Assessing drug repurposing option for emerging viral diseases: concerns, solutions, and challenges for forthcoming viral battles
Abstract
Since the beginning of time, microorganisms have been in existence. With time, new pathogens have emerged as a result of complex interplay of anthropogenic and natural factors like, human migration, shifts in weather pattern, genetic shuffling of the organisms themselves and more which have been discussed in detail. This review article focuses solely on emerging and re-emerging viruses: Chikungunya, Coronavirus, Dengue, Ebola, Hepatitis C, Herpes Simplex Virus (HSV), Human Immunodeficiency Virus (HIV), Human Papilloma Virus (HPV), Influenza and Zika; and the latest progresses made in finding effective antiviral drugs via drug repurposing as we know this approach outplays de novo production significantly with respect to time and money. In a time where new diseases are being reported once every year, drug repurposing will certainly come in handy in developing antiviral therapeutics promptly. Moreover, the article also discusses major challenges in drug repurposing from finding a patent, dealing with all relevant governing frameworks, to careful and safe handling of viruses, these are some challenges faced by drug repurposing, to name a few. Additionally, the study elaborates on the mechanisms of actions of these drugs as well as the targets whilst including recent and well-known incidences of deadly, viral outbreaks.
INTRODUCTION
Emerging and re-emerging diseases are a major public health challenge. The current rate at which new diseases are being reported is one per year [1]. In the past 3 decades, minimum 33 new pathogens, one of which is HIV, have been identified to be emerging [1]. Research and drug development have brought about effective therapies for a number of infectious diseases, yet there still many exists without a proper drug or vaccine in the 20th century. Over the years, there has been numerous outbreaks worldwide costing lives in thousands and even millions. Table 1 illustrates outbreaks and targeted areas for the year of 2020 (at the time of writing) and 2019. To contain outbreaks and prevent transmissions, the lack of data on novel pathogens has frequently come in the way of action. Most of these viruses had been previously unrecognized. Hence, there is no effective, adequate treatment till date for the majority which calls for an immediate need to produce an antiviral cure. No emergence can be taken lightly; the ongoing pandemic caused by SARS-CoV-2 previously had its relative strains responsible for SARS and MERS epidemics worldwide and both were identified with mild respiratory conditions [2]. With the unpredictable nature of emergence, urgent need for working drugs during a health crisis cannot be met with the pace of traditional drug-making process. Having repurposed drugs ready in an instance of a viral outbreak can further enable widespread control.
Drug repurposing or repositioning (DR) is currently a highly coveted mode to develop antiviral drugs against new or re-emerging diseases. Recycled drugs with antiviral properties have seen a surge in attention due to dearth of suitable treatment for emerging viruses, in the last decade. Also known as drug re-profiling, DR is a promising approach where existing, known drugs/compounds are reworked on to provide new medical indications by seeking different targets for intervention or novel molecular pathways. Potential drug candidates are determined by screening (approved and developmental) libraries of small molecule drugs and other bioactive compounds and undergo a number of computational and experimental techniques [3]. Moreover, there are already thousands of drugs that have successfully cleared clinical trials but nonetheless, failed to efficiently treat; this miscellany of compounds is another source of antiviral [4].
In comparison to the de novo drug development process, repurposed drugs have an undeniable economic upper hand: prompt clinical trials with the likelihood of bypassing phase I trials as the drug has undergone prior testing warranting safety and success [3,4,5]. From the discovery of the compound to the final approval for use on humans, it takes 12 to 16 years on average [5]. The discovery alone stretches for 3-6 years following which preclinical studies are implemented to assess the efficiency and safety of the molecule. This takes about 3 more years. If the results are optimistic, clinical trials (phase I, phase II, phase III) in humans begin and is continued for roughly 5 years. Finally, the molecule is expected to fulfill all required conditions set by an appropriate agency. It is estimated approximately 5% of all the candidates pass the investigation [4]. Adding onto the tedious procedure is the cost in billions of dollars. Besides, drugs that are recycled are undertaken for compassionate use, especially if the viral disease is untreatable or in the case of a pandemic [3]. In a period of 6 years (2012-2017), only twelve new antiviral drugs have been approved for use by the FDA in USA [14]. The de novo process is excruciatingly long and resource-consuming [6].
In this review, the global impact of emerging and re-emerging viral diseases are discussed as well as the progresses made in concocting recycled drugs for these diseases. The article also elaborates on the mechanisms of drug repurposing and the functions and target of drugs.
EMERGING AND RE-EMERGING DISEASES
Emerging diseases are infections that have been recently found in populations. Or infections whose incidence or geographic range is pacing fast and has the potential to increase in near future. Examples of emerging viruses are chikungunya virus (CHIKV), dengue virus (DENV), Ebola (EBOV), hantavirus, HIV, SARS, Zika virus (ZIKV) (Table 1). Such an infection can be caused if the agent has been previously undetected or simply, unknown. It is also possible for known agents to play a role in an emerging disease about which there has been no prior knowledge or conclusive evidence. Diseases caused by these known agents in new populations or geographic locations too are emerging. On the other hand, diseases with recent incident cases after a significantly long period of decline are known as re-emerging diseases. Due to high error rates of replication polymerases, RNA viruses adapt quickly to changing conditions and exploit host organisms. Thus, it is no surprise that most of the emerging or re-emerging viruses are indeed RNA viruses [7].
FACTORS LEADING TO EMERGENCE
Disease emergence is a result of a sophisticated interlinked network of factors and influences. These determinants are detailed below.
Genetic variation
Genetic changes such as mutation, recombination and re-assortment are common adaptive mechanisms viruses use to evolve [7,18]. In fact, viruses have an intrinsic tendency for such genetic changes to generate progenies much phenotypically diverse than the parent [19]. The mutation rate in RNA viruses is between 10-4-10-6 bp per generation [20]. These mutations, in structural or non-structural proteins, can be detrimental or advantageous to the virus, in the event of which it will aid the virus in adapting to a new host or a changing environment. This means that their target host range expands and enables non-human viruses to gain precedence over humans [18].
New strains of influenza virus responsible for the pandemics in 1957, 1968 and 2009 were a consequence of gene shuffling via re-assortment [21]. This is possible when two different strains of a virus invade the same host causing a new progeny to appear. This new strain has mixed genome derived from the two and can efficiently invade hosts that lack immunity to the novel pathogen [18].
Recombination occurs with two similar strains of virus in the same host to form a new strain after a genetic exchange [18]. Instances of infection by novel viruses have great potential to cause havoc among human and non-human populations alike. Novelty can not only be achieved by any of these methods but by a combination of any two or all three. This is simply a strategy for survival when the odds against hosts fare low.
Table 1. Viral pandemics and epidemics in the past centuries.
Environmental changes
Anthropological changes, for example, deforestation and subsequent disruption of habitats have led to migration of animals for food and shelter, thus bringing them closer to human contact. In other words, diseases that were limited to these animals are now being able to be transferred to humans [1].
Climate change
Climate change has long been known to cause indirect and even unprecedented damages to the earth and its living beings, macro- or microscopic. Thus, extremities in weather pattern like, excessive rainfall, heat waves etc. can once again lead to removal of animal species from their niches [1].
Human behavioral pattern
In the past 150 years, human population has dramatically risen coupled with significant shrinkage in time taken to travel around the globe [22].
Humans are widely changing in terms of their demographics. Since the rise in urbanization, human movement has hardly ever been stagnant. The global travel system is ever running, transporting people from one region to another, from one country to another, from one continent to another. Lack of precaution and prior knowledge easily cause transmission from one host to another.
Bioterrorism
An aberration from the natural mode of emergence, bioweapons can alone potentially score a massive death toll. This is because the technology and equipment necessary to build biological weapons, or in other words, grow killer pathogens, is rife and has multidimensional, legitimate uses that do not pose any need of secrecy [23]. Additionally, few other factors like poverty and social disparity, war and consequential famine, shortfall of political will have also been taken into account for the role they play in emergence [24].
Abattoirs
The meat industry has been criticized for its malpractices in the abattoirs or slaughterhouses. These practices include a range of human, animal and environmental issues. Apart from this, workers in these places tend to work in close proximity of one another and are not educated on how various slaughterhouse-related diseases spread.
There have been several studies conducted in abattoirs that have come to similar conclusions. Such as the one in Kumasi, where 75% of all arriving cattle have an unknown health status [25]. The survey explored the practices and facilities at the slaughterhouse as well as the knowledge and attitude of butchers. Common practices included inadequate protection, ingestion activities at lair age and killing sites and unsanitary conditions in general. These butchers also thought they were only at risk during the time of cutting and very reliant on self-medication.
Lack of hygiene and inadequate facilities increase the chances of meat contamination and occupational hazard to butchers. As a consequence of not having sufficient knowledge on disease transmission, diseases can easily be introduced to mass public. Another study found from studying 142 abattoirs in Kenya that only 31% of the workers had knowledge of zoonotic disease [26]. Even though the state of slaughterhouses varies from country to country, it is important that the workers be trained in this aspect and that sanitation be of top priority in these places.
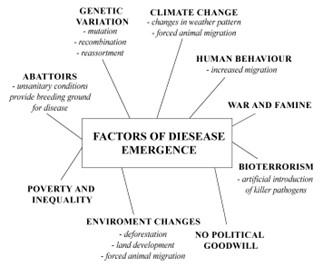
MAJOR DRUG TARGETS AGAINST VIRUSES
There are many drug targets in viruses, both structural and non-structural, which form the principle behind developing antiviral drugs (Table 2). Knowing and understanding the target of drugs is one of the most important steps in developing antiviral drugs. This allows for better understanding of how the drug may act on the target [27].
Many antiviral agents target certain features of viruses to combat diseases caused by them. Not only structural but also the non-structural parts of viruses have been the target of antiviral drugs for decades and continue to be so for modern day therapeutics (Table 1). Some of the best possible drug targets are viral enzymes like DNA and RNA polymerases and helicase, and certain structural proteins like channel proteins and envelop proteins, as these are involved in maintaining important steps of the life cycle of many viruses.
The M2 channel protein of influenza viruses are often a major target as they serve as ion channels, allowing hydrogen ions to enter inside the viral envelop, which is a very crucial step for viral uncoating. After attachment and membrane fusion the virus enters the host cytoplasm while it is inside an endosome formed from the host plasma membrane. The acidic environment of the endosome opens up M2 channel proteins on the viral envelop, allowing hydrogen ions to enter inside. The drugs, Amantadine and Rimantadine, target this M2 protein by binding to one of its pockets which hinders its usual function and inhibits hydrogen ions from entering inside the viral envelop, stopping viral uncoating and releasing its ribonucleoprotein complex into host cell cytoplasm [28,29].
