within the lymph nodes of four cattle (Figure 2B). In the liver, the lesions included nodule formation in three cattle, hemorrhages in two cattle and cirrhosis in case of twenty cattle (Figure 2D). In the lung parenchyma, multiple caseous nodules were seen in one cattle. Congestion was also seen in the affected four lungs (Figure 2C).
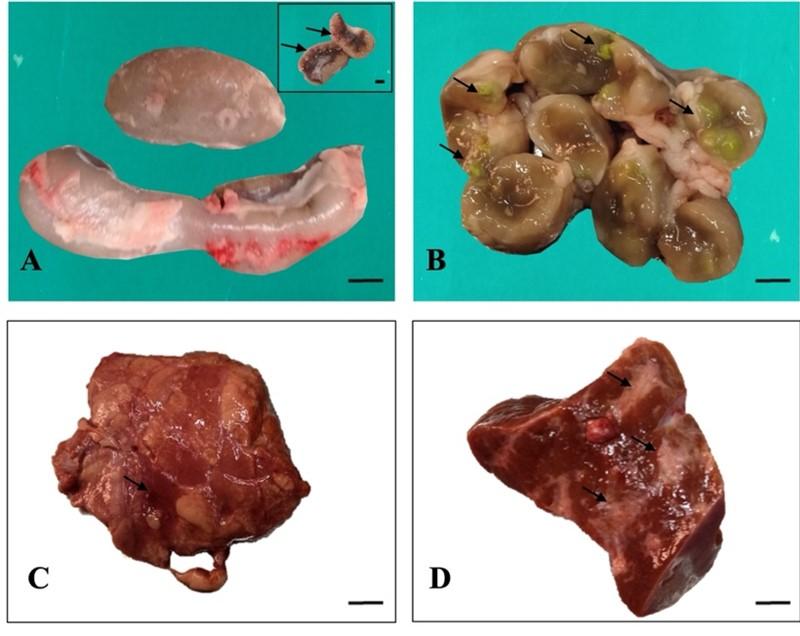
Histopathological observations
Hematoxylin and Eosin staining
Histopathologically, in the mesenteric lymph nodes, the lesions included caseous necrosis with or without calcification in four cattle (Figure 3A-B). In all cases, the caseous necrosis and calcification were surrounded by fibrous connective tissues with huge infiltration of inflammatory cells, predominantly macrophages, lymphocytes, and langhan’s type giant cells (Figure 3A-B), suggestive of infectivity due to TB. In addition, marked lymphoid depletion with enlargement of trabeculae was also seen in the mesenteric lymph nodes in Listeria suspected cases.
In the liver, the lesions included granuloma composed of macrophages, lymphocytes, epithelioid cells, and with or without langhan’s giant cells in four cattle (Figure 3D-E). Additionally, hemorrhages with deposition of hemosiderin pigment were seen in one affected liver (Figure 3D-E) and cirrhosis were also seen in the liver in some cases. In the lungs, multifocal granuloma was seen in one cattle (Figure 3C), hemorrhages, congestion, and pneumonia were observed in two cattle.
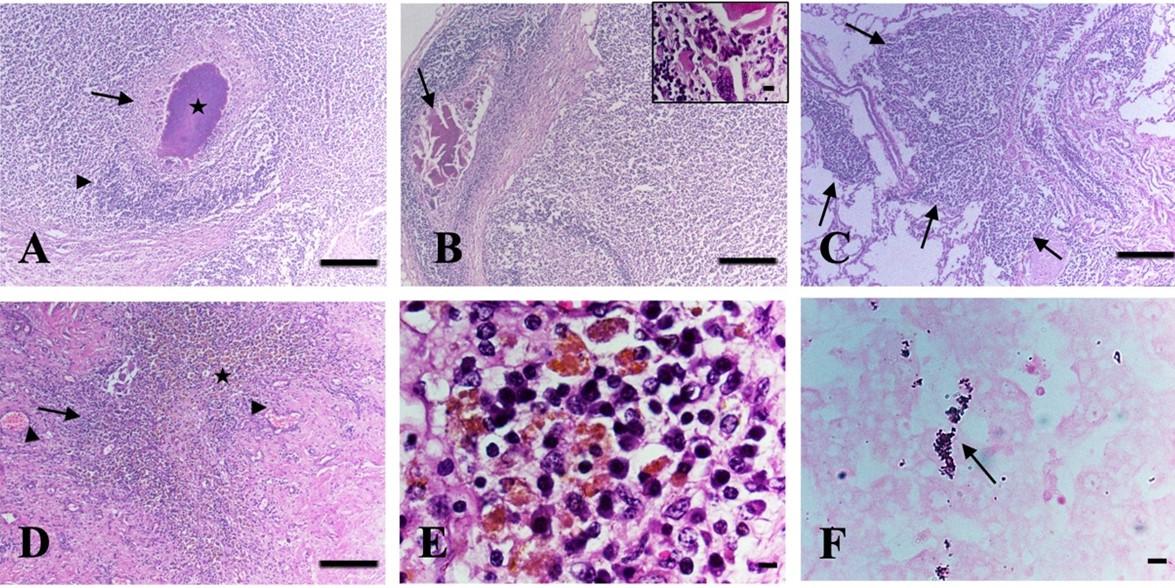
Acid-fast staining
Acid-fast staining of the tissue sections revealed pink-colored rod-shaped acid-fast bacilli in the macrophages or in the epithelioid cells of the granuloma of the liver (Figure 3F) as well as in the caseous center of the mesenteric lymph nodes in four cattle.
