Proniosomes: A provesicular system in ocular drug delivery
Abstract
The eyes are the only sense organ required for vision. Diseases like glaucoma, cataract, diabetic retinopathy etc. affect the proper functioning of the eyes and sometimes lead to blindness. The treatment of eye disorders is very challenging because of the unique structure of this organ. The traditional treatment approaches are not effective in providing good ocular bioavailability. The provesicular systems are new-generation delivery systems that can improve drug bioavailability and provide therapeutic responses in a controlled manner for desired time. Among all, liposomes are the first such delivery vehicle but due to the lack of stability and the high cost, niosomes were formulated. Niosomes are nanosized vesicles composed of non-ionic surfactants that can encapsulate both lipophilic and hydrophilic drugs. The drawbacks associated with niosomes, like fusion, aggregation, sedimentation, difficulty in sterilization, leaking, etc., gave birth to proniosomes. Proniosomes are more stable and bioavailable than niosomes and liposomes. Proniosomes are dry formulations of hydrophilic carrier particles coated with a water-soluble non-ionic surfactant that, when hydrated, instantly transforms into niosomes. Proniosomes can be used as stable, non-toxic carrier carriers to improve the ocular residence and bioavailability of many drugs. This paper reviewed proniosomes, their biomedical applications and their toxicity in ocular drug delivery.
INTRODUCTION
The eye is the most beautiful and sensitive organ of our body that helps us to perceive and understand our surroundings. It is situated in the orbital cavity of the skull. The eye is spherical in shape, with 24 mm anterior diameter. In terms of drug delivery eye can be divided into four target sites: (a) cornea, (b) anterior and posterior chamber and associated tissue, (c) posterior eye segment (including the retina and posterior cavity), and (d) preocular structure (conjunctiva and eyelids). The cornea, crystalline lens, iris, and pupil make up the anterior part of the eye. Aqueous fluid fills both the anterior and posterior chambers [1].
A growing proportion of people worldwide suffer from ocular illness. Possible significant vision problems caused by some pathological conditions of the eyes, such as diabetic retinopathy, age-related macular degeneration (AMD), HIV infections, and glaucoma may cause complete loss of vision [2]. Eyes disorders are cured or managed using either a topical or systemic delivery of drugs. Although the administration of drugs through the systemic route offers the advantage of delivering the medicine to the eye more conveniently, it has the disadvantage of unexpected side effects and insufficient therapeutic efficacy. The various delivery approaches, as given in Table 1, have been used for ocular administration. The diagrammatic representation is given in Figure 1 depicting the comparison between conventional and novel drug delivery systems in ocular administration. Some conventional forms of drug delivery, like ocular gels, solutions, suspensions, etc., have significant drawbacks in the form of tears turnover, poor corneal permeability, drug loss on eyelids and eyelashes, nasolacrimal drainage, inaccurate dosing associated with eye drops, blinking, and blurred vision. The above-mentioned challenges have raised the demand for innovative approaches to delivering drugs to the eyes [3]. Eye drops are the most common and widely used ocular dosage forms. However, the major drawback of conventional dosage forms in ocular delivery is that only 5% of the drug reaches the target site because of the various ocular barriers present in the eye [4]. Furthermore, the use of nanotechnology and other emerging drug delivery systems is widely regarded as a means of overcoming the drawbacks of conventional dosage forms and avoiding the various obstacles present in the eye [5–7]. The vesicular system encloses the drug within surfactant vesicles to achieve targeted drug delivery at the corneal surface resulting in enhanced bioavailability [8]. Ideal characteristics for vesicular ocular drug delivery include easy penetration of the drug through the corneal membrane, longer residence time in the eye to achieve a desired therapeutic effect, reduced dosing frequency, minimum drug loss, avoidance of blurred vision, and minimal adverse reaction/side effects [9]. Proniosomes are a novel class of drug delivery system that has gained considerable attention in various applications in recent years [10–13]. Proniosomes gained popularity over liposomes and niosomes because of their excellent stability and better bioavailability [14]. This current review discussed various aspects of proniosomes in ocular drug delivery.
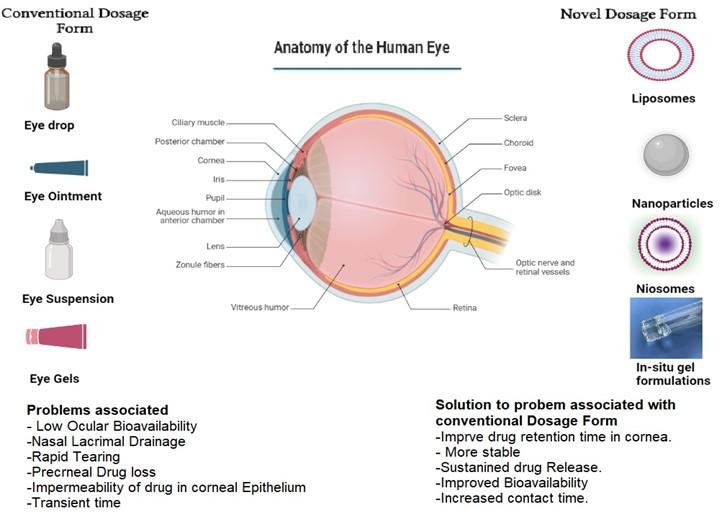
Table 1. Formulation approaches for ocular delivery.
PRONIOSOMES
Proniosomes are a form of lipid-based drug delivery system consisting of a dry mixture of surfactants and cholesterol that form vesicles in an aqueous phase. Proniosomes are solid colloidal particles that can be quickly hydrated before use to make aqueous niosome dispersions that are comparable to those made using more laborious traditional procedures [15]. The proniosomes reduce the issues with niosomes' physical stability, including aggregation, fusion, and leakage. Additionally, they make transportation, distribution, storage, and dosage more convenient. The shape, particle size, particle size distribution, and drug release of the proniosome-derived niosomes are superior to those of traditional niosomes [16]. Based on their processing, proniosomes can be classified as either dry granular or liquid crystalline. Dry granular proniosomes are prepared by coating a water-soluble carrier (maltodextrin or sorbitol) with a surfactant. Based on the type of carrier used, dry granular proniosomes are further classified into two types of maltodextrins and sorbitol dry granular proniosomes [17]. Dry granular proniosomes provide advantages such as improved stability and convenient storage. On the other hand, liquid crystalline proniosomes possess a highly organized liquid crystalline structure, which enhances drug encapsulation, sustained release, and permeation through the biological membranes [18].