The neuraminidase enzyme of the influenza virus is also a propitious drug target of antiviral drugs (Table 2). This enzyme is required to digest the hemagglutinin receptors on the surface of infected cells that are holding the virus and infected cell together which is needed so that the virus can infect new cells. It also digests neuraminic acid in respiratory mucus, helping in faster spread of virus. The drugs, Zanamivir, Oseltamivir, Peramivir and Laninamivir, bind to the active site on the viral neuraminidase, and inhibits its function. As a result, the virus cannot infect new cells [30,31].
RNA viruses use their RNA polymerase for replication and translation of mRNA transcripts to produce more virus particles, and thus this viral enzyme is seen as a promising target of antiviral drugs. Favipiravir (T-705) is an antiviral drug that targets the viral RNA polymerase and stops amplification of viral genome of influenza virus [32,33]. The envelop protein Hemagglutinin (HA) is yet another target for anti-influenza drugs (Table 2). This protein is complementary to the receptors on host cell membrane and is thus required by virus to attach to host cell. The compounds, MBX2329 and MBX2546, which seem to be very promising antiviral drugs, are complementary to the viral HA and bind to them to prevent viral HA from binding to its complementary receptors on cell surface of the host, thereby inhibiting the virus from attaching and to host cell [34]. Another drug used in China and Russia named Arbidol, intercalates into the membrane lipids of the virus and interacts with HA and stabilizes it, and consequently prevents the virus from fusing with host cell membrane [35].
Some drugs aim at viral nucleoproteins as these binds to viral RNA and prevent it from digestion by cellular RNase. Nucleozin, another drug, causes viral nucleoproteins to aggregate, preventing them from binding with viral RNA, causing viral RNA transcription to stop [36-38].
Viral DNA polymerases are also a common target for many antiviral drugs because these enzymes are responsible for viral genome multiplication. Acyclovir is used as a drug to treat Herpes Simplex Virus (HSV) infection. Acyclovir is converted to its monophosphate derivative via action of viral thymidine kinase. Consecutive diphosphorylation and triphosphorylation converts it into acyclovir triphosphate which is a competitor for the substrate of the viral DNA polymerase, deoxyguanosine triphosphate. The 3′-hydroxyl group is absent in acyclovir triphosphate, which is required to elongate the DNA chain. This terminates the synthesis of viral DNA [39,40].
Other viral enzymes, like helicase and primase, are targeted by drugs because their actions initiate viral DNA replication. Pritelivir is another drug used to combat HSV which targets the helicase-primase complex of the virus and thereby stopping viral genome replication (Table 2) [41,42].
Viral proteases serve as excellent antiviral targets as they have very important roles to play in viral life cycle. They cleave specific peptide bonds in viral polyprotein precursors so that the cleaved proteins can now carry out their functions as they are set free from the polyprotein complex [43]. Viral protease inhibitors work by selectively binding to viral proteases and preventing the proteolytic cleavage of viral protein precursors. Atazanavir is a protease inhibitor that is used to treat HIV patients [44].
These were some of the major targets aimed by antiviral drugs in attempt to alleviate different viral infections. Most of the attempts to develop antiviral drugs are made focusing on the effective blocking of one or more of these targets of a particular virus. Although, effective antiviral drugs might not be available for every virus but, different laboratory and clinical experiments of recent time have shown positive effects of different non-specific drugs and other chemicals on the major targets of a range of lethal viruses. Thus, these antiviral targets provide the blueprints for non-specific drugs to be aimed against with a view to repurposing of existing counter measures.
Table 2. Major drug targets aimed to design antiviral drugs in different viruses.
DRUG REPURPOSING FOR EMERGING VIRAL DISEASES
Drug repurposing, or drug repositioning as many may term it, is the use of an already existing and approved drug to treat some other disease which is not the drug’s initial target disease. It differs from de novo drug design and synthesis because unlike de novo drug design, drug repurposing utilizes already-existing drugs instead of having to design a completely new one from scratch. This method has many advantages over conventional de novo drug synthesis. First of all, the chances of disapproving a drug for safety reasons are very low as it has already gone through early-stage trials and have been deemed safe for use in human body. Secondly, the time requirement for overall drug development is less as a lot of important steps like preclinical testing, evaluation of safety and, in some cases, developing the formulation, already have been completed. Thirdly, any type of research with the drug is easier as information related to it is already available [45,46].
It has been estimated that it costs approximately US$1 billion and takes roughly about 10 years to bring a drug from the laboratory to market. However, when the costs of failed projects are taken into account, the real cost rises to about US$2.6 billion [47]. Repurposing drugs save a lot of money and time and thus this field of research is getting more and more attention. The FDA’s 505(b)(2) new drug application pathway allows the approval of a new drug when a product contains similar active ingredients to a previously approved drug. In 2016 the number of 505(b)(2) drug approvals reached a 13-year high of 48 [48].
In the past, there have been examples of drug repurposing to treat many diseases. Different drugs originally used to treat kidney diseases, glaucoma, epilepsy, urinary-tract infections, etc. have been repurposed to treat malaria [49]. Drugs used to locally treat cutaneous metastases of breast cancer were repositioned for oral treatment for leishmaniasis [50]. Repurposing drugs for viral diseases have become something of utmost importance in recent times as such diseases continue to be some of the deadliest in the world. Past pandemics and endemics caused by viruses have claimed thousands of lives and there are always chances of more such breakouts in the near future.
Creating more options to fight the deadliest viruses is of top priority and thus repurposing drugs to use them as antiviral agents is gaining more popularity and importance. Different experimental and clinical tests have shown that drugs that were used as antibacterial agents could be used for combatting Zika virus [51] and those used for influenza viruses and coronaviruses had been proven to successfully work against Ebola virus [52,53]. Moreover, anti-parasitic drugs are being repurposed to treat influenza viruses [54].
Taking a look at the recent pandemic caused by SARS-CoV-2, many drugs have shown positive results when repurposed to treat COVID-19. Remdesivir, a drug previously used to treat other viral infections like Ebola and MERS-CoV, has been found to produce some desirable effects against SARS-CoV-2 in clinical tests. In other clinical tests, drugs like Hydroxychloroquine and Chloroquine, which were used as remedy for malaria for decades, have also been found to be effective against COVID-19 by reducing the viral load. Among the ten major virus outbreaks in the last 50 years, some of the viruses had very high fatality rates, e.g., Marburg virus (1967) had a fatality rate of 80%, Nipah virus (1998) had 77.6 % and Hendra virus (1994) had a fatality rate of 57% [55].
With their super-fast pace of mutation, viruses may lead to worldwide pandemics and as viral diseases are mostly communicable, designing antiviral drugs is something of the highest concern. Thus, antiviral drug repositioning has become an important weapon in combating some of the most dangerous and deadliest viruses.
REDISCOVERING THERAPEUTIC FUNCTIONS OF EXISTING DRUGS AGAINST EMERGING VIRAL DISEASES
Chikungunya virus
Transferred by mosquitoes Aedes Aegypti and Aedes Albopictus, Chikungunya Virus (CHIKV) is an Alphavirus that belongs to the Togaviridae spectrum [56]. Originating from African countries and slowly marking its territory in India and Southeast Asia, CHIKV was seen to have spread to U.S. territories by the means of tourists and travelers. Reported cases of chikungunya virus infection had spiked up to over 2 million within the past few years. The maximum number of Chikungunya cases was reported in 2006 as well as approximately 2944 deaths reported during August-November 2006 epidemic in Ahmedabad, India [57]. During the most recent outbreak of this virus in 2016, the mortality rate was 47.9 deaths / 100,000 populations in Pernambuco, Brazil. Many other deaths from various countries including Puerto Rico, have been reported over years, during the same months: August- November 2014 and 2016, this was further validated through research that Chikungunya was in fact the disease responsible for these deaths [58].
CHIKV infection is explicitly observed as high fever and sharp joint pains in humans and it can be severe involving encephalitis in a few cases, especially in neonates. The virus can cause arthritis. It infects connective tissues between the femur and tibia as well. However, symptoms are seen to resolve within 10 days, but in some cases, recurrent joint pain is seen to persist, causing permanent damage and hardship for the rest of the patients’ lives [59]. For reasons stated above and more, Chikungunya posed a grave threat to humanity over the years.
Chikungunya virus was found to possess a spherical envelope with an icosahedral capsule which has a width of 65nm. The positive stranded RNA is assembled of approximately 12000 base pairs. There are two open reading frames (ORFs). The 5’ ORF codes the 5 nonstructural proteins, NSP1-4. The 3’ ORF is translated from the 26 sRNA which synthesizes the proteins: C, E1, E2, E3, 6K [60].
Up until now, no licensed vaccine is commercially available against CHIKV and instead therapeutic agents that have shown antiviral activity towards CHIKV are being tested and further examined. Many specific and non-specific drugs have been said to possess anti-virulence against this virus which are expected to be used to treat the CHIKV infection.
Adopting use of Sofosbuvir to restrict viral replication
Sofosbuvir is an antiviral drug which was initially allowed in treating HCV and seen to have antiviral activity against flaviviruses [61]. Further studies showed that Sofosbuvir was successful in inhibiting CHIKV growth in different cell lines including Human Hepatoma cells (Table 3) [62]. Under experimental conditions, infected neonatal mice were subjected to a reference dose of 20mg/kg/day of Sofosbuvir [63] for preclinical trial. Results showed it was effective (EC50 = 17 ± 5 μM) and that viral replication had reduced as well as inflammation and tissue damage. However, phase II of clinical trials was not yet done, so effectiveness of Sofosbuvir in humans could not be guaranteed [64] and given these findings, further research and other interventions might be required here to determine whether it has the potential to act as an antiviral against CHIKV in humans.
Use of Mycophenolic Acids against CHIKV
Mycophenolic acids may have been previously used as anti-proliferative, but studies show that at a concentration of 10μM, it is able to obstruct 99.9% replication of viruses that were tested [65]. Hence MA can be counted as a prospective remedy against CHIKV, however, until it’s activity against CHIKV is explored, effectiveness of this drug is difficult to determine.
Miltefosine for treating CHIKV infection
Miltefosine is an FDA approved, antineoplastic compound, used against visceral leishmaniasis, was seen to have antiviral activity against CHIKV. When cells in a culture medium were experimentally infected with CHIKV, miltefosine was then administered at a dosage of 8.1–16.3 μg/mL, which helped the cells to survive the infection (Table 3) [66]. Similar to Sofosbuvir, further clinical trials are required to validate its effectiveness in humans.
Curcumin forbids surface protein binding
Curcumin is a naturally occurring component of Turmeric, which possesses antiviral activity against viral infections, cancers and has been administered to fight against Dengue Virus (DENV) [67], Herpes Simplex [68] and Human Immunodeficiency Virus (HIV) [69] in various laboratory experiments. Curcumin aids in reducing viral replication by disabling attachment of virus at the cell surface, it does not directly degrade viral RNA. This ability of Curcumin suggests the potential use of its derivatives for antiviral development. Other studies have shown Curcumin to have little to zero side effects when administered at a regular dosage, thus making it less harmful to be considered as a potential antiviral agent [70].