Results of PCR
In this study, out of 50 cattle tested, TB, L. interrogans serovar Hardjoprajitno, and L. monocytogenes were confirmed by molecular detection technique (PCR). The extracted DNA (mesenteric lymph nodes, liver, and lungs) was subjected to amplify fragments of the 16S rRNA gene of MTBC (372 bp) (Figure 4A) in nine cattle. To identify further the specific causes of TB, PCR was performed targeting the MPB83 and the H37RvHP genes to detect the infectivity due to M. bovis (600 bp) and M. tuberculosis (667 bp), respectively. MPB83 gene specific amplification of genomic DNA was seen in the mesenteric lymph nodes/liver/lungs of nine cattle (Figure 4B). However, the H37RvHP gene of M. tuberculosis specific amplification was not seen in any cases. PCR was also performed targeting the HP gene of L. interrogans serovar Hardjoprajitno and amplified 323 bp fragments (Figure 5A) in five cattle. The PCR amplified the InlC gene of L. monocytogenes (517 bp) (Figure 5B) in five cattle. PCR targeting to the alkB gene of B. abortus (621 bp) was not generated in this study.
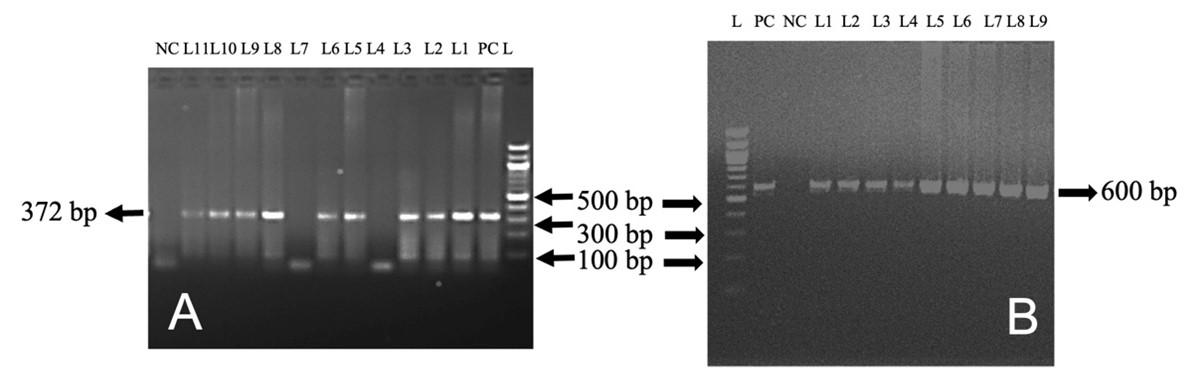
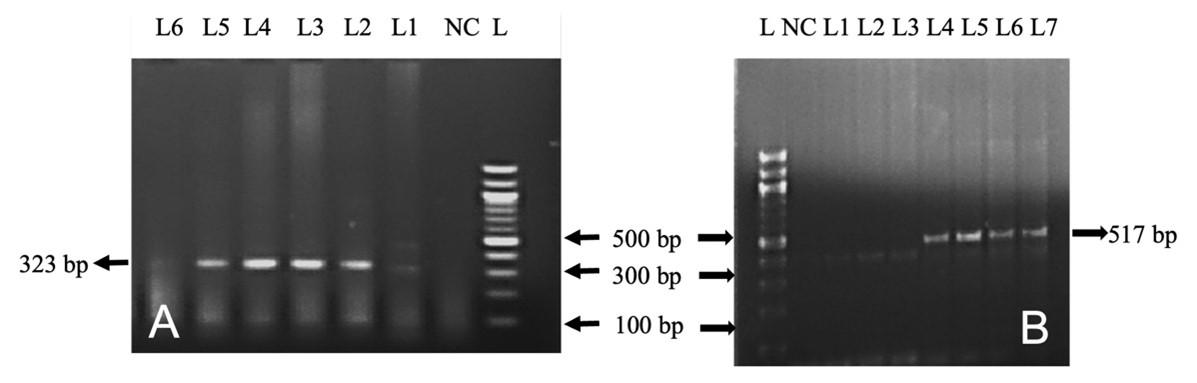
Overall summary statistics
Out of 50 cattle tested, 9 (18%) cattle were infected with TB, 5 (10%) with leptospirosis and 5 (10%) with listeriosis. Among them, percentage of TB was significantly higher (χ2=9.479, df=3, p=0.024) than other organisms in cattle. However, brucellosis was not detected in any cases (Figure 6).