Proniosomes are designed to improve the solubility and bioavailability of drugs and were investigated for a range of applications, including ocular delivery [19]. Proniosomes exhibit favorable characteristics for gene delivery as they are easy to formulate, economical, stable, and nontoxic because of non-ionic surfactant inclusion [20]. Drug targeting is one of the most advantageous properties of proniosomes. Proniosomes are employed in transdermal drug delivery systems to deliver hypertension drug captopril [21]. Frusemide is also delivered non-invasively by proniosomes [22]. In hormonal therapy, levonorgestrel, an emergency contraceptive, has been tested for transdermal distribution using proniosomes [23]. Peptide delivery is also possible with proniosomes. Oral peptide breakdown by gastrointestinal enzymes is problematic [24]. Nevertheless, peptides were shielded successfully using niosomes against peptide degradation in the digestive tract [25]. An entrapment inside the vesicles considerably boosted the stability of the peptide when it was given orally as a vasopressin derivative in niosomes [26]. Proniosomes have been widely used to deliver antibiotics and anti-inflammatory agents [27]. There are different methods available for the preparation of proniosomes, including the slurry method, coacervation phase separation method and slow spray coating method. In the slurry method, a non-ionic surfactant is dissolved in a volatile organic solvent to form a slurry, to which the drug is added followed by carrier material like lactose or mannitol. The organic solvent is evaporated under reduced pressure resulting in dry proniosomal powder [28]. In the coacervation method proniosomes are prepared by mixing non-ionic surfactant and carrier in a solvent and then adding a coacervation-inducing agent such as calcium or magnesium ions which leads to the formation of coacervate [29]. The slow spray coating method involves the use of a spray dryer to coat the proniosomes with a thin layer of a polymer or other coating material. Proniosomes produced by the spray coating method are stable. However, it is a tedious and time-consuming process. Each method offers its advantages and can be chosen based on specific requirements of the formulation [30].
PRONIOSOMES IN OCULAR DRUG DELIVERY
The eye's unique physiological and anatomical features make the ocular drug delivery system a challenging field. The eye has a limited surface area, and the tear film and the blood-retinal barrier limit the penetration of drugs into the eye [31]. Thus, developing a drug delivery system that can overcome these barriers and deliver drugs effectively to the eye is critical [32]. Proniosomes offer a promising solution for ocular drug delivery due to their unique properties [33]. Proniosomes are formulated using a range of surfactants, including non-ionic, cationic, and anionic surfactants and other excipients, as given in Table 2. The physiochemical parameters of drugs and the intended proniosomes features determine the surfactant to be used. Surfactant and cholesterol mixture is typically dried and reconstituted with an aqueous phase to form Niosomes. Niosomes can then be further processed to form proniosomes by lyophilization or spray drying [34]. Proniosomes can protect drugs from degradation by enzymes and pH changes in the body, improving their stability and shelf-life. The surfactant in proniosomes also helps to increase the solubility of the drug, which can improve its bioavailability [35].
Table 2. Commonly used excipients.
Factor affecting the formulation of proniosomes
The formulation of proniosomes depends on several processing factors such as surfactant chain length, cholesterol content, pH of the hydration medium, total lipid concentration and charge of the lipids as shown in Figure 2 and discussed below-
a) Surfactant chain length: A longer alkyl chain length results in greater drug entrapping effectiveness. For example, Spans are widely used in the production of proniosomes. The order of entrapment efficiency for surfactant is Span 60(C18)>Span 40(C16) >Span 20(C12) [36].
b) Cholesterol content: Depending on the type of surfactant or its concentration in the formula, cholesterol either improves or decreases the percentage of encapsulation efficiency. A higher amount of cholesterol in the formulation increases the rigidity of the bilayer leading to a decrease in the drug release from the encapsulated formulation.
c) pH of the hydration medium: A decrease in the pH of the hydration medium leads to an increase in the percentage encapsulation efficiency.
d) Total lipid concentration: As the concentration of lipids rises, the fraction of lipids participating in encapsulation decreases.
e) Charge of the lipids: The incorporation of a positive charge (Stearyl amine and stearyl pyridinium chloride) or a negative charge (diacetyl phosphate and phosphatidic acid) decreases the percentage encapsulation efficiency of proniosomes [37].
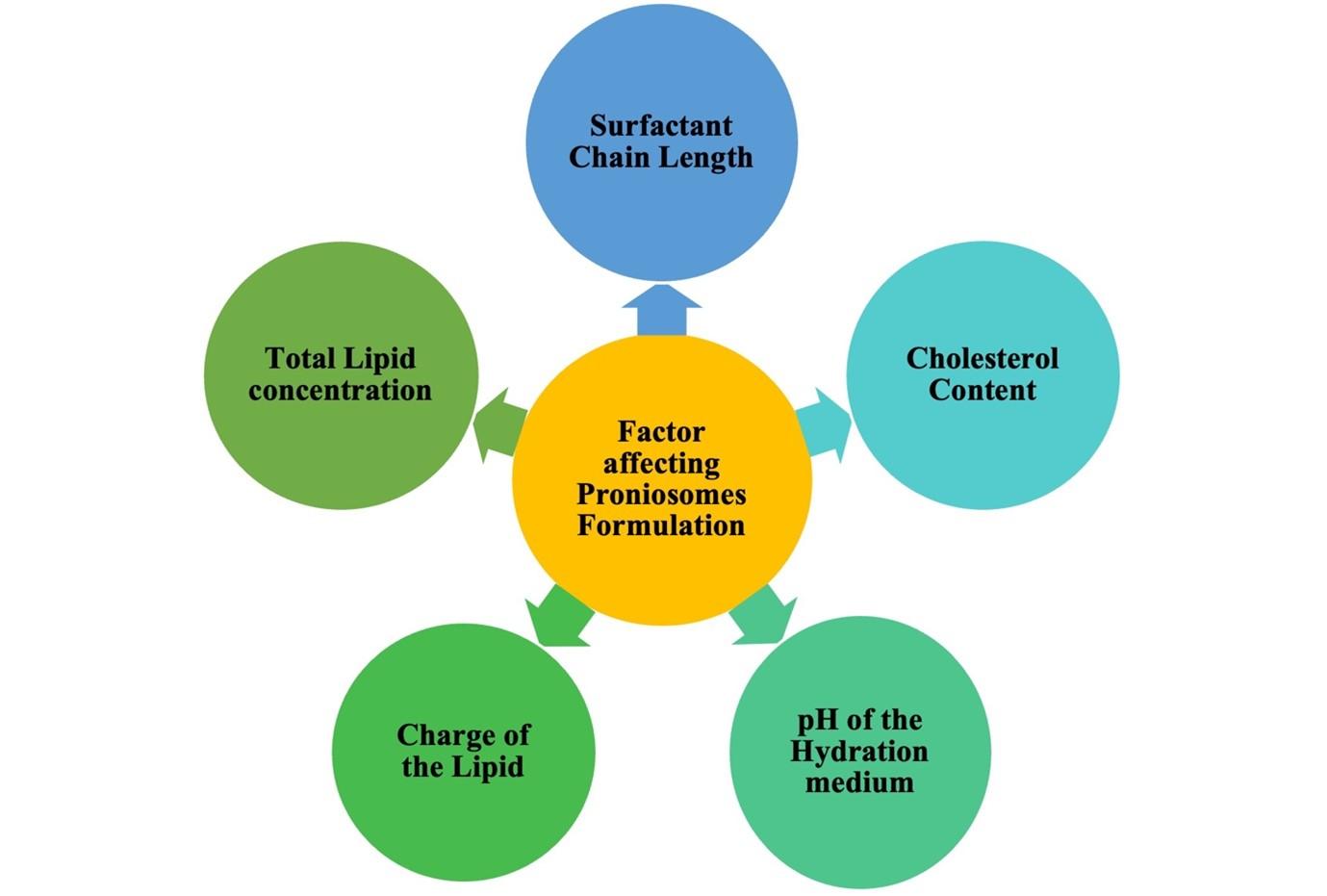
EVALUATION PARAMETERS OF OCULAR PRONIOSOMES
The evaluation of proniosomes is essential to ensure their quality, safety, and effectiveness in delivering drugs to the eyes. Several key parameters are mentioned in Table 3 and below, which are required in evaluating the performance of proniosomes in the ocular route.