Commonly Used DENV drug, Ribavirin for CHIKV treatment
Ribavirin is a medication adopted for inhibiting DENV [71] which also displays anti CHIKV activity with a 1:1 ratio in combination with Doxycycline. An EC50 value of 4.52 ± 1.42 μM was recorded when this combination was tested on mice for its potential anti-virulence against CHIKV [72]. Other drugs such as Chloroquine had an EC50= 17.2 in an in vitro cell culture [73] but was seen ineffective during human trials. Arbidol was also seen to inhibit CHIV but EC50 value was less than 10 μg/mL in Vero cells [74] and so was considered a weak agent. Although these drugs portray antiviral activity against CHIKV, further research proved Sofosbuvir to be more effective than drugs such as Arbidol, Chloroquine etc.
Influenza virus
Influenza Virus (IFV) is a virus surrounded by an envelope, with a negative sense RNA and is a member of the Orthomyxoviridae family. Influenza virus consists of two types of surface proteins, Hemagglutinin (H) and Neuraminidase (N). There are four categories of Influenza virus (IFV A-D) where Influenza A and B are responsible for the seasonal flu and epidemic, worldwide [75]. Influenza A can be further categorized according to their protein subtypes i.e., H1N1, H3N2 etc. Antigenic shift in surface proteins cause their genome to change and this change in protein subtypes are denoted by the numbers i.e., H3\H1. The mutation renders the immune response of the body to be useless as the new viral progeny is not recognized upon re-infection [76]. According reports made by the World Health Organization (WHO), Influenza is the cause of over 400,000 casualties around the globe.
Influenza virus infection results in respiratory illness that has a series of clinical symptoms such as acute fever, shivering, congested nose, muscle pain as well as diarrhea. A weakened immune system may lead to increase in severity of these symptoms, observed especially in infants and elderly people following infection [77].
There are two licensed Neuraminidase Inhibitors (NAIs) that are adopted for treating IFV infections in many countries, called Zanamivir and Oseltamivir, out of which Oseltamivir has been triumphant in reducing hospital immortality rates and so is the most recognized NAI [78]. However, Oseltamivir was reduced to redundancy due to the rapid, widespread of mutated Oseltamivir- resistant IFV H1N1 during 2008 and by hesitant immunocompromised patients, who did not prefer antiviral therapy due to prolonged viral shedding as it would eventually lead to drug resistance [79]. Thus, researchers set out to discover drugs that would possess anti-virulence as well as be undisrupted when treating resistant viruses.
Nitazoxanide as an antiviral
Nitazoxanide (NTZ) happens to be a lucrative example of IFV. It was primarily introduced for treating intestinal infections caused by helminthic and protozoan parasites. Ever since the rediscovery of its broad-spectrum antiviral properties, it is also recommended for curing IFV infections.
Patients volunteering in a Phase 2b/3 trial, with an age range from 12 to 65, were subjected to double 600mg dosages of Nitazoxanide for 5 consecutive days. Manifested clinical symptoms of Influenza were seen to have alleviated significantly and a better response to 600mg dose rather than a 300mg was observed (Table 3) [80]. Furthermore, combinations of Nitazoxanide with targeted drugs such as Oseltamivir and/or Zanamivir, yielded synergistic results with combination index values ranging from 0.39 to 0.63 [81]. By these values it can be concluded that Nitazoxanide hinders the virulence of IFV more effectively and can be opted for Influenza treatment.
Favipiravir detects viral RNA synthesis
Favipiravir (T-705) gained recognition when it served as a cure against the deadly Ebola Virus (EBOV) back in 2014 [82] and by recent analysis, it has been discovered that T-705 effectively inhibits viral RNA synthesis, demonstrating potential as an anti-influenza drug [83].
An experiment on mice cells were carried out where the mice were infected with intranasal influenza and treated with concentrations 0.3-100 µg/ml of Favipiravir, these cells were seen to sustain from influenza by suppressing synthesis of Tumor Necrosis Factor α (TNF-α) [84]. This was a crucial breakthrough as; TNF-α is a cytokine, responsible for pathogenesis in lungs of humans and mice [85] and had a strong positive correlation to severe lung lesions and pneumonia [86]. A significant reduction in the mean pulmonary virus yield and the rate of mortality in mice infected with influenza virus A/PR/8/34 (3 × 102 PFU) was observed during oral administration of T-705 at 100 mg/kg of body weight/day (four times a day) for 5 days [87].
Additionally, Favipiravir portrayed superior antiviral activity by preventing death of the mice (especially in high viral load) whereas Oseltamivir only delayed their inevitable demise [88] thus proving the hierarchy where T-705 remains most effective against Influenza A, B and C.
A trio drug combination was adopted in a further phase 2b/3 trial where Clarithomycin, Naproxen and Oseltamivir portrayed efficacy in treating severe Influenza [89].
Even though vaccines are considered the most effective measure of prevention, sporadic antigenic shift/mutation amongst influenza viral strains cause produced vaccines to be useless as vaccine production may take up to 6 months and more to fully replenish the population. Hence, a change in its genetic makeup during its production renders the vaccine ineffective. Considering that drug repurposing uses compounds such as enzymes which aid in stopping viral replication instead of protein binding like vaccines, these are comparatively less prone to resistance occurring due to mutation and hence would not require neither as much funds nor time as a simple vaccine would take to reach the population of a country.
Ebola virus
Ebola virus (EBOV) is a group of five genetically distinct members of genus Ebolavirus and Filoviridae family, these are enveloped, non-segmented, negatively stranded RNA virus [90]. The first EBOV breakout took place in Central Africa in a span of 2 years from 2014 – 2016 and was considered to be one of the deadliest epidemics in history, claiming over 11000 lives, receiving a biosafety level 4. Original causes of Ebola virus Disease (EVD) were unknown; however, the medium of spreading was through blood or exchange of bodily fluids with a carrier. Later on, it was discovered that Pteropodidae bats were natural hosts of EBOV. It was difficult to determine whether a person was infected since the symptoms were nonspecific and started out with fever, muscle pain, fatigue etc. but severe cases lead to vomiting, diarrhea, and even brain hemorrhage [91].
Even though it has been more than 40 years since the virus has been discovered, out of the 12 proposed vaccines, only one so far has accomplished phase III trials [92] and thus researchers, in the meantime have tested and accumulated data regarding pre-existing drugs that could be proven to possess anti-virulence against EBOV.
Lysosomatropic Chloroquine against the deadliest Ebola Disease
One such flourishing compound frequently used for its antiviral activity is Chloroquine (CQ), initially introduced as an antimalarial drug. CQ specializes in obstructing viral fusion [93] and has shown its strength against viral diseases conducted in in vitro studies such as HIV, CHIKV and SARS-CoV (Table 3). This FDA approved drug not only reduces the mortality rate but also yielded an EC50 value of 12.5 against the virus when 90mg/kg of CQ was enforced upon the mice, 2 times a day [94]. Although these results increase the probability of CQ being used as an anti-ebola drug, a pseudovirus was used in this experiment to recognize the mechanism of CQ, these questions the efficacy of this drug against EBOV.
Amiodarone, reprocessed for EBOV diseases
Designed as a means to battle arrhythmia, Amiodarone qualified as one of the most meritorious cationic amphiphiles (CAD) for its inhibitory effects on Ebola’s viral mechanism. Amiodarone reportedly halts the viral life cycle during its initial stages adopting the use of multi-ion channel stoppers [95]. Maximum hindrance on viral replication was observed when Amiodarone induced an NPC- like phenotype which yielded an IC50 value of 5.6 in Vero cells infected with recombinant GP-pseudotyped VSV [96]. However, one of the previously mentioned studies claimed that an IC50 value of 0.4 μM was attained when Amiodarone was used against Zaire EBOV (ZEBOV) [97]. Amiodarone and its derivatives make for a suitable inhibitory drug against EBOV, if it is administered before the infection takes place. This is because accumulation of Amiodarone proved better results [98], in contrast to prescribing it after EBOV infection.
Few other notable drugs were seen to have compromised the viral activity of EBOV via early phases in clinical studies, examples include Favipiravir (T-705) [99], GS-5734 (a broad-spectrum antiviral). Most of these FDA approved drugs could be utilized against EBOV infection, but these could only be adapted commercially once it passes the clinical trials proving its inhibiting abilities against EBOV.
Coronavirus
Notoriously known as the culprit behind the 2020 pandemic, coronavirus, better acquainted as SARS-CoV-2, surfaced from Hubei of Mainland China and has successfully carved its name amongst one of the most fatal viruses with the highest number of casualties in history.
The novel Coronavirus (nCoV) is linked with severe acute respiratory syndrome made of positive sense RNA and a genome size of 32 kbp long, belonging to the coronaviridae family [100]. Coronavirus is a zoonotic disease that has triumphantly broken inter-species barriers by transmitting from avian hosts to mammals such as Humans. The coronavirus harboring in humans is characterized by dry cough, sore throats, fever until it infiltrates the lungs leading to acute cases of pneumonia, diarrhea, organ failure, respiratory arrest even cardiovascular diseases and brain damage as seen in the elderly people.
However, pathogenic they may be, most coronaviruses are considered to be mild except for the beta corona virus severe acute respiratory syndrome (SARS) coronavirus (SARS-CoV), first emergence reported in China, 2002 [101]. Middle East respiratory syndrome (MERS) corona virus (MERS-CoV) remains the other notable exception amongst coronavirus associates that were seen to have caused 800+ fatalities originating from Saudi Arabia in 2012 [102]. Genomic analysis revealed SARS-CoV-2 -was seen to belong to a distinct clade from the aforementioned corona viruses.
With passing days, the aggravating global pandemic has pushed the public to their wits ends as number of deceased has crossed over 1.33 million worldwide, causing researchers to wrack their brains and work overtime to ameliorate the dire conditions. Even though a vaccine is the most preferred, most proposed projects are yet to undergo toxicology tests and clinical trials, thus scientists favor the option to reconstruct drugs already existing in the market to fight against this deadly SARS-CoV-2.
Previously denounced Remdesivir to the rescue
Gaining spotlight for being inferior in comparison to other antivirals against EBOV, Remdesivir (GS-5734) was dropped from clinical phases soon when it failed to inhibit EBOV infection as effectively as drugs such as CQ (Table 3). However, that led to it being adopted for further investigation of its efficacy against the novel coronavirus. An EC90 value of 1.76uM was yielded when put to test against in Vero E6 cells. It also successfully blocked infection in Human Liver Cancer Huh7 cells [103]. Remdesivir has become a worldwide sensation, so much so Beximco Pharmaceuticals, Bangladesh has already started commercially manufacturing Bemsivir to be administered against COVID-19 [104]. Due to lack of antivirals against SARS-CoV-2, FDA issued an emergency approval upon evaluating the effectiveness of the adenosine analogue Remdesivir [105]. Renal damage as well as liver malfunction symptoms were encountered such as hypotension and increased creatinine levels during the use of Remdesivir during clinical trials, however, due to lack of placebo and randomized trials, the causation could not be determined [106]. Thus, due to lack of FDA approved drugs against SARS-CoV-2, Remdesivir is serving as a savior against this dreadful disease.