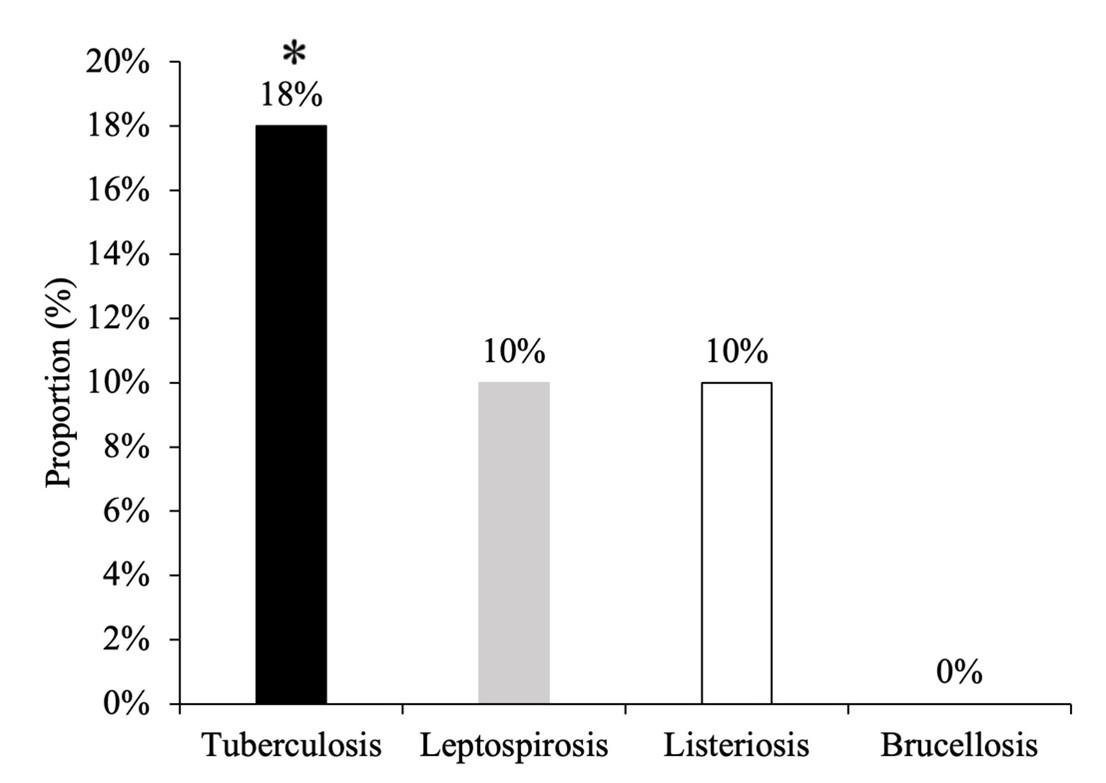
DISCUSSION
Slaughterhouses act as major public health hotspots in terms of spreading diseases in developing countries, including Bangladesh [11, 13]. Thus, this research work was designed to apply few technologies to bring about a proper diagnosis of diseases, with special emphasis on bacterial zoonoses in slaughtered cattle in Mymensingh district of Bangladesh. Out of 50 cattle investigated, TB, leptospirosis, and listeriosis were diagnosed tentatively by gross and histopathological observation. Further, molecular detection technique (PCR) confirmed TB, leptospirosis, and listeriosis in nine, five, and five cattle, respectively.