Table 3. Evaluation parameter of proniosomes.
Surface morphology
It can be determined using various microscopy methods like scanning electron microscopy (SEM) or transmission electron microscopy (TEM). Such techniques allow visualization of the size, shape, and surface characteristics by providing high-resolution images of proniosomes [38]. The surface morphology of proniosomes can also be determined using fluorescence microscopy by incorporating fluorescent dyes in proniosomes to provide results of the size, shape, and distribution of proniosomes [39]. The vesicle size of proniosomes intended for ocular delivery is an important parameter that can influence their stability, drug release kinetics and ocular tissue penetration. Vesicle size range for proniosomes used in ocular delivery should be less than 10 μm. For example, Fouda et al. 2018 determined the surface morphology of dorzolamide proniosomes using TEM after negative staining with potassium phophotungustate [40]. TEM image showed that the proniosomes formed were spherical in shape [40].
Abaoli et al. (2020) investigated the surface morphology of curcumin proniosomes by SEM and TEM [41]. TEM image confirmed the smooth and spherical shape of proniosomes without aggregation. SEM results showed that the surface was smoother and more compact with no apparent pores suggesting good entrapment efficiency and a vesicle size of 212 nm [41].
Encapsulation efficiency
To determine encapsulated drugs in the formulation, it is necessary to isolate free drugs using methods like column chromatography, dialysis, ultra-centrifugation, freeze-thawing, gel filtration, etc. The effectiveness of trapped drugs can be assessed in two ways: proniosomal vesicle destruction with a triton (0.1%) or propane (50%) and identification of the drug trapped inside [42]. Alternatively, untapped drugs can be measured after the vesicle has been destroyed. The amount of drug entrapped can calculate by using the formula:
EE (%) = [(Ct - Cf)/ Ct] × 100
Where, Ct = the concentration of total drug
Cf= the concentration of the unentrapped drug
The ideal entrapment efficiency can vary depending on the specific drug, therapeutic goal, and formulation requirements. Generally, a higher entrapment efficiency (>50% or above) is desirable for effective ocular delivery. High entrapment efficiency ensures that a significant amount of drug is entrapped inside the vesicle leading to efficient drug delivery to the target ocular tissues and reducing potential drug loss or wastage.
Rheological Measurement
Rheological measurements of proniosomes for ocular delivery are valuable for understanding their physical behaviour, stability, and suitability for application to the ocular surface. Rheological measurements such as viscosity can provide information about the formulation’s consistency and its ability to spread and adhere to the ocular surface. For ocular delivery, the viscosity should be low enough to facilitate easy spreading and administration on the ocular surface but high enough to ensure sufficient retention and contact time. Viscosity for ocular preparation should range between 2 to 3 mPa.s. [43,44].
Isotonicity and osmolarity
Isotonicity of the ocular formulations is important to minimize potential discomfort or irritation to the ocular surface. For ocular application, the ideal isotonicity range for proniosomes should be between 250-350 mOsm/kg as this range is close to the osmolarity of tears which is 308 mOsm/kg. Formulating proniosomes within this isotonic range help to minimize the risk of irritation or adverse effect on the ocular tissue. An osmolarity of less than 100 mOsm/kg or greater than 640 mOsm/kg is considered an eye irritant [44,45].
In vitro studies
The drug release from proniosomes can be determined using a dialysis membrane, Franz diffusion cell, reverse dialysis, and USP dissolution apparatus type 1. It is possible to analyze the drug release kinetics from the in-vitro drug release data. The release date is fitted into various kinetic models like- zero order, first order, Higuchi, Korsmeyer-Peppas, and Hixson Crowell models to find the order and mechanisms of drug release [46]. The “best-fit model” for drug release/dissolution can be selected based on a variety of factors like the coefficient of determination (R2), R2Adjusted, Sum of squares of residue (SSR), Akaike information criteria (AIC), Mean square error (MSE), and Correlation coefficient (R) [47].
Ocular irritation
The Draize test is an effective and accurate method for accessing the irritation potential of proniosomes. It involves applying a test substance to the eyes of an animal, usually a rabbit (because of their wide eyes, ease of handling, and well-described anatomy). The responses are observed to determine any irritation caused due to proniosomes. The Draize test evaluates ocular irritation based on the study score. The score ranges from 0 to 3 for no irritation to redness and highest irritation. Nowadays, non-animal approaches such as computer modelling, and in-vitro experiments employing human cells and tissues are being used [48]. Eldeeb et al., 2019 performed the Draize test on male albino rabbits which were subjected to Brimonidine Tartrate proniosomal formulation and marketed formulation (Alphagan) to evaluate irritancy [49]. The marketed formulation showed irritancy for the first 1 h whereas the test formulation showed no sign of irritation indicating the safety of the formulation for ocular delivery [49].
Zeta potential
The zeta potential determines both the stability of a colloidal system and a vesicle's surface charge. Zeta potential measures the electrical potential at the shear plane around a particle or vesicle [50]. The Zeta potential of proniosomes can affect drug loading, stability, and drug release characteristics. The ideal zeta potential for proniosomes used in ocular delivery should be around +30 mv. Higher zeta potential prevents particle aggregation and coalescence, ensuring dispersion stability. A low zeta potential indicates a risk of aggregation. Zeta potential can be measured using a Malvern zeta sizer [51].