Use of Chloroquine over hydroxychloroquine against SARS-CoV-2
Chloroquine’s effectiveness has been mentioned before for other viral diseases and similar to those, its efficacy against SARS-CoV-2 was seen in a clinical trial in China with over 100 COVID-19 positive patients. 300mg of CQ helped reduce pneumonia in these patients and an EC50 value of 5.74% μM in vitro with SARS-CoV-2 virus. In the same test in vitro with SARS-CoV-2 virus, Hydroxychloroquine (HCQ) had an EC50 value of 0.72% μM [107]. Further testing with synergistic combinations HCQ and Azithromycin was discontinued in patients as there was little to no decrease in rate of virological clearance, as well as the death of a patient while under these doses [108].
Several other inhibitors await further clinical trials to confirm their efficacy against SARS-CoV-2 in humans. Examples include JAK inhibitors, these were seen to reach inhibition concentration in plasma [109], Glucocorticoids were seen to alleviate heart diseases, renal problems occurring due to COVID-19 [110] etc.
However, a few studies have reported no therapeutic effects of Remdesivir, Hydroxychloroquine and Chloroquine against COVID-19. This is most likely due to factors such as genetic diversity which influence drug action across different regions of the globe [111,112].
Herpes Simplex virus
Herpes Simplex Virus (HSV) is a large DNA molecule with a genome length of 152 kbp minimum, encased in an icosahedral capsid and is also enveloped. From the Herpesviridae family, HSV is a pathogen causing lesions in mucosal cavities, both oral cavity and genital infections. There are 8 known types of HSV which can be further divided into three subtypes: alpha, beta, and gamma. Human A-herpes cause HSV-1 and HSV-2, the two common HSV infections worldwide. HSV-1 is responsible for oral and perioral infections while HSV-2 is the agent of genital infections [113], although HSV-1,2, are more commonly known for these two conditions, they also cause several other eye infections, skin diseases and infections that adversely affect other organs [114].
Since the breakout of HSV in 1980, a nucleoside analogue called acyclovir (ACV) had been clinically adopted to battle this disease. However, due to its long-term use, HSV has grown to become resistant towards ACV, rendering it useless [115,116]. Thus, other drugs are being tested for their efficacy against HSV.
Ciclopirox for Simplex Virus
The FDA approved anti-mycotic drug, Ciprolax Olamine (CPO), is mainly used against fungi, dermatophytes, yeast etc. infection but it has also recently shown antiviral activity against HSV infections occurring in the eye. CPO reduced HSV replication in mice and in Vero cells an EC50 value of 0.27 μM (Table 3) [117].
Dengue virus
The flavivirus, Dengue virus (DENV) is a positive sense RNA virus wrapped inside an envelope that mainly spreads via mosquitoes and ticks. This spherical virion is of 40-50 nm in diameter with its RNA of around 11kbp. There are 4 serotypes from DENV1-4 and each of these possess an icosahedral capsid with a lipid bilayer on the nucleocapsid [118].
DENV carrier mosquitoes were considered to be endemic in tropical regions and across South Asia but are seen to have spread to American countries as well. Annually an estimate of 10-50 million people is infected by DENV worldwide and as vaccines are not yet available, drugs and repurposed drugs are used for treatment. Some major symptoms remain fever, nausea, vomiting and weakness but dengue infections can cause hemorrhaging fever denoted by vascular permeability, plasma leakage, hypovolemia, shock, and so on.
Table 3. List of drugs that could be repurposed for a range of different emerging viral diseases.
PCZ protects against lethality of DENV
Prochlorperazine (PCZ) is an FDA approved drug to treat headache, nausea which happens to be DENV symptoms, it was further tested out on mice in vivo infected with DENV-2 as it showed positive results against other flaviviridae viruses such as Hepatitis C virus (HCV) in lab tested experiments (Table 3) [119].
Mice were treated with PCZ in both oral and injection routes where an LD90 value of 400 and 191 was achieved respectively. Since these values are well below hazardous, higher dosages as well as low ones were adopted where both showed successful survival rates. High dose of 5 mg PCZ/kg/day was seen to have prevented fatalities. Furthermore, an EC50 value of 137uM was seen when DENV-2 infected mice were treated with PCZ-dimaleate [120]. With further testing on specific DENV protein complexes, the mode of action of newly discovered antiviral activity of PCZ could be explored and used as a remedy against PCZ.
Ivermectin to treat DENV
Ivermectin (IVC) is an anti-parasitic drug introduced in 1981 to be used against nematodes and arthropods, against which it is extremely potent [121]. It has shown effectiveness against DENV Helicase in a FRET based helicase assay where an IC50 value of 500 nm was achieved [122] and this initiates the possibility of adapting Ivermectin as an anti-dengue drug after further research.
Anticholestrderemic drug for DENV treatment
Lovastatin, as the name suggests, is a statin used in treating cholesterol. This fungal metabolite reduces lipid level and decreases risks of developing cardiovascular diseases. It has also shown antiviral properties against DENV replication in Vero cells, with an IC50 of 38.2 μM and an IC50 value of 11.9 μM when tested on HEMC-1 cells (Table 2) [123].
There is one commercially available vaccine used against DENV but due to its verifying efficacy in various patients, repurposed drugs are a better option for treatment.
Zika virus
Zika Virus (ZIKV) is an arbovirus of the same family as DENV with RNA as its genetic material. It was isolated in 1947 from mosquito, Aedes Africanus, however the first time reported of human ZIKV infection was 1953 [124]. Although it is mainly known to be transmitted via mosquitos especially during daytime, other forms of spreading were also reported which include transfer from mother to infant during pregnancy where, the infant either dies of abnormalities soon after birth or the mother faces a miscarriage [125-127]. Cases of ZIKV transmitted via sexual intercourse were also seen where fluid exchange between infected and healthy caused the infection to occur. Some clinical symptoms manifested are conjunctivitis, rash (mostly, papular), headache, edema, nausea, arthralgia etc. [128].
Azithromycin for ZIKV
Azithromycin (AZ) is an antibacterial drug used to treat different types of bacteria such as pneumonia, diarrhea, ear infection etc. but it has been seen to inhibit ZIKV infected hPSC cells. An EC 50 value of 15 μM at 75% baseline infection was seen which is evidence of its efficacy against ZIKV infections. AZ was further chosen as it is an FDA approved drug that can be used during pregnancy without adverse effects [51].
Some previously mentioned drugs that were seen to have antiviral activities against flaviviruses, Sofosbuvir, Ivermectin, Mycophenolic Acid etc. were also tested against ZIKV and gave promising results. Ivermectin had successfully inhibited ZIKV replication in hNSC cells with a Cmax value of 280 ng/mL [129]. Mycophenolic acids have shown efficacy against ZIKV as well when tested on HeLa cells, an EC50 value of 1uM was found [130]. Thus, with further research efficacy against ZIKV can be evaluated.
Human Papilloma virus
Another notorious, asymptomatic sexually transmitted infection is the Human Papillomavirus (HPV) with around 79 million affected only in the US. It usually does not cause any adverse effects and goes away with time, but some extreme cases were seen to have led to cancer. Currently HPV vaccine shields the population from this infection but out of 100 types, it only protects from 4 HPV types and thus drugs are opted for inhibiting viral replication and curing this infection.
Oral medicines such as acyclovir have been used for curing HPV. In a pilot test, ACV showed remarkable results by alleviating HPV in 4 out of 6 patients and has been administered as a cure in many countries, however further testing is lacking [131].
Cidofovir gel creams
Cidofovir is originally an AIDS anti-viral drug that happens to be a nucleotide analogue but has shown efficacy against HPV by inhibiting 50% cell inhibition and proliferation at 15 μm dosage, with a HeLa cell survival rate of 75.35% ± 14% (Table 2) [132].
Hepatitis C virus
Hepatitis C virus (HCV) is made of a single strand RNA that is of positive sense and is approximately 9.6kb in length that affected over 170 million people worldwide [133]. Western countries are most commonly affected by a liver disease called Chronic Hepatitis C which is caused by HCV infection, usually observed amongst the elderly (30+). HCV is a blood-borne disease that is transmitted during blood transfusion, intravenous drug use, high risk sexual activity, childbirth etc. [134].
An effective vaccine is not yet present as existing drugs are adopted as remedies for HCV. Although there are target drugs present to treat HCV, a lot of other drugs, not specific to HCV can be repositioned to inhibit HCV.
Chlorcyclizine
Originally over the counter, allergy medicine, Chlorcyclizine successfully inhibits early-stage replication of the Hepatitis C virus and has an EC50 value of 50nm in vitro using HuH 7.5.1 cells [135].
Synergistic use of Sofosbuvir and Ribavirin
A successful trial with 321 patients showed the use of Sofosbuvir and Ribavirin over a span of 12-16 weeks showed how well these drugs work against HCV. This was calculated using sustained virological response after 12 weeks of therapy which was 88.2% (Table 3) [136].
Human immunodeficiency virus
Human Immunodeficiency Virus (HIV) is a retrovirus belonging to the Retroviridae family and genus Lentivirus genus. It is made of 2 identical copies of single stranded RNA. This branches in to two categories of Human Immunodeficiency Viruses, HIV-1 and HIV-2 which differ according to their certain genomic characteristics but can both lead to Acquired Immunodeficiency Syndrome (AIDS). AIDS is a chronic, fatal condition caused by HIV which leads to both physical as well as societal hazards [137]. Since its emergence in 1981, USA, HIV has taken over 20 million lives during the Sub-Saharan Epidemic and has over 65 million affected worldwide. These life-threatening situations have forced the hands of scientists to wield existing pharmacopoeia to battle this virus before it takes control over the world.
Auranofin aided reduction of Viral RNA
The anti-rheumatic drug used against arthritis was adopted in clinical trials against HIV where each group of five people were administered Auranofin along with antiretroviral drugs (ARV). There was a fall in Viral RNA load in the blood of the patients without having adverse effects [138].
Combination of Auranofin and Buthionine Sulfomin had beneficial long-term effects in the patients’ immunity against HIV as viral titer had been relatively low for longer periods of time [139]. Thus, Auranofin as anti-HIV medicine can be used alongside other ARVs to aid viral inhibition (Table 3).
Combination of Lopinavir-Ritonavir yielded satisfactory results against HIV
400mg of Lopinavir and 100mg of Ritonavir decreased viral load of wild type HIV by 50% of EC50 where another second phase clinical trial showed that this combination could inhibit 50 copies/mm of HIV RNA effectively in 78% of the patients [140]. Thus, showing how both these drugs are capable of suppressing retroviral RNA and is useful against HIV in the long run.
Hence, due to lack of vaccines and ineffective immunization methods against HIV, refurbished drugs would have to be considered to decrease mortality rate due to AIDS.