TB is a chronic granulomatous disease which is characterized by exudative granulomatous caseous inflammatory lesions in the lungs, lymph nodes, also in visceral organs. The disease is occurred in a wide range of animals including domestic and wild animals as well as humans with a high impact on veterinary and public health. TB is caused primarily by acid-fast bacteria of the members of the MTBC, including M. tuberculosis, M. africanum, M. bovis, the Bacillus Calmette– Guérin strain, M. microti, M. canettii, M. caprae, M. pinnipedii, and M. mung. However, infectivity due to M. bovis and M. tuberculosis is common in animals and humans. Bovine TB caused by M. bovis is currently one of the most serious problems in cattle farming in Bangladesh [14]. As primarily a respiratory disease, TB is mainly transmitted by the airborne route although other different routes have been reported. Animals infected through inhalation, organisms enter into the lungs and begin to multiply. Bacilli infect the alveolar macrophages, then picked up by dendritic cells and spread to the local lymph node, followed by the bloodstream reach various organs where they are phagocytosed by macrophages and interact with the cells of the immune system, leading to the development of granuloma. On the other hand, animals infected through ingestion, bacilli enter into the lymph nodes of the mesentery and disseminate to other internal organs [15].
Grossly, in this study, mesenteric lymph nodes were enlarged and swollen. After cross section of the lymph nodes, encapsulated caseous, caseo-calcified, calcified, sticky or gritty materials were seen. Similar lesions were also observed in the liver and lungs. Histopathologically, granuloma composed of foamy macrophages, lymphocytes, with or without langhan’s type giant cells. In mesenteric lymph nodes, a central core of caseous necrosis with or without calcifications encapsulated by fibrous connective tissues was seen. In all cases, the lesions were infiltrated by mononuclear cells with or without langhan’s type giant cells. Pink-colored rod-shaped acid-fast bacilli were observed in the granulomatous lesions, in the cytoplasm of epithelioid cells and in necrosed areas. Such characteristics of TB in various animals were discussed earlier [16, 17]. Additionally, hemorrhages along with hemosiderosis were seen in the liver. This may be due to vasculitis resulting in capillary injury, escape of blood, and destruction of RBC inside the phagocytic system [18] and is considered a cachectic animal.
To confirm TB, PCR was performed to identify the 16S rRNA gene of the MTBC and generated 372 bp amplicons in nine cattle. MTBC is primarily made up of related M. spp., but TB in domestic animals and humans is primarily caused by two MTBC members, M. bovis and M. tuberculosis [14]. Further, PCR was used to identify the MPB83 gene (600 bp) of M. bovis and the H37RvHP gene (667 bp) of M. tuberculosis from cattle. Finally, M. bovis was confirmed in nine cattle, but M. tuberculosis was not confirmed in any cases. Previously, PCR had been successfully applied to detect the 16S rRNA gene of the MTBC, the MPB83 gene of M. bovis and the H37RvHP gene of M. tuberculosis in bovine tissue samples in Bangladesh [9, 11] and nowadays, the incidence of TB due to M. bovis in cattle is increasing in Bangladesh, as described earlier [9].
Leptospirosis is one of the most important zoonotic diseases with a large economic impact on animal production that causes a fall in milk production, abortion, still birth, and low fertility [19]. Humans can be affected from infected animals as a result of occupational or environmental hazards. According to Azevedo et al. (2004) [20], the disease was transmitted to slaughterhouse workers who handled leptospirosis-infected carcasses and organs. The disease is caused by L. interrogans, pathogenic bacteria that infects humans as well as wild and domestic animals [21]. There are around 300 serovars in the Leptospira species, which are divided into 28 serogroups [22]. Among all serovars, the major serovar responsible for leptospirosis in cattle is L. interrogans serovar Hardjo [23]. In many countries of the world, several studies have been conducted to determine the prevalence of L. serovar Hardjo infection in cattle and have reported prevalence rates ranging from 3% to 50% at the animal level [24, 25]. In northern Tanzania, the molecular prevalence of pathogenic Leptospira infection was 7.08% in cattle (n = 452) sampled in local slaughterhouses [26]. In Kelantan, Malaysia, the molecular prevalence of Leptospira sp. in cattle was (0.63%; 4/635) in blood samples and (3.23%; 1/31) in urine samples [27]. Information is lacking about the molecular prevalence of leptospirosis in ruminants in Bangladesh, although the seroprevalence of L. interrogans serovar Hardjo antibody was (47.27%, 52/110) detected by ELISA in commercial dairy cattle [28]. Our study detected L. interrogans serovar Hardjoprajitno in five cattle by PCR. Previously, PCR has been successfully applied to detect L. interrogans serovar Hardjoprajitno from a calf in Chile [29].
