Antibacterial activity of silver nanoparticles synthesized from leaf and flower extracts of Galinsoga formosa
Abstract
Silver Nanoparticles (Ag-NPs) are progressively exercised as an antimicrobial agent among myriad applications. The rapid emergence of microbial resistance to conventional antibiotics by multidrug-resistant pathogens has become a threat to the global health community. Traditionally used herbal plants are good sources of bioactive phytochemicals and Galinsoga formosa is one of them. Thus, Ag-NPs are biologically synthesized, intending to evaluate the antibacterial activity of G. formosa. Disc diffusion assay was used to assess the antibacterial activity of leaf and flower crude extracts distinctly as well as of green synthesized Ag-NPs in vitro. The biosynthesis of Ag-NPs was primarily confirmed by brownish color solution and later by UV-visible spectrophotometer. Ag-NPs synthesized from G. formosa leaf, and flower extract showed antibacterial activity against gram-positive (S. aureus, S. mutans, and S. epidermidis) and gram-negative (K. pneumoniae and P. aeruginosa) bacteria, where gram-negative bacteria were more sensitive than gram-positive bacteria. The highest zone of inhibition was observed against P. aeruginosa (13.33±0.58 mm) by applying Ag-NPs synthesized from G. formosa flower extract. In contrast, the lowest zone of inhibition was observed against S. epidermidis (6.33±0.58 mm). Antibacterial activity of Ag-NPs from flower and leaf extracts was considerably higher as compared to their respective crude extract. Further, Ag-NPs from the flower extract was exhibited more growth inhibitory response than the leaf extract. Hence, the findings of this research suggested that the synthesized Ag-NPs from G. formosa leaf and flower extract were exhibited antibacterial activity. Such synthesized Ag-NPs might help to develop new drug for combating against various diseases.
INTRODUCTION
The gradual resistance of antimicrobial drugs, especially antibacterial agents, has become a serious issue and a global threat to the public health community[1,2]. In the US, over 2.8 million antibiotic-resistant infections occur annually, and quite 35,000 people are died according to the antibiotic resistance threats reports 2019 [3]. Antimicrobial resistance is one of the highest ten global public health challenges declared by the World Health Organization (WHO), and in Bangladesh, up to 80% of deaths occur in intensive care units (ICUs) [4]. However, medicinal plants are commonly used in rural areas as a remedy for various diseases and such plants can find a solution for these challenging bacterial infections because they are rich source of biologically active compounds like antimicrobials [5]. Plants have been using as prophylaxis for a long time since they possess secondary metabolites with several biological activities, such as antimicrobial, antioxidant, antifungal, and anti-inflammatory [6,7]. The disposition to use plants has augmented due to having trepidation from side effects of synthetic chemical drugs as well as the resistance of drugs to a specific treatment [8,9]. Galinsoga, a genus of flowering herbs in the family of Asteraceae, has been being used especially flower as folk medicine owing to different medicinal activities [10]. In specific, G. formosa is commonly used to alleviate gum pain, mouth infections, and serves as an anti-inflammatory, antimicrobial, or wound healing agent [11].
Nanotechnology to medicine (nanomedicine) has profound application in the pharmaceutical and biotechnology industries [12–15]. In the advent of bacterial multidrug resistance, silver nanoparticles provided a radically new solution to the problem as well as in other fields [16]. The biological synthesis of silver nanoparticles can enhance the antimicrobial activity of plant bioactive compounds in the crude extract as well as the activity of conventional inactive antibiotics [17–19]. In modern nanoscience and nanotechnology, silver nanoparticles play a key role in medicine, and because of their distinctive features like the crossing of brain-blood barrier, it has concentrated on their potential uses in the detection and treatment of cancer [20,21]. Ag-NPs can be synthesized in several ways, where conventional physical and chemical techniques appear to be very costly and challenging. Still, biological approaches are likely to be simple, fast, non-toxic, effective, and environmentally sustainable [22–25]. Because of its broad-spectrum efficacy and low cytotoxicity, it has been shown to be effective as an antiseptic and antimicrobial against both gram-positive and gram-negative bacteria. [26,27]. Therefore, this research aimed to investigate the medicinal value of G. formosa leaf and flower extracts in terms of antibacterial activity by the synthesis of Ag-NPs against five pathogenic bacteria.
MATERIALS AND METHODS
Sample collection
Fresh G. formosa leaves and flowers were collected during the late summer (June 2019 ) from the Mawlana Bhashani Science and Technology University campus at Tangail, Bangladesh (24°14’09.3″N 89°53’26.2″E).