SCOPES AND CHALLENGES OF DRUG REPURPOSING AGAINST VIRAL DISEASES
Scopes for drug repurposing lie in various fields, like research institutions, repurposing technology companies and the pharmaceutical industry, all with the same goal but with different skills, abilities and business ideas. The research institutions are involved with all the scientific work of identifying new indications for existing drugs. The repurposing technology companies are more concerned with services like providing databases, creating drug libraries and assessing different compounds. The pharmaceutical companies deal with the last stages of development of a particular drug and also take care of post-marketing activities [141]. Many renowned companies and organizations are collaborating with others for drug discovery. GSK (GlaxoSmithKline) had announced on collaborating with The University of California to set up The Laboratory for Genomic Research, a project worth $67 million, to help with drug discovery in the oncology, immunology and neuroscience areas. Atomwise Inc. declared to use its AI technology to assist Eli Lily and with their drug discovery endeavors. Perlara, a company dealing with the treatment of rare diseases, collaborated with Cure Sanfilippo Foundation and Mission: Cure to target chronic pancreatitis, to try to repurpose existing drugs to treat Sanfilippo Syndrome and chronic pancreatitis, respectively. Benevolent AI, Europe’s largest AI company, decided to join forces with Parkinson’s UK and The Cure Parkinson’s Trust, to repurpose three existing drugs to treat Parkinson’s disease [142]. There are many biomedical research organizations who are ready to support any type of biological research related to human diseases, like Sanford-Burnham [143].
The Global Virus Network (GVN) is a platform which is a coalition consisting of the top virologists from across the world, whose objective is to work on the knowledge of the mode of action of different viruses and developing drugs and vaccines for viral diseases. It is operating in more than 30 countries and has 53 centers and 9 affiliates. GVN is such an entity where top scientists from different countries can come together under one roof and combine their expertise to find better and more efficient solutions for viral diseases [144]. There are also many individual research institutions in many countries whose focus is on viral research, e.g., The Institute of Human Virology, Institute of Molecular Virology (USA), The Macfarlane Burnet Centre (Australia), The John P. Robarts Research Institute (Canada), Institute of Virology (Germany), Institute for Virus Research at the University of Kyoto (Japan), Centre for Virology at the University of Bergen (Norway), D.I. Ivanovskiy Institute of Virology, JSC NARVAC (Russia), to name a few [145]. The presence of such labs, academia and organizations, operating in different countries and the collaboration among them, greatly enhance the scopes of repurposing drugs for viral diseases.
Certain measures can be acquired to increase scope of drug repositioning. A common platform like Experimental Drug Network can be established which will consist of experts to provide economic, regulatory and legal knowledge concerning the repurposing of drugs. Moreover, if there is one single database that will contain relevant information concerning all available marketed drugs and drugs sent for approval, which will be accessible by the WHO and other regulatory agencies of the world, then work will become easier. Role of FDA, EMA and other agencies can be extended, and they can be made to do research on drug safety and toxicology.
As much as promising and useful it may be, drug repurposing faces quite some challenges including regulatory and legal framework issues, intellectual property rights, market exclusivity, maintenance of product lifecycle, etc. [146]. Finding a patent and ensuring patent rights for a new repurposed drug are some major obstacles. If the patent claims cannot be differentiated from already-available information, then the existing knowledge of the new use of the repurposed drug often curbs chance of obtaining a patent. The patentee must also be able to present accurate, conclusive and persuasive data that shows the use of the repurposed drug to treat its new indication. The Off-patent Drug Bill 2015-16 was introduced in June 2015 to address instances in which a drug’s patent has become invalid, but a new indication of the drug has been found. Although it is supported by a number of medical affiliations, it still has not passed into legislation. There may be problems from pharmaceutical industry perspective, especially if the new target disease is outside of an organization’s usual target diseases.
In 2015, when Turing Pharmaceuticals acquired marketing authorization for the drug, Daraprim (Pyrimethamine), a drug originally used to treat malaria but later found to be useful against toxoplasmosis and pneumocystis pneumonia when used in conjunction with other drugs, increased the price of one tablet from $13.50 to $750 [147].
In case of off-patent drugs, finding intellectual property (IP) protection is more difficult. Another limiting factor in this emerging field is the lack of skilled people who can deal with all the legal issues related to a new repurposed drug. Although many institutions and biotech companies are involved in drug repurposing, they often fail to find the correct resources to commercialize their products and also lack required funds. At times, the communication and partnership between academia and industry is not enough. Another major challenge of drug repurposing is that it is usually considered that the effect of the drug on its new target will be less efficient than its older indication, and thus, the new target validation must be proven strongly [148].
In addition, carrying out a research that deals with viruses are often less favored because there is always a chance of the virus getting outside of the lab even when the highest biosecurity measures are followed. Many countries do not have the sophisticated and state-of-the-art laboratories and equipment needed to carry out such sensitive trials and experiments. The fear of not being able to handle a virus properly often may stop the repurposing of drugs for viral diseases.
CONCLUSIONS
Viral diseases have been and still is a major health concern globally. Diseases caused by viruses spread more easily, are some of the most leading causes of deaths each year all around the world and are the causative agents for endemics and pandemics. The ability of viruses to mutate so easily and quickly makes it more difficult to control them. Thus, developing antiviral drugs remain one of the most important agenda in the science community. Antiviral drugs target both structural (e.g., envelope, channel proteins, etc.) and non-structural (e.g., viral enzymes) parts of viruses to try to stop the viral life cycle and prevent multiplication of new viruses inside the body. More compounds and molecules, both synthetic and natural, are being discovered to be able to combat viruses. In addition to de novo drug synthesis, drug repurposing or drug repositioning, is also being used to treat viral diseases. Drug repurposing is the use of an already approved and marketed drug to treat another disease, which is not the disease it was initially designed for. It has become an emerging field because it has many advantages over conventional de novo drug synthesis- it requires less time and less money, to name a few. Repositioning of drugs to treat some of the leading viral diseases has become a major point of interest, and many drugs have already been repurposed, or are on the way.
Many antibacterial drugs, anticancer drugs or even drugs used to treat malfunctioning body organs have been found to be useful against viruses. Not only that, but drugs also previously used for one virus have been found to be effective on a completely different virus. Drugs have been repurposed to work against some of the deadliest viruses like coronaviruses, HSV, Ebola and Zika, thus paving ways to tackle such dangerous pathogens in better ways. Many institutions and laboratories around the world specialize in research on disease-causing viruses, and the collaboration of these academia with other companies, organizations and health agencies greatly increase scope for drug repurposing for emerging viral diseases. However, it does face some difficulties, especially related to patent, legal framework, regulatory and price issues. But with more involvement of the concerned authorities and regulating bodies, these challenges can be overcome.
Viral diseases have been and continue to be one of the major death-causing factors/entities annually all over the world. Moreover, viruses have been the causative agent for a lot of the world’s pandemics and endemics, which have not only affected and killed humans, but led to substantial and often irrecoverable economic and infrastructural losses. Thus, discovering drugs and designing vaccines for the world’s deadliest viruses always remain of topmost priority for the science community. In this fight against viruses, coming up with more antiviral drugs is perhaps the best course of action and strategy to combat them, and keeping this in mind, drug repurposing is undoubtedly a promising field in this regard. Drug repurposing has several advantages over conventional de novo drug synthesis, and as a result it is getting more attention, interest and popularity among researchers. The repurposing of drugs allows multiple known substances and compounds to be used against one virus, instead of just relying on one. It can be said that drug repositioning is a strong ray of hope to combat viral diseases because of all the benefits it has over traditional drug synthesis. And although it does face certain challenges, with proper involvement and efforts of the relevant government bodies and institutions, drug repurposing can be used as a very efficient tool to fight some of the most dangerous viruses of the world, which had previously claimed lives and affected countless more and pose the same threat for the future.
ACKNOWLEDGEMENTS
Authors are thankful to the members of Swift Integrity Computational Lab, Dhaka, Bangladesh, a virtual platform of young researchers, for their supports during the preparation of the manuscript. Authors received no funding from external sources.
AUTHOR CONTRIBUTIONS
SBS, FK, and HI conducted the literature searches and reviews. They have also written the draft manuscript along with MAU. YA and MAU conceived and designed the study. MHR assisted in the formatting. BS, MAU, and YA edited and revised the original draft. All authors approved the final version for publication.
CONFLICTS OF INTEREST
The authors declare that there is no conflict of interest regarding the publication of this manuscript.
References
- [1]Heymann DL, Rodier GR. Hot spots in a wired world: WHO surveillance of emerging and re-emerging infectious diseases. The Lancet infectious diseases. 2001 Dec 1;1(5):345-353.
- [2]Islam H, Rahman A, Masud J, Shweta DS, Araf Y, Ullah MA, Sium SM, Sarkar B. A Generalized Overview of SARS-CoV-2: Where Does the Current Knowledge Stand?. Electron J Gen Med. 2020; 17 (6): em251.
- [3]Mercorelli B, Palù G, Loregian A. Drug repurposing for viral infectious diseases: how far are we?. Trends in microbiology. 2018 Oct 1;26(10):865-876.
- [4]García-Serradilla M, Risco C, Pacheco B. Drug repurposing for new, efficient, broad spectrum antivirals. Virus research. 2019 Apr 15;264: 22-31.
- [5]Nosengo N. New tricks for old drugs. Nature. 2016 Jun 16;534(7607):314-316.
- [6]U.S. Food and Drug Administration [Internet]. U.S. Food and Drug Administration. 2020. Available from: https://www.fda.gov/
- [7]Nichol ST, Arikawa J, Kawaoka Y. Emerging viral diseases. Proceedings of the National Academy of Sciences. 2000 Nov 7;97(23):12411-2.
- [8][Internet]. 2020 [cited 13 August 2020]. Available from: https://www.washingtonpost.com/graphics/2020/local/retropolis/coronavirus-deadliest-pandemics/
- [9]LePan N. Visualizing the History of Pandemics [Internet]. Visual Capitalist. 2020 [cited 13 August 2020]. Available from: https://www.visualcapitalist.com/history-of-pandemics-deadliest/
- [10]1918 Pandemic (H1N1 virus) | Pandemic Influenza (Flu) | CDC [Internet]. Cdc.gov. 2020 [cited 13 August 2020]. Available from: https://www.cdc.gov/flu/pandemic-resources/1918-pandemic-h1n1.html
- [11]Number of deaths due to HIV/AIDS [Internet]. World Health Organization. 2020 [cited 13 August 2020]. Available from: https://www.who.int/gho/hiv/epidemic_status/deaths_text/en/
- [12]WHO | SARS (Severe Acute Respiratory Syndrome) [Internet]. Who.int. 2020 [cited 13 August 2020]. Available from: https://www.who.int/ith/diseases/sars/en/
- [13]Gholipour B. 2009 Swine-Flu Death Toll 10 Times Higher Than Thought [Internet]. livescience.com. 2020 [cited 13 August 2020]. Available from: https://www.livescience.com/41539-2009-swine-flu-death-toll-higher.html
- [14]CDC Novel H1N1 Flu | The 2009 H1N1 Pandemic: Summary Highlights, April 2009-April 2010 [Internet]. Cdc.gov. 2020 [cited 13 August 2020]. Available from: https://www.cdc.gov/h1n1flu/cdcresponse.htm
- [15]Ebola virus disease [Internet]. Who.int. 2020 [cited 13 August 2020]. Available from: https://www.who.int/health-topics/ebola/#tab=tab_1
- [16]MERS-CoV worldwide overview [Internet]. European Centre for Disease Prevention and Control. 2020 [cited 13 August 2020]. Available from: https://www.ecdc.europa.eu/en/middle-east-respiratory-syndrome-coronavirus-mers-cov-situation-update
- [17]Coronavirus Update (Live): 20,960,953 Cases and 750,188 Deaths from COVID-19 Virus Pandemic – Worldometer [Internet]. Worldometers.info. 2020 [cited 13 August 2020]. Available from: https://www.worldometers.info/coronavirus/
- [18]Jaijyan DK, Liu J, Hai R, Zhu H. Emerging and reemerging human viral diseases. Annals of Microbiology and Research. 2018;2(1):31-44.