Stability
According to ICH recommendations, Stability studies on proniosomes for dry powder meant for reconstitution should be conducted as per climatic conditions and climatic zones (WHO, 1996). For accelerated stability, a temperature and relative humidity (RH) of 40 0C/75% RH is required, and for long-term studies, 25 0C/ 60% RH is required for Zone 1 and Zone 2, respectively, whereas Zone 3 and Zone 4 must maintain a condition of 30 0C /65% RH and measured over time for in vitro drug release, poly dispersibility index (PDI), zeta potential, pH, sterility, pyrogenicity, etc [52]. Whereas stability studies for proniosomal gel should be conducted at a refrigeration temperature of 2-80C, room temperature of 25±0.5° and an elevated temperature of 45±0.50° for 90 days. Conduction stability studies are vital to ensure the safety and efficacy of the product during its shelf life [53]. According to a stability study performed by Li et al. (2014) [54] on Tacrolimus-derived proniosomes for topical ocular delivery, studies were performed at 40 ± 2 0C/75 ± 5%RH; 4±2 0C/75 ± 5%RH; 25 ± 2 0C/60 ± 5%RH by storing proniosomes in a glass container. Physical stability studies were carried out to investigate the leakage of drug from the proniosomes during storage after 1,2 and 3 months of storage. Results indicated that proniosomes remain visually unchanged and entrapment efficiencies slightly reduce from 95% to 93% after 3 months of storing at 4 0C [54].
Toxicity of proniosomes
The eye is a very sensitive and very useful organ of humans. Therefore, attention should be given to the safety of any dosage form administered to the eye. There are limited research reports on the toxicity of proniosomes. Researchers need to assess the possible toxicity of ocular formulations and the materials utilized to prepare these formulations during repeated and prolonged application in the eye before their clinical use and commercial production. Most of the biocompatibility and safety studies of proniosomes are performed on animal eyes. However, the structure of animal eyes is quite different from human eyes. Unfortunately, the results of these preclinical studies are sometimes not validated in humans. Most preclinical findings concluded no signs of toxicity and altered pharmacological effects of proniosomes in the eyes. Proniosomes are composed of surfactants and cholesterol. Surfactants were used in proniosomal formulation to increase drug permeability through the cell membrane [55]. However, the chemical nature of surfactants imparts toxicity. Various studies have shown that the toxicity of proniosomes in ocular delivery depends on several factors, including the type of surfactant used, the concentration of surfactant, and the duration of exposure. A study by Govindarajan et al., 2022 [56] has shown that the ester derivative surfactant is harmful compared to the ether derivative. Surfactants like tween 80, can cause ocular irritation and damage to the cornea and conjunctiva when used at high concentrations [56]. CTAB (cetyltrimethylammonium bromide), a commonly used cationic surfactant, has been associated with cytotoxicity and disrupts cell membranes [57,58]. The most preferred constituents of proniosomes Span 60 and cholesterol are safe and do not produce any ocular toxic effect [59]. Overall potential toxicity of surfactants should be carefully considered, and at the same time, it is essential to conduct thorough safety evaluations of proniosomes before using them for ocular delivery in humans [60].
APPLICATION OF PRONIOSMOMES IN OCULAR DISEASES
Proniosomes have gained significant attention in the pharmaceutical field due to their potential application in various medical fields. One of those areas is the treatment of various ocular diseases as shown in Table 4.
Glaucoma
Glaucoma is an eye disorder marked by elevated intraocular pressure that eventually results in optic nerve damage, leading to vision loss or blindness. Glaucoma is the primary cause of blindness worldwide [61]. Dorzolamide (Carbonic anhydrase inhibitor) is used in the treatment of glaucoma. The proniosomes of dorzolamide showed decreased intraocular pressure with enhanced therapeutic efficacy in the in-vivo studies in male albino rats [62,63]. Brimonidine tartrate (α-2 adrenergic agonist) proniosomes decrease the production of aqueous humour and reduce the ischemia-induced optic nerve damage by enhancing the ocular bioavailability of the drug [49]
Conjunctivitis
Infection of the conjunctiva is known as conjunctivitis, commonly referred to as pink eyes. Conjunctiva is a thin transparent membrane covering the white part of the eyes. Proniosomes may use to encapsulate and deliver anti-inflammatory and antibiotic drugs directly to the eye to treat conjunctivitis [64,65]. Various studies have demonstrated the benefits of proniosomes conjunctivitis treatment. Lomefloxacin-proniosomal formulation was found effective in reducing the severity of bacterial conjunctivitis in rabbits [66]. In another study, Levofloxacin-loaded proniosomal formulation showed increased ocular residence time by providing sustained drug release [67].
Table 4. Application of proniosomes in ocular delivery.
Fungal infection
Fungal keratitis/mycotic/keratomycosis is a severe eye infection that affects the cornea of the eyes leading to corneal damage and vision loss. Candida parapsilosis, candida albicans, candida tropicalis, and candida glabrata mainly causes ocular fungal infections [68]. Proniosomes may be used to encapsulate and deliver antifungal medication for fungal keratitis. One of the studies indicated that proniosomes loaded with Amphotericin B were able to effectively treat fungal keratitis in rabbits [69].
Uveitis
Uveitis, or chorioretinitis, is an inflammation of the middle layer of the eyes causing inflammation of eyes. Proniosomes can be used to encapsulate and deliver anti-inflammatory medication, such as corticosteroids or NSAIDS, directly to the eye to treat uveitis [70]. Tacrolimus-loaded proniosomes gave higher precorneal permeation and retention in an in vitro study in rabbit cornea for 21 days [71].
SCALE-UP CHALLENGES OF PRONIOSOMES
Despite the development of proniosomes and nanoparticles in general being successful, there are still many obstacles to overcome, such as complicated regulatory issues, scale-up practicability, and reproducibility. As a result, producing nanomaterials on a large scale might be difficult [59]. To produce proniosomes on an industrial scale for commercial use, it is necessary to integrate new methods and technology transfer. However, any preparation procedure may fail to translate from a laboratory scale to an industrial scale due to process restrictions in small-scale preparation. Proniosomes’ characteristics, such as particle size, drug encapsulation, process leftover materials, stability, and surface properties, are most affected by scaling up. Moreover, the scale-up process may decrease the drug loading in the proniosomes. Therefore, a well-designed scale-up procedure is required which can guarantee the effectiveness, affordability, and fast production of these nanomedicines [59]. The stability of the materials used for the production and the use of toxic solvents (such as the use of chloroform or dichloromethane as an organic phase) are some of the process limitations. Therefore, new techniques utilizing aqueous solvents or solvents with low toxicity must be developed for the pharmaceutical industry to produce nanomedicines.