Listeriosis is an emerging foodborne bacterial disease caused by L. monocytogenes that affects a wide range of animals, including humans. It is widespread in the environment, but it can cause significant invasive disease in both ruminants and people. Listeriosis is spread by the ingestion of food and water contaminated with saliva, feces, nasal secretions, and aborted material from infected animals. Previously, in Mymensingh municipality of Bangladesh, L. monocytogenes was isolated from 16.66% (2/16) beef and 8.33% (1/12) chevon by bacteriological identification methods [30]. In Dhaka, Bangladesh, 13.2% of Listeria spp. was isolated from various cattle farm environments. In Iraq, the presence of L. monocytogenes DNA was detected by PCR in 2 (1.3%) cattle [31]. In Northeast India, the prevalence of listeriosis in chevon (9.8%) and beef (8.9%) [32]. In addition, human infection with L. monocytogenes has been detected in different countries such as Iran [33], Japan, North America, and Europe [34], as well as in neighboring countries like India [35]. Information is limited about human case studies of listeriosis in Bangladesh. Previous literature suggests that human infections mostly result from ingestion of contaminated and unprocessed animal food materials [36]. Therefore, the public health significance of listeriosis should not be underestimated.
Grossly, in this study, marked lymphadenomegaly and hemorrhages were observed in the mesenteric lymphnodes. Fluen et al. (2019) [37] reported similar findings in case of listeriosis infected animals. Histopathologically, marked lymphoid depletion with widened and inflamed trabeculae was seen in lymphnodes, supported the findings of Fairley et al. (2012) [38]. To confirm L. monocytogenes, PCR was used to amplify fragments of the InlC gene (517 bp) of L. monocytogenes and was found positive in five cattle. Molecular detection is a rapid test to identify L. monocytogenes from all types of food samples, including animal-derived food [39]. Although the first isolations of Listeria spp. were generally performed by the direct culture method, it is difficult to isolate pathogenic Listeria spp. [40]. Molecular tools including PCR, multiplex PCR, and real-time PCR using virulence-associated genes like the mpl gene, prfA gene [41], ssrA gene [42], and InlC gene [43] have been proven to be fast, specific, reproducible, and reliable [44].
Another important bacterial zoonotic disease is brucellosis, caused by the genus Brucella. Brucellosis can result in decreased fertility, miscarriage, poor weight gain, lowered draught power, and a significant decrease in milk production in domestic ruminants. In humans, it is regarded as an occupational disease affecting farmers, slaughterhouse workers, butchers, and veterinarians who work with domestic ruminants. In Bangladesh, brucellosis is endemic in both humans and animals [45]. According to previous studies, the overall seroprevalence of brucellosis in cattle ranged from 2.4%–18.4% and 62.5% at herd-level in Bangladesh [46-48] and in Mymensingh district, the seroprevalence was 2% in cattle [49].
Unfortunately, in this study, brucellosis was not detected in any cases, which may be due to small sample sizes. Therefore, further studies should be conducted with large sample sizes and samples should be collected from different districts of Bangladesh.
CONCLUSIONS
The present study identified the existence of important bacterial zoonotic diseases mainly TB, leptospirosis and listeriosis in the slaughterhouse samples. Among them, percentage of TB was significantly higher in number (9/50) followed by leptospirosis (5/50) and listeriosis (5/50). However, brucellosis was not detected in any cases, which could be due to a smaller number of samples investigated. A high level of TB detected in slaughtered cattle has the potential to enter into the food chain, posing a negative impact on both humans and animals health. Humans and scavenging animals like dogs and cats can be easily affected by these zoonotic diseases from meat-producing animals. The PCR protocols adapted in this study will help detect these diseases within a short period of time. Such sensitive molecular diagnostic tools and epidemiological surveys are necessary for effective detection, prevention, and control of these diseases at the slaughterhouse origin.
ACKNOWLEDGEMENT
The authors thank the Ministry of Science and Technology for National Science and Technology fellowship (NST) (grant no. 95, 2019-20) and the Department of Pathology, Bangladesh Agricultural University for technical and financial support.
AUTHOR CONTRIBUTIONS
MAHNAK and MP were involved in conception and design of the study. NS, SMSHB and MM collected and processed the samples. NS, SS, and MM did DNA extraction. NS did PCR and agarose gel electrophoresis. NS did histopathology. NS, MAHNAK and MP interpreted the results. NS contributed to drafting the article. MAHNAK and MP contributed to revising it critically for important intellectual content. MAHNAK made the final approval of the version to be published. All authors read and agreed on the final version of the manuscript.