- [19]Menéndez-Arias L. Molecular basis of fidelity of DNA synthesis and nucleotide specificity of retroviral reverse transcriptases. Progress in nucleic acid research and molecular biology. 2002 Jan 1;71: 91-148.
- [20]Lauring AS, Frydman J, Andino R. The role of mutational robustness in RNA virus evolution. Nature Reviews Microbiology. 2013 May;11(5):327-336.
- [21]Holmes EC, Ghedin E, Miller N, Taylor J, Bao Y, St George K, Grenfell BT, Salzberg SL, Fraser CM, Lipman DJ, Taubenberger JK. Whole-genome analysis of human influenza A virus reveals multiple persistent lineages and reassortment among recent H3N2 viruses. PLoS Biol. 2005 Jul 26;3(9): e300.
- [22]Murphy FA, Nathanson N. The emergence of new virus diseases: an overview. InSeminars in Virology 1994 Apr 1 (Vol. 5, No. 2, pp. 87-102). Academic Press.
- [23]O’toole T. Emerging illness and bioterrorism: implications for public health. Journal of urban health. 2001 Jun;78(2):396-402.
- [24]Lederberg J, Hamburg MA, Smolinski MS, editors. Microbial threats to health: emergence, detection, and response. National Academies Press; 2003 Sep 25.
- [25]Otupiri E, Adam M, Laing E, Akanmori BD. Detection and management of zoonotic diseases at the Kumasi slaughterhouse in Ghana. Acta tropica. 2000 Jul 21;76(1):15-19.
- [26]Cook EA, de Glanville WA, Thomas LF, Kariuki S, de Clare Bronsvoort BM, Fèvre EM. Working conditions and public health risks in slaughterhouses in western Kenya. BMC Public Health. 2017 Dec 1;17(1):14.
- [27]Croston GE. The utility of target-based discovery. Expert Opinion on Drug Discovery. 2017;12(5):427–429.
- [28]Betakova T, Nermut MV, Hay AJ. The NB protein is an integral component of the membrane of influenza B virus. Journal of General Virology. 1996Jan;77(11):2689–2694
- [29]Schnell JR, Chou JJ. Structure and mechanism of the M2 proton channel of influenza A virus. Nature. 2008;451(7178):591–595.
- [30]Calfee DP, Hayden FG. New Approaches to Influenza Chemotherapy. Drugs. 1998;56(4):537–553.
- [31]Sidwell RW, Huffman JH, Barnard DL, Bailey KW, Wong M-H, Morrison A, et al. Inhibition of influenza virus infections in mice by GS4104, an orally effective influenza virus neuraminidase inhibitor. Antiviral Research. 1998;37(2):107–120.
- [32]Furuta Y, Takahashi K, Kuno-Maekawa M, Sangawa H, Uehara S, Kozaki K, et al. Mechanism of Action of T-705 against Influenza Virus. Antimicrobial Agents and Chemotherapy. 2005;49(3):981–986.
- [33]Kiso M, Takahashi K, Sakai-Tagawa Y, Shinya K, Sakabe S, Le QM, et al. T-705 (favipiravir) activity against lethal H5N1 influenza A viruses. Proceedings of the National Academy of Sciences. 2009;107(2):882–887.
- [34]Basu A, Antanasijevic A, Wang M, Li B, Mills DM, Ames JA, et al. New Small Molecule Entry Inhibitors Targeting Hemagglutinin-Mediated Influenza A Virus Fusion. Journal of Virology. 2013Jun;88(3):1447–1460.
- [35]Leneva IA, Russell RJ, Boriskin YS, Hay AJ. Characteristics of arbidol-resistant mutants of influenza virus: Implications for the mechanism of anti-influenza action of arbidol. Antiviral Research. 2009;81(2):132–140.
- [36]Kao RY, Yang D, Lau L-S, Tsui WHW, Hu L, Dai J, et al. Identification of influenza A nucleoprotein as an antiviral target. Nature Biotechnology. 2010;28(6):600–605.
- [37]Gerritz SW, Cianci C, Kim S, Pearce BC, Deminie C, Discotto L, et al. Inhibition of influenza virus replication via small molecules that induce the formation of higher-order nucleoprotein oligomers. Proceedings of the National Academy of Sciences. 2011Jun;108(37):15366–15371.
- [38]Pang B, Cheung NN, Zhang W, Dai J, Kao RY, Zhang H, et al. Structural Characterization of H1N1 Nucleoprotein-Nucleozin Binding Sites. Scientific Reports. 2016;6(1).
- [39]St Clair M, Miller W, Miller R, Lambe C, Furman P. Inhibition of cellular alpha DNA polymerase and herpes simplex virus-induced DNA polymerases by the triphosphate of BW759U. Antimicrobial Agents and Chemotherapy. 1984;25(2):191-194.
- [40]Klysik K, Pietraszek A, Karewicz A, Nowakowska M. Acyclovir in the Treatment of Herpes Viruses – a Review. Current Medicinal Chemistry. 2018Sep;25.
- [41]Burrel S, Rouard C, Boutolleau D. Helicase–Primase Inhibitor Pritelivir for HSV-2 Infection. New England Journal of Medicine. 2014;370(17):1663–1664.
- [42]Field HJ, Huang M-L, Lay EM, Mickleburgh I, Zimmermann H, Birkmann A. Baseline sensitivity of HSV-1 and HSV-2 clinical isolates and defined acyclovir-resistant strains to the helicase–primase inhibitor pritelivir. Antiviral Research. 2013;100(2):297–299.
- [43]Debouck C, Metcalf B. Human immunodeficiency virus protease: A target for aids therapy. Drug Development Research. 1990;21(1):1-17.
- [44]Molina J, Andrade-Villanueva J, Echevarria J, Chetchotisakd P, Corral J, David N et al. Once-daily atazanavir/ritonavir versus twice-daily lopinavir/ritonavir, each in combination with tenofovir and emtricitabine, for management of antiretroviral-naive HIV-1-infected patients: 48 weeks efficacy and safety results of the CASTLE study. The Lancet. 2008;372(9639):646-655.
- [45]Corsello S, Bittker J, Liu Z, Gould J, McCarren P, Hirschman J et al. The Drug Repurposing Hub: a next-generation drug library and information resource. Nature Medicine. 2017;23(4):405-408.
- [46]Breckenridge A, Jacob R. Overcoming the legal and regulatory barrier to drug repurposing. Nature Reviews Drug Discovery. 2018;18(1):1-2
- [47]Tufts Center for the Study of Drug Development. Cost to Develop and Win Marketing Approval for a New Drug Is $2.6 Billion.: Tufts Center for the Study of Drug Development; 2014
- [48]Bloom B. Drug Repositioning, Repurposing and Rescue is Growing By Leaps and Bounds!. The 6th Annual Drug Repositioning, Repurposing and Rescue Conference June 27-28; Chicago 2017
- [49]Supuran C. Editorial [ Carbonic Anhydrases as Drug Targets Executive Editor: Claudiu T. Supuran ]. Current Pharmaceutical Design. 2008;14(7):601-602.
- [50]Paladin Labs Inc., 2010. Application for Inclusion of Miltefosine on WHO. Model List of Essential Medicines
- [51]Retallack H, Di Lullo E, Arias C, Knopp K, Laurie M, Sandoval-Espinosa C et al. Zika virus cell tropism in the developing human brain and inhibition by azithromycin. Proceedings of the National Academy of Sciences. 2016;113(50): 14408-14413
- [52]Liu G, Wong G, Su S, Bi Y, Plummer F, Gao G et al. Clinical Evaluation of Ebola Virus Disease Therapeutics. Trends in Molecular Medicine. 2017;23(9):820-830.
- [53]Correction: Experimental Treatment with Favipiravir for Ebola Virus Disease (the JIKI Trial): A Historically Controlled, Single-Arm Proof-of-Concept Trial in Guinea. PLOS Medicine. 2016;13(6): e1002066.
- [54]Rossignol J. Nitazoxanide: A first-in-class broad-spectrum antiviral agent. Antiviral Research. 2014;110: 94-103.
- [55]Mortality rates of viruses worldwide | Statista [Internet]. Statista. 2020 [cited 19 May 2020].
- [56]Schwartz O, Albert M. Biology and pathogenesis of chikungunya virus. Nature Reviews Microbiology. 2010;8(7).
- [57]Mavalankar D, Shastri P, Bandyopadhyay T, Parmar J, Ramani KV. Increased Mortality Rate Associated with Chikungunya Epidemic, Ahmedabad, India. Emerging Infectious Diseases. 2008;14(3)
- [58]Freitas ARR, Donalisio MR, Alarcón-Elbal PM. Excess Mortality and Causes Associated with Chikungunya, Puerto Rico, 2014–2015. Emerging Infectious Diseases. 2018;24(12):2352–5.
- [59]Reddy V, Desai A, Krishna SS, Vasanthapuram R. Molecular Mimicry between Chikungunya Virus and Host Components: A Possible Mechanism for the Arthritic Manifestations. PLOS Neglected Tropical Diseases. 2017;11(1).
- [60]Jose J, Snyder JE, Kuhn RJ. A structural and functional perspective of alphavirus replication and assembly. Future Microbiology. 2009;4(7).
- [61]Bullard-Feibelman KM, Govero J, Zhu Z, Salazar V, Veselinovic M, Diamond MS, et al. The FDA-approved drug Sofosbuvir inhibits Zika virus infection. Antiviral Research. 2017.
- [62]Sofia MJ, Bao D, Chang W, Du J, Nagarathnam D, Rachakonda S, et al. Discovery of a β-d-2′-Deoxy-2′-α-fluoro-2′-β-C-methyluridine Nucleotide Prodrug (PSI-7977) for the Treatment of Hepatitis C Virus. Journal of Medicinal Chemistry. 2010;53(19):7202–7218.