It is critical to demonstrate the ability to transfer the technology to a development facility or contract manufacturing business where a practical, scalable, and cost-effective process can be established to produce large batch sizes under good laboratory practices (GLP) and ultimately good manufacturing practices (GMP) conditions [72].
Due to their simple fabrication method and flexibility in drug delivery, proniosomes have the potential to be manufactured on a large scale. Proniosomes were investigated as potential replacements for liposomes and other carrier systems for entrapping both hydrophobic and hydrophilic or polar and nonpolar pharmaceuticals. Proniosomes also have the advantage of minimal toxicity due to their non-ionic nature and the lack of any specific production or processing requirements. Additionally, it is an easy approach for producing proniosomes regularly and in big quantities without the use of undesirable solvents [73].
FUTURE PROSPECTS OF PRONIOSOMES
Proniosomes have been proven as an efficient delivery method that offers several benefits over conventional delivery methods in the form of better stability, bioavailability, and targeted delivery of therapeutic molecules. Further potential future applications of proniosomes include their use in gene therapy, vaccine delivery, and cancer treatment. Moreover, using proniosomes in combination with other drug delivery technologies, such as nanotechnology could lead to the formation of even more effective drug delivery systems. However, the formulation of proniosomes may present several challenges due to their complex nature and the need for precise control over their properties to ensure efficient drug delivery. Scaling up proniosomes from laboratory to large-scale production can be difficult, and it is important to ensure consistent product quality for regulatory approval. Tackling these challenges will be essential to developing and adopting proniosomes as a drug delivery system. Some patented formulations of proniosomes in various delivery systems are given in Table 5.
Table 5. Patented formulation of proniosomes.
CONCLUSION
In conclusion, proniosomes represent a promising drug delivery platform for ocular drug delivery (Figure 3). These are essentially dry formulations that consist of a blend of surfactant and carrier material. The ability of proniosomes to improve drug absorption and retention in the eyes, along with their ease of handling and stability, makes them an attractive option for developing novel ocular therapies. These are widely applicable for the delivery of various drugs, including antibiotics, anti-cancer agents, and anti-inflammatory drugs. Furthermore, research is needed to optimize the formulation and delivery of proniosomes for clinical use.
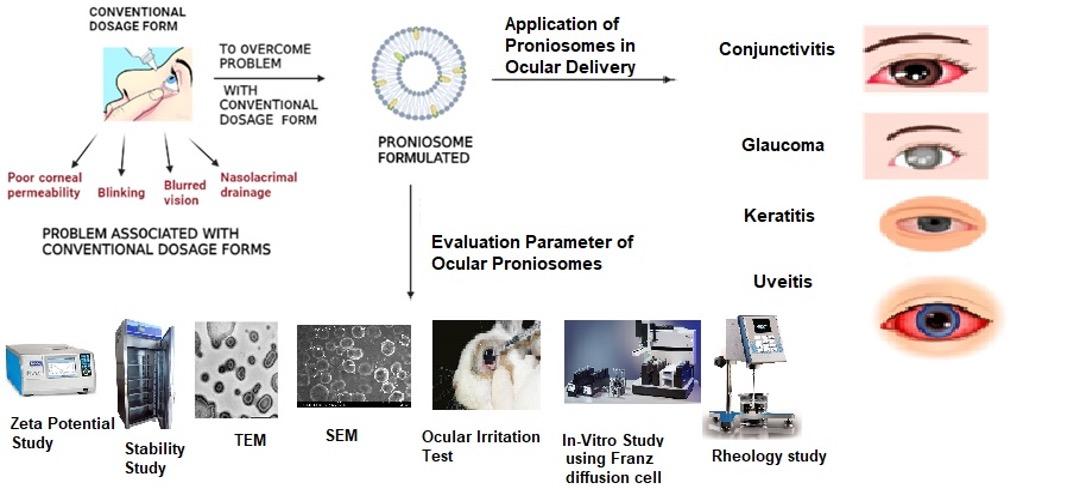
ACKNOWLEDGEMENT
None
AUTHOR CONTRIBUTIONS
This manuscript was drafted by MNK and NN. MYA provided supervision throughout the manuscript preparation. Other authors contributed directly or indirectly to the revision and publication of this manuscript.
CONFLICTS OF INTEREST
There is no conflict of interest among the authors.
References
- [1]Irsch K, Guyton DL. Anatomy of Eyes. In: Encyclopedia of Biometrics, 2009. Springer US: 11–16.
- [2]Urtti A. Challenges and obstacles of ocular pharmacokinetics and drug delivery. Adv Drug Deliv Rev. 2006;58(11):1131–1135.
- [3]Jumelle C, Gholizadeh S, et al. Advances and limitations of drug delivery systems formulated as eye drops. J Control Release. 2020; 321:1–22.
- [4]Souto EB, Dias-Ferreira J, et al. Advanced Formulation Approaches for Ocular Drug Delivery: State-Of-The-Art and Recent Patents. Pharmaceutics. 2019;11(9).
- [5]Barar J, Asadi M, et al. Ocular drug delivery; Impact of in vitro cell culture models. J Ophthalmic Vis Res. 2014;4(4):238–252.
- [6]Gaudana R, Ananthula HK, et al. Ocular Drug Delivery. The AAPS Journal 2010 12:3. 2010;12(3):348–360.
- [7]Silva MM, Calado R, et al. Chitosan Nanoparticles as a Mucoadhesive Drug Delivery System for Ocular Administration. Marine Drugs 2017, Vol 15, Page 370. 2017;15(12):370.
- [8]Patel PB, Shastri DH, et al. Ophthalmic drug delivery system: Challenges and approaches. Systematic Reviews in Pharmacy. 2010;1(2):113–120.
- [9]Sayed S, Abdelmoteleb M, et al. Effect of Formulation Variables and Gamma Sterilization on Transcorneal Permeation and Stability of Proniosomal Gels as Ocular Platforms for Antiglaucomal Drug. AAPS PharmSciTech. 2020;21(3):1–13.
- [10]Sakran W, Abdel-Rashid RS, et al. Ethosomal gel for rectal transmucosal delivery of domperidone: design of experiment, in vitro, and in vivo evaluation.
- [11]Durai Prof RD. Drug delivery approaches of an antiviral drug: A comprehensive review. Asian Journal of Pharmaceutics (AJP). 2015;1–12.
- [12]Demirbolat Melike Gulen AEPOO. New Approach to Formulate Methotrexate-Loaded Niosomes: In Vitro Characterization and Cellular Effectiveness. Journal of Pharmaceutical Innovation , 2021;1–16.