CONFLICTS OF INTEREST
There is no conflict of interest among the authors.
References
- [1]Samad MA. Public health threat caused by zoonotic diseases in Bangladesh. Bangladesh J Vet Med. 2011; 9 (2): 95-120.
- [2]Alawa CB, Etukudo-Joseph I, Alawa JN. A 6-year survey of pathological conditions of slaughtered animals at Zango abattoir in Zaria, Kaduna State, Nigeria. Trop Anim Health Prod. 2011; 43(1):127-131. doi: 10.1007/s11250-010-9664-5.
- [3]Quammen D. Spillover: Animal Infections and the Next Human Pandemic. W.W. Norton & Company, New York, USA, 2012.
- [4]Karesh WB, Dobson A, Lloyd-Smith JO, Lubroth J, Dixon MA, Bennett M, et al. Ecology of zoonoses: natural and unnatural histories. Lancet. 2012; 380(9857):1936-1945. doi: 10.1016/S0140-6736(12)61678-X.
- [5]Cutler SJ, Fooks AR, van der Poel WH. Public health threat of new, reemerging, and neglected zoonoses in the industrialized world. Emerg Infect Dis. 2010; 16(1):1-7. doi: 10.3201/eid1601.081467.
- [6]Christou L. The global burden of bacterial and viral zoonotic infections. Clin Microbiol Infect. 2011; 17(3):326-330. doi: 10.1111/j.1469-0691.2010.03441.x.
- [7]Luna L. Manual of Histological Staining Methods of the Armed Forces Institute of Pathology. 3rd edn. McGraw-Hill Book Company, New York, 1968.
- [8]Cousins DV, Wilton SD, Francis BR, Gow BL. Use of polymerase chain reaction for rapid diagnosis of tuberculosis. J Clin Microbiol. 1992; 30(1):255-258. doi: 10.1128/jcm.30.1.255-258.1992.
- [9]Hossain MZ, Rima UK, Islam MS, Habib MA, Chowdhury MGA, Saha PC, et al. Designing polymerase chain reaction (PCR) technique for the detection of specific causes of tuberculosis (TB) in dairy cattle and human. J Vet Sci Med Diagn. 2016; 5(4):4. doi: 10.4172/2325-9590.1000208.
- [10]Jiang XY, Wang CF, Wang CF, Zhang PJ, He ZY. Cloning and expression of Mycobacterium bovis secreted protein MPB83 in Escherichia coli. J Biochem Mol Biol. 2006; 39(1):22-25. doi: 10.5483/bmbrep.2006.39.1.022.
- [11]Jahan AA, Ruba T, Mumu TT, Rana MS, Belal SM, Khan MA, et al. Pathological and molecular detection of diseases of cattle at slaughter. Bangladesh J Vet Med. 2018; 16(2): 213-222. doi: 10.4172/2325-9590.1000208.
- [12]Liu D, Lawrence ML, Austin FW, Ainsworth AJ. A multiplex PCR for species- and virulence-specific determination of Listeria monocytogenes. J Microbiol Methods. 2007; 71(2):133-140. doi: 10.1016/j.mimet.2007.08.007.
- [13]Prabhakar ZN, Lokesh M, Saidaiah M, Sai ES. Awareness regarding zoonotic diseases among the butchers of Proddatur, Kadapa District, AP, India. Iran J Health Saf Environ. 2017; 4: 729-737.
- [14]Ayele WY, Neill SD, Zinsstag J, Weiss MG, Pavlik I. Bovine tuberculosis: an old disease but a new threat to Africa. Int J Tuberc Lung Dis. 2004; 8(8):924-937.
- [15]Ameni G, Aseffa A, Engers H, Young D, Gordon S, Hewinson G, et al. High prevalence and increased severity of pathology of bovine tuberculosis in Holsteins compared to zebu breeds under field cattle husbandry in central Ethiopia. Clin Vaccine Immunol. 2007; 14(10):1356-1361. doi: 10.1128/CVI.00205-07.
- [16]Ambaw M, Gelalcha BD, Bayissa B, Worku A, Yohannis A, Zewude A, et al. Pathology of bovine tuberculosis in three breeds of dairy cattle and spoligotyping of the causative Mycobacteria in Ethiopia. Front Vet Sci. 2021; 8:715598. doi: 10.3389/fvets.2021.715598.