- [63]European Medicines Agency. 2013. Committee for Medicinal Products for Human Use (CHMP) assessment report. European Medicines Agency, London, United Kingdom.
- [64]Ferreira AC, Reis PA, Freitas CSD, Sacramento CQ, Hoelz LVB, Bastos MM, et al. Beyond Members of the Flaviviridae Family, Sofosbuvir Also Inhibits Chikungunya Virus Replication. Antimicrobial Agents and Chemotherapy. 2018;63(2).
- [65]Subudhi BB, Chattopadhyay S, Mishra P. Current strategies for inhibition of chikungunya infection. Viruses. 2018;10(5)
- [66]Vora J, Patel S, Sinha S, Sharma S, Srivastava A, Chhabria M, et al. Structure based virtual screening, 3D-QSAR, molecular dynamics and ADMET studies for selection of natural inhibitors against structural and non-structural targets of Chikungunya. Journal of Biomolecular Structure and Dynamics. 2018;37(12)
- [67]Padilla-S L, Rodríguez A, Gonzales MM, Gallego-G JC, Castaño-O JC. Inhibitory effects of curcumin on dengue virus type 2-infected cells in vitro. Archives of Virology. 2013Jan;(3).
- [68]Kutluay SB, Doroghazi J, Roemer ME, Triezenberg SJ. Curcumin inhibits herpes simplex virus immediate-early gene expression by a mechanism independent of p300/CBP histone acetyltransferase activity. Virology. 2008;(2).
- [69]Mazumder A, Raghavan K, Weinstein J, Kohn KW, Pommier Y. Inhibition of human immunodeficiency virus type-1 integrase by curcumin. Biochemical Pharmacology. 1995;49(8).
- [70]Devassy JG, Nwachukwu ID, Jones PJH. Curcumin and cancer: barriers to obtaining a health claim. Nutrition Reviews. 2015;73(3).
- [71]Subudhi BB, Chattopadhyay S, Mishra P. Current strategies for inhibition of chikungunya infection. Viruses. 2018;10(5)
- [72]Rothan HA, Bahrani H, Mohamed Z, Teoh TC, Shankar EM, Rahman NA, et al. A Combination of Doxycycline and Ribavirin Alleviated Chikungunya Infection. Plos One. 2015;10(5).
- [73]Roques P, Thiberville S-D, Dupuis-Maguiraga L, Lum F-M, Labadie K, Martinon F, et al. Paradoxical Effect of Chloroquine Treatment in Enhancing Chikungunya Virus Infection. Viruses. 2018;10(5):268.
- [74]Ghildiyal R, Gabrani R. Antiviral therapeutics for chikungunya virus. Expert Opinion on Therapeutic Patents. 2020
- [75]Forrest HL, Webster RG. Perspectives on influenza evolution and the role of research. Animal Health Research Reviews. 2010;11(1)
- [76]Types of Influenza Viruses [Internet]. Centers for Disease Control and Prevention. Centers for Disease Control and Prevention; 2019 [cited 2020May16]. Available from: https://www.cdc.gov/flu/about/viruses/types.htm
- [77]Antigenic Characterization [Internet]. Centers for Disease Control and Prevention. Centers for Disease Control and Prevention; 2019 [cited 2020May16]. Available from: https://www.cdc.gov/flu/about/professionals/antigenic.htm
- [78]Guillemard E, Tondu F, Lacoin F, Schrezenmeir J. Consumption of a fermented dairy product containing the probiotic Lactobacillus caseiDN-114 001 reduces the duration of respiratory infections in the elderly in a randomized controlled trial. British Journal of Nutrition. 2009;103(1)
- [79]Fiore AE, Fry A, Shay D, Gubareva L, Bresee JS, Uyeki TM, et al. Antiviral agents for the treatment and chemoprophylaxis of influenza — recommendations of the Advisory Committee on Immunization Practices (ACIP) [Internet]. MMWR. Recommendations and reports: Morbidity and mortality weekly report. Recommendations and reports. U.S. National Library of Medicine; 2011 [cited 2020May18]. Available from: https://www.ncbi.nlm.nih.gov/pubmed/21248682
- [80]Haffizulla J, Hartman A, Hoppers M, Resnick H, Samudrala S, Ginocchio C, et al. Effect of nitazoxanide in adults and adolescents with acute uncomplicated influenza: a double-blind, randomised, placebo-controlled, phase 2b/3 trial. The Lancet Infectious Diseases. 2014;14(7).
- [81]Belardo G, Cenciarelli O, Frazia SL, Rossignol JF, Santoro MG. Synergistic Effect of Nitazoxanide with Neuraminidase Inhibitors against Influenza A VirusesIn Vitro. Antimicrobial Agents and Chemotherapy. 2014Jan;59(2).
- [82]Oestereich L, Lüdtke A, Wurr S, Rieger T, Muñoz-Fontela C, Günther S. Successful treatment of advanced Ebola virus infection with T-705 (favipiravir) in a small animal model. Antiviral Research. 2014;105.
- [83]Furuta Y, Takahashi K, Fukuda Y, Kuno M, Kamiyama T, Kozaki K, et al. In Vitro and In Vivo Activities of Anti-Influenza Virus Compound T-705. Antimicrobial Agents and Chemotherapy. 2002.
- [84]Tanaka T, Kamiyama T, Daikoku T, Takahashi K, Nomura N, Kurokawa M, et al. T-705 (Favipiravir) suppresses tumor necrosis factor α production in response to influenza virus infection: A beneficial feature of T-705 as an anti-influenza drug. Acta virologica. 2017;61(01):48–55.
- [85]Wang Y, Zhang Y, Lu W, Wang L. Serum Tumor Necrosis Factor-α and Interferon-γ Levels in Pediatric Mycoplasma pneumoniae Pneumonia: A Systematic Review and Meta-Analysis [Internet]. Canadian respiratory journal. Hindawi; 2018 [cited 2020Jun7]. Available from: https://www.ncbi.nlm.nih.gov/pmc/articles/PMC6151362/
- [86]Cheung C, Poon L, Lau A, Luk W, Lau Y, Shortridge K, et al. Induction of proinflammatory cytokines in human macrophages by influenza A (H5N1) viruses: a mechanism for the unusual severity of human disease? The Lancet. 2002;360(9348):1831–1837.
- [87]Takahashi K, Furuta Y, Fukuda Y, Kuno M, Kamiyama T, Kozaki K, et al. In Vitroandin Vivo Activities of T-705 and Oseltamivir against Influenza Virus. Antiviral Chemistry and Chemotherapy. 2003;14(5):235–241.
- [88]Hung IF, To KK, Chan JF, Cheng VC, Liu KS, Tam A, et al. Efficacy of Clarithromycin-Naproxen-Oseltamivir Combination in the Treatment of Patients Hospitalized for Influenza A(H3N2) Infection. Chest. 2017;151(5).
- [89]Paules C, Subbarao K. Influenza. The Lancet. 2017;390(10095):697–708.
- [90]Feldmann H, Geisbert TW. Ebola hemorrhagic fever. The Lancet. 2011;377(9768):849–862.
- [91]Meyers L, Frawley T, Goss S, Kang C. Ebola Virus Outbreak 2014: Clinical Review for Emergency Physicians. Annals of Emergency Medicine. 2015;65(1):101–108.
- [92]Marzi A, Mire CE. Current Ebola Virus Vaccine Progress. BioDrugs. 2019Feb;33(1):9–14.
- [93]Savarino A, Boelaert JR, Cassone A, Majori G, Cauda R. Effects of chloroquine on viral infections: an old drug against today’s diseases? [Internet]. The Lancet. Infectious diseases. Elsevier Ltd.; 2003 [cited 2020Jun7]. Available from: https://www.ncbi.nlm.nih.gov/pubmed/14592603/
- [94]Madrid PB, Chopra S, Manger ID, Gilfillan L, Keepers TR, Shurtleff AC, et al. A Systematic Screen of FDA-Approved Drugs for Inhibitors of Biological Threat Agents. PLoS ONE. 2013May;8(4).
- [95]Shoemaker CJ, Schornberg KL, Delos SE, Scully C, Pajouhesh H, Olinger GG, et al. Multiple Cationic Amphiphiles Induce a Niemann-Pick C Phenotype and Inhibit Ebola Virus Entry and Infection. PLoS ONE. 2013;8(2).
- [96]Chatelain P, Laruel R, Gillard M. Effect of amiodarone on membrane fluidity and Na+ K+ ATPase activity in rat-brain synaptic membranes. Biochemical and biophysical research communications. 1985 May 31;129(1):148-154
- [97]Salata C, Baritussio A, Munegato D, Calistri A, Ha HR, Bigler L, Fabris F, Parolin C, Palù G, Mirazimi A. Amiodarone and metabolite MDEA inhibit Ebola virus infection by interfering with the viral entry process. Pathogens and disease. 2015 Jul 1;73(5):ftv032..
- [98]Sissoko D, Laouenan C, Folkesson E, M’lebing AB, Beavogui AH, Baize S, Camara AM, Maes P, Shepherd S, Danel C, Carazo S. Experimental treatment with favipiravir for Ebola virus disease (the JIKI Trial): a historically controlled, single-arm proof-of-concept trial in Guinea. PLoS medicine. 2016 Mar 1;13(3):e1001967.
- [99]Sheahan TP, Sims AC, Graham RL, Menachery VD, Gralinski LE, Case JB, Leist SR, Pyrc K, Feng JY, Trantcheva I, Bannister R. Broad-spectrum antiviral GS-5734 inhibits both epidemic and zoonotic coronaviruses. Science translational medicine. 2017 Jun 28;9(396).
- [100]Peiris JS, Guan Y, Yuen KY. Severe acute respiratory syndrome. Nat Med 2004; 10 (suppl 12): S88–597
- [101]Zhu N, Zhang D, Wang W. China Novel Coronavirus Investigating and Research Team. A novel coronavirus from patients with pneumonia in China, 2019 [published January 24, 2020]. N Engl J Med.
- [102]Zaki AM, van Boheemen S, Bestebroer TM, Osterhaus AD, Fouchier RA. Isolation of a novel coronavirus from a man with pneumonia in Saudi Arabia. N Engl J Med.
- [103]Warren T, Jordan R, Lo M, Soloveva V, Ray A, Bannister R, Mackman R, Perron M, Stray K, Feng J, Xu Y. Nucleotide prodrug GS-5734 is a broad-spectrum filovirus inhibitor that provides complete therapeutic protection against the development of Ebola virus disease (EVD) in infected non-human primates. InOpen Forum Infectious Diseases 2015 (Vol. 2, No. suppl_1, pp. LB-2). Infectious Diseases Society of America.