- [13]Ammar H.O. GM-NIM. Proniosomes as a carrier system for transdermal delivery of tenoxicam. International Journal of Pharmaceutics, 405(1-2), 142–152.
- [14]Varsha VR, Savitha SK., et al. Proniosomes: As a potential drug delivery system. 2019; 1-12.
- [15]Bonthagarala B, Sivaprasad SN V, et al. Proniosomes: A novel approach to vesicular drug delivery system. International journal of drug discovery,2013; 85-90.
- [16]Ajay Singh S, Chaudhari Y, et al. Proniosomes: A recent advancement in vesicular drug delivery system. World journal of pharmaceutical research, 4(4), 2015; 202-206.
- [17]Rajkumar J, Gv R, et al. Recent update on proniosomal gel as topical drug delivery system. Asian Journal of Pharmaceutical and Clinical Research. 2019;12(1):54.
- [18]Muzzalupo R. Niosomes and proniosomes for enhanced skin delivery. Percutaneous Penetration Enhancers Chemical Methods in Penetration Enhancement: Nanocarriers. 2016;147–160.
- [19]Hema Sagar G, Arunagirinathan MA, et al. Self-assembled surfactant nano-structures important in drug delivery: A review. Indian J Exp Biol. 2007;45:133–159.
- [20]Pardakhty A, Moazeni E., et al. Nano-niosomes in drug, vaccine and gene delivery: a rapid overview Nanoniosomes review 2. Nanomedicine journal, 2013;1-12.
- [21]Gupta A, Kumar Prajapati S, et al. Design and Development of a Proniosomal Transdermal Drug Delivery System for Captopril. Tropical journal of pharmaceutical research, 2007; 6(2), 687-693.
- [22]Azeem A, JN. Feasibility of Proniosomes-Based Transdermal Delivery of Frusemide: Formulation Optimization and Pharmacotechnical Evaluation. Pharmaceutical Development and Technology, 2008; 13(2), 155–163.
- [23]Kumhar SK, Jain SK, et al. Provesicular Transdermal Drug Delivery System Of Ethinylestradiol And Levonorgestrel For Contraception And Hormone Replacement Therapy. Indian J Pharm Sci.65(6):620.
- [24]Khatoon M, Shah KU, et al. Proniosomes derived niosomes: recent advancements in drug delivery and targeting. https://doi.org/101080/1071754420171384520. 2017;24(2):56–69.
- [25]Manosroi A, Khanrin P, et al. Entrapment enhancement of peptide drugs in niosomes. http://dx.doi.org/103109/02652040903131293. 2010;27(3):272–280.
- [26]Akhilesh D, Faishal G, et al. Comparative Study of Carriers Used in Proniosomes. International journal of pharmaceutical and chemical sciences, 2012;164–173.
- [27]Alsarra IA. Evaluation of proniosomes as an alternative strategy to optimize piroxicam transdermal delivery. 2009;26(3):272–278.
- [28]Suryawanshi SS, Patil PP, et al. Proniosomes: modern drug delivery system. Pharmaceutical resonance, 2021; 41-56.
- [29]Radha G, Rani Ts, et al. A review on proniosomal drug delivery system for targeted drug action. J Basic Clin Pharm. 2013;4(2):42.
- [30]SrividyaVardhaniCH NB. View of Proniosomal gel- An effective approach for topical and transdermal drug delivery. 2016;179–183.
- [31]Achouri D, Alhanout K, et al. Recent advances in ocular drug delivery. Taylor and francis, 2013;39(11):1599– 1617.
- [32]Bachu RD, Chowdhury P, et al. Ocular Drug Delivery Barriers—Role of Nanocarriers in the Treatment of Anterior Segment Ocular Diseases. Pharmaceutics.2018;10(1):28.
- [33]Sankar V, Jailani S, et al. Proniosomes as drug carriers Cellular imaging and folate receptor targeting delivery View project Green synthesis of metal & metal oxide nanoparticles for analysing their biological & electrical assay. Pakistan Journal of Pharmaceutical Sciences. 2010;23(1):103–107.
- [34]Subodh DAJMSC; GPJSSJ. Niosomes: The ultimate drug carrier. Drug Invention Today. 2010;72–77.
- [35]Goudanavar P, Tavari P, et al. Review on Proniosomes. International journal of pharmacy and pharmaceutical research, 2019; 345-356.
- [36]Dakhilesh R, Pprabhu D, et al., Development and optimization of proniosomes for oral delivery of glipizide. Int J Pharm Pharm Sci. 2012; 4(3), 307-314.
- [37]Kumar K, Rai AK, et al. Development and evaluation of proniosomes as a promising drug carrier to improve transdermal drug delivery.International research journal of pharmacy, 2011; 2(11),112-123.
- [38]Yousuf Muhammad AM. Ketotifen Fumarate and Salbutamol Sulphate Combined Transdermal Patch Formulations: In vitro release and Ex vivo Permeation Studies. Indian Journal Of Pharmaceutical Sciences. 2013;569–577.
- [39]Thakur Reena AKMSSM. Proniosomal transdermal therapeutic system of losartan potassium: development and pharmacokinetic evaluation. Journal of Drug Targeting,2009; 17(6), 442–449.
- [40]Fouda NH, Abdelrehim RT, et al. Sustained ocular delivery of dorzolamide-HCL via proniosomal gel formulation: In-vitro characterization, statistical optimization, and in-vivo pharmacodynamic evaluation in rabbits. Drug Deliv. 2018;25(1):1340–1349.
- [41]Aboali FA, Habib DA, et al. Curcumin-loaded proniosomal gel as a biofreindly alternative for treatment of ocular inflammation: In-vitro and in-vivo assessment. Int J Pharm. 2020;589. doi:10.1016/j.ijpharm.2020.119835.
- [42]Rahman SA, Abdelmalak NS, et al. Formulation of tretinoin-loaded topical proniosomes for treatment of acne: in-vitro characterization, skin irritation test and comparative clinical study. Drug Deliv. 2015;22(6):731–739.
- [43]Jurišić Dukovski B, Juretić M, et al. Functional ibuprofen-loaded cationic nanoemulsion: Development and optimization for dry eye disease treatment. Int J Pharm. 2020;576:118979.
- [44]Ahmed S, Amin MM, et al. Ocular Drug Delivery: a Comprehensive Review. AAPS PharmSciTech. 2023;24(2):66.
- [45]Singh M, Bharadwaj S, et al. Therapeutic nanoemulsions in ophthalmic drug administration: Concept in formulations and characterization techniques for ocular drug delivery. Journal of Controlled Release. 2020;328:895–916.