- [17]Domingo M, Vidal E, Marco A. Pathology of bovine tuberculosis. Res Vet Sci. 2014; 97 Suppl: S20-9. doi: 10.1016/j.rvsc.2014.03.017.
- [18]Vihol PD, Patel JM, Patel JH, Raval JK, Varia RD, Makwana PM. Pathomorphological study on leptospirosis in slaughtered goats. J Pharm Innov. 2020; 9(9): 84-88.
- [19]Hernández-Rodríguez P, Díaz CA, Dalmau EA, Quintero GM. A comparison between polymerase chain reaction (PCR) and traditional techniques for the diagnosis of leptospirosis in bovines. J Microbiol Methods. 2011; 84(1):1-7. doi: 10.1016/j.mimet.2010.10.021.
- [20]Azevedo SD, Alves CJ, Andrade JS, Santos FA, Freitas TD, Batista CS. Isolation of Leptospira spp. from kidneys of sheep at slaughter. Arq Inst Biol. 2004; 71(3):383-385.
- [21]Ko AI, Goarant C, Picardeau M. Leptospira: the dawn of the molecular genetics era for an emerging zoonotic pathogen. Nat Rev Microbiol. 2009; 7(10):736-747. doi: 10.1038/nrmicro2208.
- [22]Saito M, Villanueva SYAM, Kawamura Y, Iida KI, Tomida J, Kanemaru T, et al. Int J Syst Evol Microbiol. 2013; 63(Pt 7):2457-2462. doi: 10.1099/ijs.0.047233-0.
- [23]Lilenbaum W, Martins G. Leptospirosis in cattle: a challenging scenario for the understanding of the epidemiology. Transbound Emerg Dis. 2014; 61 Suppl 1:63-68. doi: 10.1111/tbed.12233.
- [24]Savalia CV, Pal M. Studies on the reservoir status of leptospirosis in Gujarat. Indian J Field Vet. 2008; 4(1):7-9.
- [25]Schoonman L, Swai ES. Herd- and animal-level risk factors for bovine leptospirosis in Tanga region of Tanzania. Trop Anim Health Prod. 2010; 42(7):1565-1572. doi: 10.1007/s11250-010-9607-1.
- [26]Allan KJ, Halliday JEB, Moseley M, Carter RW, Ahmed A, Goris MGA, et al. Assessment of animal hosts of pathogenic Leptospira in northern Tanzania. PLoS Negl Trop Dis. 2018; 12(6):e0006444. doi: 10.1371/journal.pntd.0006444.
- [27]Sabri AR, Khairani-Bejo S, Zunita Z, Hassan L. Molecular detection of Leptospira sp. in cattle and goats in Kelantan, Malaysia after a massive flood using multiplex polymerase chain reaction. Trop Biomed. 2019; 36(1):165-171.
- [28]Parvez MA, Prodhan MA, Rahman MA, Faruque MR. Seroprevalence and associated risk factors of Leptospira interrogans serovar Hardjo in dairy cattle of Chittagong, Bangladesh. Pak Vet J. 2015; 35(3): 350-354.
- [29]Salgado M, Otto B, Moroni M, Sandoval E, Reinhardt G, Boqvist S, et al. Isolation of Leptospira interrogans serovar Hardjoprajitno from a calf with clinical leptospirosis in Chile. BMC Vet Res. 2015; 11:66. doi: 10.1186/s12917-015-0369-x.
- [30]Islam MS, Husna AA, Islam MA, Khatun MM. Prevalence of Listeria monocytogenes in beef, chevon and chicken in Bangladesh. Am J Food Sci Health. 2016; 2(4):39-44.
- [31]Al-Ali HJ, Al-Rodhan MA, Al-Hilali SA, Al-Charrakh AH, Al-Mohana AM, Hadi ZJ. Molecular detection of serotype groups of Listeria monocytogenes isolated from gallbladder of cattle and sheep in Iraq. Vet World. 2018; 11(4):431-436. doi: 10.14202/vetworld.2018.431-436.
- [32]Shakuntala I, Das S, Ghatak S, Milton AA, Sanjukta R, Puro KU, et al. Prevalence, characterization, and genetic diversity of Listeria monocytogenes isolated from foods of animal origin in North East India. Food Biotech. 2019; 33: 237-250. doi:10.1080/08905436.2019.1617167.
- [33]Heidarzadeh S, Dallal MMS, Pourmand MR, Pirjani R, Foroushani AR, Noori M, et al. Prevalence, antimicrobial susceptibility, serotyping and virulence genes screening of Listeria monocytogenes strains at a tertiary care hospital in Tehran, Iran. Iran J Microbiol. 2018; 10(5):307-313.