- [104]Tirumalaraju D. Beximco Pharmaceuticals launches first generic remdesivir for Covid-19 [Internet]. Pharmaceutical Technology. 2020 [cited 2020Jun28]. Available from: https://www.pharmaceutical-technology.com/news/beximco-pharmaceuticals-remdesivir-generic/
- [105]Ashley Rhoades, MBS, RAC Senior Associate, Regulatory … [Internet]. [cited 2020, Jul’4]. Available from: https://www.fda.gov/media/137564/download
- [106]Eastman RT, Roth JS, Brimacombe KR, Simeonov A, Shen M, Patnaik S, Hall MD. Remdesivir: A Review of Its Discovery and Development Leading to Emergency Use Authorization for Treatment of COVID-19. ACS Central Science. 2020 May 27.
- [107]Dong L, Hu S, Gao J. Discovering drugs to treat coronavirus disease 2019 (COVID-19). Drug DiscovTher [Internet]. 2020; 14 (1): 58–60.
- [108]Molina JM, Delaugerre C, Le Goff J, Mela-Lima B, Ponscarme D, Goldwirt L, de Castro N. No evidence of rapid antiviral clearance or clinical benefit with the combination of hydroxychloroquine and azithromycin in patients with severe COVID-19 infection. Med Mal Infect. 2020 Mar 30;50(384):30085-30088.
- [109]Richardson P, Griffin I, Tucker C, Smith D, Oechsle O, Phelan A, Stebbing J. Correspondence Baricitinib as potential. The Lancet. 2020;6736: 2019-2020.
- [110]Talukdar J, Bhadra B, Dasgupta S, Nagle V. Potential of natural astaxanthin in alleviating the risk of cytokine storm and improve health in COVID-19: A scoping review.
- [111]Beigel JH, Tomashek KM, Dodd LE, Mehta AK, Zingman BS, Kalil AC, Hohmann E, Chu HY, Luetkemeyer A, Kline S, de Castilla DL. Remdesivir for the treatment of Covid-19—preliminary report. The New England journal of medicine. 2020 May 22.
- [112]Acharya Y, Sayed A. <? covid19?> Chloroquine and hydroxychloroquine as a repurposed agent against COVID-19: a narrative review. Therapeutic Advances in Infectious Disease. 2020 Aug; 7:2049936120947517.
- [113]Grinde B. Herpesviruses: latency and reactivation—viral strategies and host response. J. Oral Microbiol. 5: 22766.
- [114]Suazo PA, Tognarelli EI, Kalergis AM, González PA. Herpes simplex virus 2 infection: molecular association with HIV and novel microbicides to prevent disease. Medical microbiology and immunology. 2015 Apr 1;204(2):161-76.
- [115]Latief MA, Chikama T, Ko JA, Kiuchi Y, Sakaguchi T, Obana A. Inactivation of acyclovir-sensitive and-resistant strains of herpes simplex virus type 1 in vitro by photodynamic antimicrobial chemotherapy. Molecular Vision. 2015
- [116]Pan D, Kaye SB, Hopkins M, Kirwan R, Hart IJ, Coen DM. Common and new acyclovir resistant herpes simplex virus-1 mutants causing bilateral recurrent herpetic keratitis in an immunocompetent patient. The Journal of infectious diseases. 2014 Feb 1;209(3):345-349.
- [117]Bernier KM, Morrison LA. Antifungal drug ciclopirox olamine reduces HSV-1 replication and disease in mice. Antiviral research. 2018 Aug 1;156: 102-106.
- [118]Clyde K, Kyle JL, Harris E. Recent advances in deciphering viral and host determinants of dengue virus replication and pathogenesis. Journal of virology. 2006 Dec 1;80(23):11418-11431.
- [119]Gastaminza P, Whitten-Bauer C, Chisari FV. Unbiased probing of the entire hepatitis C virus life cycle identifies clinical compounds that target multiple aspects of the infection. Proceedings of the National Academy of Sciences. 2010 Jan 5;107(1):291-6.
- [120]Simanjuntak Y, Liang JJ, Lee YL, Lin YL. Repurposing of prochlorperazine for use against dengue virus infection. The Journal of infectious diseases. 2015 Feb 1;211(3):394-404.
- [121]Tay MY, Fraser JE, Chan WK, Moreland NJ, Rathore AP, Wang C, Vasudevan SG, Jans DA. Nuclear localization of dengue virus (DENV) 1–4 non-structural protein 5; protection against all 4 DENV serotypes by the inhibitor Ivermectin. Antiviral research. 2013 Sep 1;99(3):301-6.
- [122]Wagstaff KM, Sivakumaran H, Heaton SM, Harrich D, Jans DA. Ivermectin is a specific inhibitor of importin α/β-mediated nuclear import able to inhibit replication of HIV-1 and dengue virus. Biochemical Journal. 2012 May 1;443(3):851-856.
- [123]Martinez-Gutierrez M, Correa-Londoño LA, Castellanos JE, Gallego-Gómez JC, Osorio JE. Lovastatin delays infection and increases survival rates in AG129 mice infected with dengue virus serotype 2. PloS one. 2014 Feb 21;9(2): e87412.
- [124]Dick GW, Kitchen SF, Haddow AJ. Zika virus (II). Pathogenicity and physical properties. Transactions of the royal society of tropical medicine and hygiene. 1952;46(5).
- [125]Macnamara FN. Zika virus: a report on three cases of human infection during an epidemic of jaundice in Nigeria. Transactions of the royal society of tropical medicine and hygiene. 1954 Mar 1;48(2):139-145.
- [126]Meaney-Delman D, Hills SL, Williams C, Galang RR, Iyengar P, Hennenfent AK, Rabe IB, Panella A, Oduyebo T, Honein MA, Zaki S. Zika virus infection among US pregnant travelers—August 2015–February 2016. Morbidity and Mortality Weekly Report. 2016 Mar 4;65(8):211-214.
- [127]Thomas DL, Sharp TM, Torres J, Armstrong PA, Munoz-Jordan J, Ryff KR, Martinez-Quiñones A, Arias-Berríos J, Mayshack M, Garayalde GJ, Saavedra S. Local transmission of Zika virus—Puerto Rico, November 23, 2015–January 28, 2016. Morbidity and Mortality Weekly Report. 2016 Feb 19;65(6):154-158.
- [128]Triunfol M. Microcephaly in Brazil: confidence builds in Zika connection. The Lancet Infectious Diseases. 2016 May 1;16(5):527-528.
- [129]Guzzo CA, Furtek CI, Porras AG, Chen C, Tipping R, Clineschmidt CM, Sciberras DG, Hsieh JY, Lasseter KC. Safety, tolerability, and pharmacokinetics of escalating high doses of ivermectin in healthy adult subjects. The Journal of Clinical Pharmacology. 2002 Oct;42(10):1122-33.
- [130]Devillers J. Repurposing drugs for use against Zika virus infection. SAR and QSAR in Environmental Research. 2018 Feb 1;29(2):103-115.
- [131]Endres DR, Burke D, Bauman NM, Smith RJ. Acyclovir in the treatment of recurrent respiratory papillomatosis: a pilot study. Annals of Otology, Rhinology & Laryngology. 1994 Apr;103(4):301-315.
- [132]Yang J, Dai LX, Chen M, Li B, Ding N, Li G, Liu YQ, Li MY, Wang BN, Shi XL, Tan HB. Inhibition of antiviral drug cidofovir on proliferation of human papillomavirus infected cervical cancer cells. Experimental and therapeutic medicine. 2016 Oct 31;12(5):2965-73
- [133]Blight KJ, Kolykhalov AA, Rice CM. Efficient initiation of HCV RNA replication in cell culture. Science. 2000 Dec 8;290(5498):1972-1974.
- [134]Schreiber GB, Busch MP, Kleinman SH, Korelitz JJ. for the Retrovirus Epidemiology Donor Study. The risk of transfusion-transmitted viral infections. N Engl J Med. 1996;334(26):1685-90.
- [135]He S, Lin B, Chu V, Hu Z, Hu X, Xiao J, Wang AQ, Schweitzer CJ, Li Q, Imamura M, Hiraga N. Repurposing of the antihistamine chlorcyclizine and related compounds for treatment of hepatitis C virus infection. Science translational medicine. 2015 Apr 8;7(282):282ra49-.
- [136]Welzel TM, Nelson DR, Morelli G for the HCV-TARGET Study Group, et al Effectiveness and safety of Sofosbuvir plus ribavirin for the treatment of HCV genotype infection: results of the real-world, clinical practice HCV-TARGET study Gut 2017.
- [137]Luciw PA. Human immunodeficiency viruses and their replication. Fields virology. 1996
- [138]Diaz RS, Shytaj IL, Giron LB, Obermaier B, della Libera Jr E, Galinskas J, Dias D, Huqanter J, Janini M, Gosuen G, Ferreira PA. Potential impact of the antirheumatic agent auranofin on proviral HIV-1 DNA in individuals under intensified antiretroviral therapy: Results from a randomized clinical trial. International journal of antimicrobial agents. 2019 Nov 1
- [139]Roder C, Thomson MJ. Auranofin: repurposing an old drug for a golden new age. Drugs in R&D. 2015 Mar 1
- [140]Walmsley S, Bernstein B, King M, Arribas J, Beall G, Ruane P, Johnson M, Johnson D, Lalonde R, Japour A, Brun S. Lopinavir–ritonavir versus nelfinavir for the initial treatment of HIV infection. New England Journal of Medicine. 2002 Jun 27;346(26).
- [141]Cha Y, Erez T, Reynolds I, Kumar D, Ross J, Koytiger G et al. Drug repurposing from the perspective of pharmaceutical companies. British Journal of Pharmacology. 2017;175(2):168-180.
- [142]Portal DR. Drug Repurposing Portal: News [Internet]. Drug Repurposing Portal | News. [cited 2020, May 29]. Available from: http://www.drugrepurposingportal.com
- [143]Hassig, Smith, Jackson. Creating the World’s Most Comprehensive Drug Repurposing Library [Internet]. Americanlaboratory.com. 2020 [cited 28 May 2020]. Available from: https://www.americanlaboratory.com/913-Technical-Articles/114059-Creating-the-World-s-Most-Comprehensive-Drug-Repurposing-Library/
- [144]Austell J. Home [Internet]. GVN. Jesse Austell http://gvn.org/wp-content/uploads/2017/06/logoGVN.png; 2020 [cited 2020May29]. Available from: https://gvn.org/
- [145]Virology Departments and Institutes – All the Virology on [Internet]. [cited 2020, May29]. Available from: http://virology.net/garryfavweborg.html
- [146]Agrawal P. Drug Discovery and Development: An Insight into Pharmacovigilance. Journal of Pharmacovigilance. 2016;2(3).
- [147]Placchi M, Phillips R. The Benefits and Pitfalls of Repurposing Drugs, Link: https://oliver-dev.s3.amazonaws.com/2018/11/07/21/59/08/240/Benefits%20and%20Pitfalls%20of%20Repurposing%20Drugs.pdf
- [148]Polamreddy P, Gattu N. The drug repurposing landscape from 2012 to 2017: evolution, challenges, and possible solutions. Drug Discovery Today. 2019;24(3).