- [46]Panotopoulos GP, Haidar ZS. Mathematical Modeling for Pharmaco-Kinetic and-Dynamic Predictions from Controlled Drug Release NanoSystems: A Comparative Parametric Study. 2019; 312-328. doi:10.1155/2019/9153876.
- [47]Lokhandwala H, Deshpande A, et al. Kinetic modelling and dissolution profiles comparision: an overview drug release kinetic models, model dependent method, model independent method, statistical model, pairwise comparison. Int J Pharm Bio Sci. 2013;4(1):728–737.
- [48]Tayel SA, El-Nabarawi MA, et al. Promising ion-sensitive in situ ocular nanoemulsion gels of terbinafine hydrochloride: Design, in vitro characterization and in vivo estimation of the ocular irritation and drug pharmacokinetics in the aqueous humor of rabbits. Int J Pharm. 2013;443(1–2):293–305.
- [49]Emad Eldeeb A, Salah S, et al. Proniosomal gel-derived niosomes: an approach to sustain and improve the ocular delivery of brimonidine tartrate; formulation, in-vitro characterization, and in-vivo pharmacodynamic study. Drug Deliv. 2019;26(1):509–521.
- [50]Dai Wenting ZD cunxian. Preparation and characteristics of oridonin-loaded nanostructured lipid carriers as a controlled-release delivery system. Journal of Microencapsulation, 2010; 27(3), 234–241.
- [51]Ramkanth S, Chetty CM, et al. Development, characterization & invivo evaluation of proniosomal based transdermal delivery system of Atenolol. Futur J Pharm Sci. 2018;4(1):80–87.
- [52]Kumar GP, Rajeshwarrao P. Nonionic surfactant vesicular systems for effective drug delivery—an overview. Acta Pharm Sin B. 2011;1(4):208–219.
- [53]Nagalakshmi S. DN, TJ , et al. NiosomesinOcularDrugDeliverySystem (1). IntJPharmSci. 2015;61–66.
- [54]Li Q, Li Z, et al. Proniosome-derived niosomes for tacrolimus topical ocular delivery: In vitro cornea permeation, ocular irritation, and in vivo anti-allograft rejection. European Journal of Pharmaceutical Sciences. 2014;62:115–123.
- [55]Saah Safiah et al. Cytotoxic Effect of Surfactants Used in Self-Microemulsifying Drug Delivery Systems (SMEDDS) on Normal and Cancer Gastrointestinal Cell Lines. Latin american journal of pharmacy. 2018; 530- 542.
- [56]Govindarajan S, Swamivelmanickam M, et al. A Comprehensive Study on Provesicular Drug Delivery System: Proniosomal Gel. Indian J Pharm Sci. 2022;84(1):1–13.
- [57]Pardakhty A, Shakibaie M, et al. Preparation and evaluation of niosomes containing autoclaved Leishmania major: a preliminary study. J Microencapsul. 2012;29(3):219–224.
- [58]Schachter D. The source of toxicity in CTAB and CTAB-stabilized gold nanorods. 2013; 226-239.
- [59]Maiti S, Paul S, et al. Nanovesicular formulation of brimonidine tartrate for the management of glaucoma: in vitro and in vivo evaluation. AAPS PharmSciTech. 2011;12(2):755–763.
- [60]Sabale V, Charde M, et al. Recent Developments in Proniosomal Transdermal Drug Delivery: An Overview. Curr Drug Deliv. 2022;20(6):683–693.
- [61]Schuster AK, Erb C, et al. The Diagnosis and Treatment of Glaucoma. Dtsch Arztebl Int 2020; 631-640.
- [62]Fouda NH, Abdelrehim RT, et al. Sustained ocular delivery of dorzolamide-HCL via proniosomal gel formulation: In-vitro characterization, statistical optimization, and in-vivo pharmacodynamic evaluation in rabbits. Drug Deliv. 2018;25(1):1340–1349.
- [63]Sayed Sinar AMMM. Effect of Formulation Variables and Gamma Sterilization on Transcorneal Permeation and Stability of Proniosomal Gels as Ocular Platforms for Antiglaucomal Drug. AAPS PharmSciTech,2020; 21(3),1-13.
- [64]Azari AA, Barney NP. Conjunctivitis: A Systematic Review of Diagnosis and Treatment. JAMA. 2013;310(16):1721–1730.
- [65]Azari AA, Arabi A. Conjunctivitis: A Systematic Review. J Ophthalmic Vis Res. 2020;15(3):372.
- [66]Khalil RM, Abdelbary GA, et al. Design and evaluation of proniosomes as a carrier for ocular delivery of lomefloxacin HCl. http://dx.doi.org/103109/0898210420161167737. 2016;27(2):118–129.
- [67]Raj K. Dhangar SSMPNR. View of Formulation and Evaluation of Proniosomal Gel in Ocular Drug. Current research in pharamceutical sciences. 2012;42–47
- [68]Ahmed S, Amin MM, et al. Ocular Drug Delivery: a Comprehensive Review. AAPS PharmSciTech. 2023;24:3.
- [69]Baig Parvez Reesha WM. View of FORMULATION AND DEVELOPMENT OF PRONIOSOMAL GEL FOR TOPICAL DELIVERY OF AMPHOTERICIN B | International Journal of Pharmacy and Pharmaceutical Sciences. International Journal of Pharmacy and Pharmaceutical Sciences. 2021;37–49.
- [70]Sève P, Cacoub P, et al. Uveitis: Diagnostic work-up. A literature review and recommendations from an expert committee. Autoimmun Rev. 2017;16(12):1254–1264.
- [71]Li Q, Li Z, et al. Proniosome-derived niosomes for tacrolimus topical ocular delivery: In vitro cornea permeation, ocular irritation, and in vivo anti-allograft rejection. European Journal of Pharmaceutical Sciences. 2014;62:115–123.
- [72]Ahmad, M. Z., Mohammed, A. A., et al. Technology overview and drug delivery application of proniosome. Pharmaceutical Development and Technology, 2016; 22(3), 302–311.
- [73]Khatoon M, Ullah Shah K, et al. Drug Delivery Proniosomes derived niosomes: recent advancements in drug delivery and targeting. 2017; 712-726.
- [74]Dave VS. Formulation Approaches for Ocular Drug Delivery. In: Nano-Biomaterials For Ophthalmic Drug Delivery. Springer International Publishing, 2016; 147–175.
- [75]Gote V, Sikder S, et al. Ocular drug delivery: Present innovations and future challenges. Journal of Pharmacology and Experimental Therapeutics. 2019;370(3):602–624.
- [76]Pandita A, Sharma P. Pharmacosomes: An Emerging Novel Vesicular Drug Delivery System for Poorly Soluble Synthetic and Herbal Drugs. ISRN Pharm. 2013; 1–10
- [77]Mori Dhaval, Jatin devani, et al. Formulation, and optimization of microemulsion based sparfloxacin in-situ gel for ocular delivery: In vitro and ex vivo characterization. Journal of Drug Delivery Science and Technology, 2019;1-34.