- [34]Swaminathan B, Gerner-Smidt P. The epidemiology of human listeriosis. Microbes Infect. 2007; 9(10):1236-1243. doi: 10.1016/j.micinf.2007.05.011.
- [35]Tirumalai PS. Listeriosis and Listeria monocytogenes in India. Wudpecker J Food Technol. 2013; 1(6):98-103.
- [36]Dhama K, Karthik K, Tiwari R, Shabbir MZ, Barbuddhe S, Malik SV, et al. Listeriosis in animals, its public health significance (food-borne zoonosis) and advances in diagnosis and control: a comprehensive review. Vet Q. 2015; 35(4):211-235. doi: 10.1080/01652176.2015.1063023.
- [37]Fluen TW, Hardcastle M, Kiupel M, Baral RM. Listerial mesenteric lymphadenitis in 3 cats. J Vet Intern Med. 2019; 33(4):1753-1758. doi: 10.1111/jvim.15539.
- [38]Fairley RA, Pesavento PA, Clark RG. Listeria monocytogenes infection of the alimentary tract (enteric listeriosis) of sheep in New Zealand. J Comp Pathol. 2012; 146(4):308-313. doi: 10.1016/j.jcpa.2011.08.004.
- [39]Law JW, Ab Mutalib NS, Chan KG, Lee LH. An insight into the isolation, enumeration, and molecular detection of Listeria monocytogenes in food. Front Microbiol. 2015; 6:1227. doi: 10.3389/fmicb.2015.01227.
- [40]Beumer RR, Hazeleger WC. Listeria monocytogenes: diagnostic problems. FEMS Immunol Med Microbiol. 2003; 35(3):191-197. doi: 10.1016/S0928-8244(02)00444-3.
- [41]Rossmanith P, Krassnig M, Wagner M, Hein I. Detection of Listeria monocytogenes in food using a combined enrichment/real-time PCR method targeting the prfA gene. Res Microbiol. 2006; 157(8):763-771. doi: 10.1016/j.resmic.2006.03.003.
- [42]O’ Grady J, Sedano-Balbás S, Maher M, Smith T, Barry T. Rapid real-time PCR detection of Listeria monocytogenes in enriched food samples based on the ssrA gene, a novel diagnostic target. Food Microbiol. 2008; 25(1):75-84. doi: 10.1016/j.fm.2007.07.007.
- [43]Pournajaf A, Rajabnia R, Sedighi M, Kassani A, Moqarabzadeh V, Lotfollahi L, et al. Prevalence, and virulence determination of Listeria monocytogenes strains isolated from clinical and non-clinical samples by multiplex polymerase chain reaction. Rev Soc Bras Med Trop. 2016; 49(5):624-627. doi: 10.1590/0037-8682-0403-2015.
- [44]Hage E, Mpamugo O, Ohai C, Sapkota S, Swift C, Wooldridge D, et al. Identification of six Listeria species by real-time PCR assay. Lett Appl Microbiol. 2014; 58(6):535-540. doi: 10.1111/lam.12223.
- [45]Rahman AKMA, Saegerman C, Berkvens D, Melzer F, Neubauer H, Fretin D, et al. Brucella abortus is prevalent in both humans and animals in Bangladesh. Zoonoses Public Health. 2017; 64(5):394-399. doi: 10.1111/zph.12344.
- [46]Belal SM, Ansari AR. Seroprevalence of Brucella abortus antibodies in the cattle population in the selected upazilas of Sirajgonj district. Bangladesh J Vet Med. 2013; 11(2):127-130.
- [47]Rahman AKMA, Smit S, Devleesschauwer B, Kostoulas P, Abatih E, Saegerman C, et al. Bayesian evaluation of three serological tests for the diagnosis of bovine brucellosis in Bangladesh. Epidemiol Infect. 2019; 147: e73. doi: 10.1017/S0950268818003503.
- [48]Sikder S, Rahman AKMA, Faruque R, Das S, Gupta AD, Farm CCD, et al. Bovine brucellosis: An epidemiological study at Chittagong, Bangladesh. Pak Vet J. 2012; 32, 499– 502.
- [49]Amin MRK, Rahman MB, Sarkar SK, Kabir SML, Akand MSI. Serological Epidemiology of brucellosis in cattle of Mymensingh district of Bangladesh. J Anim Vet Adv. 2004; 3(12), 898 – 900.