- [78]Qamar Z, Qizilbash FF, et al. Nano-Based Drug Delivery System: Recent Strategies for the Treatment of Ocular Disease and Future Perspective. Recent Pat Drug Deliv Formula. 2019;13(4):246–254.
- [79]Maulvi FA, Shetty KH, et al. Recent advances in ophthalmic preparations: Ocular barriers, dosage forms and routes of administration. Int J Pharm. 2021;608.
- [80]Di Prima G, Licciardi M, et al. Microfibrillar polymeric ocular inserts for triamcinolone acetonide delivery. Int J Pharm. 2019;567.
- [81]Souto EB, Dias-Ferreira J, et al. Advanced formulation approaches for ocular drug delivery: State-of-the-art and recent patents. Pharmaceutics. 2019;11(9): 32-48.
- [82]Shen YC, Liang CY, et al. Pharmacokinetics and safety of intravitreal caspofungin. Antimicrob Agents Chemother. 2014;58(12):7234–7239.
- [83]Benedini L, Messina P. Proniosomes and niosomes for enhanced drug delivery. Systems of Nanovesicular Drug Delivery. 2022;115–128
- [84]Akhilesh D, Faishal G, et al. Comparative Study of Carriers Used in Proniosomes. International journal of pharmaceutical and chemical sciences,2017; 456-471. www.ijpcsonline.com.
- [85]Jadhav KR, Pawar AY, et al. Proniosome: A Novel Non-ionic Provesicules as Potential Drug Carrier. Asian Journal of Pharmaceutics. 2016; 10(3): S210-S222.
- [86]Boddu M, Choppari V, et al. Formulation and Evaluation of Proniosomes of Felodipine. Drug Des. 2017;06(03).
- [87]Biju S, Talegaonkar S, et al. Vesicular systems: An overview. Indian J Pharm Sci. 2006;68(2):141–153.
- [88]A. Abd-Elbary HME. Sucrose stearate-based proniosome-derived niosomes for the nebulisable delivery of cromolyn sodium. International Journal of Pharmaceutics,2008; 357(1-2), 189–198 .
- [89]Kumar GP, Rajeshwarrao P. Nonionic surfactant vesicular systems for effective drug delivery—an overview. Acta Pharm Sin B. 2011;1(4):208–219.
- [90]Mittal S, Chaudhary A, et al. Proniosomes: The effective and efficient drug-carrier system. Therapeutic Delivery. 2020;11(2):125–137.
- [91]Fouda NH, Abdelrehim RT, et al. Sustained ocular delivery of dorzolamide-HCL via proniosomal gel formulation: In-vitro characterization, statistical optimization, and in-vivo pharmacodynamic evaluation in rabbits. Drug Deliv. 2018;25(1):1340–1349.
- [92]Abdurrahman M. Fahmy DAE-SABIBAHSAT& NAB. Penetration enhancer-containing spanlastics (PECSs) for transdermal delivery of haloperidol: in vitro characterization, ex vivo permeation and in vivo biodistribution studies. Drug Delivery, 2017;25(1), 12–22.
- [93]Khalil RM, Abdelbary GA, et al. Design and evaluation of proniosomes as a carrier for ocular delivery of lomefloxacin HCl. J Liposome Res. 2017;27(2):118–129.
- [94]Vicario-de-la-Torre M, Benítez-del-Castillo JM, et al. Design and characterization of an ocular topical liposomal preparation to replenish the lipids of the tear film. Invest Ophthalmol Vis Sci. 2014;55(12):7839–7847.
- [95]Hotujac Grgurević M, Juretić M, et al. Tear fluid-eye drops compatibility assessment using surface tension. Drug Dev Ind Pharm. 2017;43(2):275–282.
- [96]Singh G, Dwivedi H, et al. Niosomal Delivery of Isoniazid - Development and Characterization. Tropical Journal of Pharmaceutical Research. 2011;10(2):203–210.
- [97]William BS, L AJ, et al. A Review on Niosomes in Ocular Drug Delivery System. International Journal of Research and Review (ijrrjournal.com). 2020;7(10):10.
- [98]El-Emam GA, Girgis GNS, et al. Ocular inserts of voriconazole-loaded proniosomal gels: Formulation, evaluation and microbiological studies. Int J Nanomedicine. 2020;15:7825–7840.
- [99]Abdelbary GA, Amin MM, et al. Ocular ketoconazole-loaded proniosomal gels: Formulation, ex vivo corneal permeation and in vivo studies. Drug Deliv. 2017;24(1):309–319.
- [100]Lokapur JS. Formulation and Evaluation of Timolol Maleate Proniosomal Gel for Ocular Drug Delivery. International Journal of Pharmaceutical Investigation. 2022;386–390.
- [101]Singh Shambhavi SP. View of design and development of proniosomal gel of acetazolamide for the treatment of glaucoma. Journal of Pharmaceutical Negative Results. 2022;2341–2353.
- [102]Viswanath V, Tulasi P. Formulation, optimization and characterization of Betaxolol hydrochloride proniosomes using 3-2 factorial design. International Journal of Research in Pharmaceutical Sciences and Technology. 2020;1(3):89–97.
- [103]Yadav kiran, Yadav deepak, et al. Special Issue of The Patent Office Journal continued from part-8. 2016.
- [104]Munish garg MJ. Novel Proniosomal Gel of Withania Somnifera - Patent. 2012. https://www.quickcompany.in/patents/novel-proniosomal-gel-of-withania-somnifera. Accessed 21 February 2023.
- [105]Munish garg, parul garg. Novel Ursolic Acid Loaded Proniosomal Gel and Method Of Preparation. 2012. https://www.quickcompany.in/patents/novel-ursolic-acid-loaded-proniosomal-gel-and-method-of-preparation-thereof. Accessed 21 February 2023.
- [106]wipo. Targeted vesicular constructs for cytoprotection and treatment of h. pylori infections - Google Patents. 2000. https://patents.google.com/patent/WO2000042987A8/en. Accessed 21 February 2023.
- [107]Qiang L XJLLHHSLBZ. Formulation of paeonol proniosomes and preparing method thereof. Patent CN103340823A. 2013.
- [108]Hartmann A, Schmitt -Kopplin P. Methods and compositions for treating inflammatory diseases. 2017; 812-827.
- [109]Govindarajan S, Swamivelmanickam M, et al. A Comprehensive Study on Provesicular Drug Delivery System: Proniosomal Gel. Indian J Pharm Sci. 2022;84(1):1–13.