Extract preparation of G. formosa leaf and flower
Freshly collected G. formosa leaf and flower were washed with tap water and then rinsed with distilled and deionized water (Total water purification, Dhaka, Bangladesh.) correspondingly to remove dust and other unwanted particles from the surface area. The leaf and flower of the G. formosa were ground separately with mortar and pestle. The pulverized leaf and flower were mixed with deionized water and filtered with a cotton cloth and Whatman No.1 filter paper respectively. Then it was centrifuged at 6000 rpm for 10 minutes. Finally, the extracts were stored at 4 °C for further use.
Synthesis of Ag-NPs from G. formosa
Different concentrations (10 mM and 5 mM) of AgNO3 (Merck KGaA, Germany) solutions were mixed with G. formosa leaf and flower extract at a ratio of 2:3 to a final volume of 10 mL. The extracts were mixed properly and kept at room temperature for 24h in a dark place to avoid photoactivation of AgNO3 until the brownish color was formed [28,29]. For the separation of silver nanoparticles, the mixtures were subjected to centrifugation at 9000 rpm for 15 minutes. Removal of excessive silver ions was completed through the rinsing of precipitates of Ag-NPs. Finally, Ag-NPs solutions were obtained by re-dispersed in deionized water, and the sample was then stored at 4°C for further analysis.
UV- visible spectroscopy analysis
The reduction of silver ions in silver nanoparticles was monitored by a UV-visible spectrophotometer (T60 UV-visible Spectrophotometer, PG Instruments, UK) at the wavelength range of 280 – 600 nm. The absorbance of silver nanoparticle aliquots was measured after they were diluted with deionized water.
Disc diffusion method for antibacterial activity
The antimicrobial activities of synthesized Ag-NPs were tested using disc diffusion bioassay described by Bakht et al. (2011) with slight modification [30]. Six hours old bacterial cultures on MHA (Mueller Hinton Agar; Becton, Dickinson, and Company) plates were uniformly spread with the glass spreader. Three discs of 5 mm diameter were placed on each MHA plate which contained 15 µl synthesized Ag-NPs from plant extract as sample, deionized water without sample as negative control, and ampicillin and azithromycin (Himedia, India) as positive control. The test samples were incubated at 37°C for 18 hours, and then the zone of inhibition was measured. Diameters of the inhibition zone formed were measured in millimeters using a measuring scale. To better understand the entire study, a pictorial representation of the procedure is outlined in Figure 1.
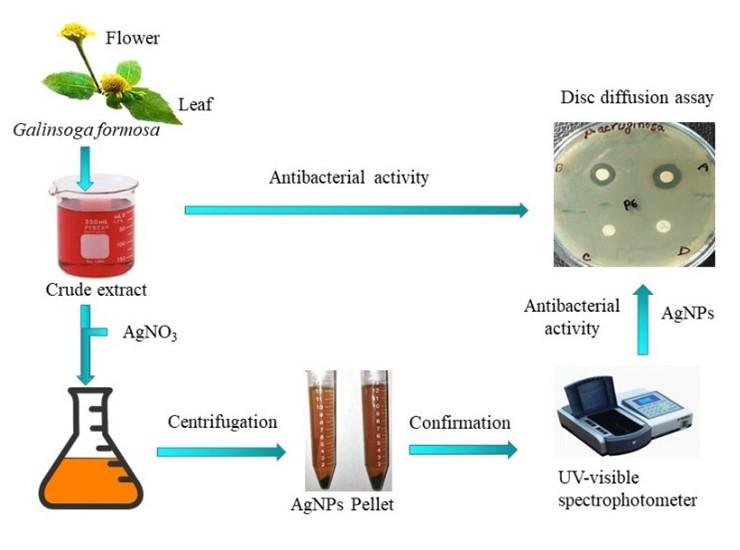
Statistical analysis
One way analysis of variance (ANOVA) and independent-sample t-test were performed by Statistical Packages for Social Sciences (SPSS Statistics for Windows, Version 20. Armonk, NY: IBM Corp) software, where p<0.05 was considered as significant and Microsoft Excel 2013 were used for graphical evaluations. All experiments were repeated three times and results were displayed as Mean ± SD.
RESULTS
Confirmation of the synthesized Ag-NPs by observing visible color change and UV-visible spectral analysis
The G. formosa leaf and flower water extracts were used in the synthesis of Ag-NPs. The bio-reductive formation of Ag-NPs was primarily confirmed by visual observation. The addition of aqueous AgNO3 solution with G. formosa leaf and flower extract resulted in the formation of a brownish color solution after overnight incubation at room temperature in the dark, which indicated the biosynthesis of Ag-NPs. The control AgNO3 solution without plant extract was not shown any color change under similar conditions. Besides, the formation of Ag-NPs was confirmed by a UV-visible spectrophotometer (Figure 2). The Ag-NPs formed by leaf extract showed a peak near 350 nm to 400 nm wavelength, and a sharp peak was observed around 300 nm for the G, formosa flower extract.
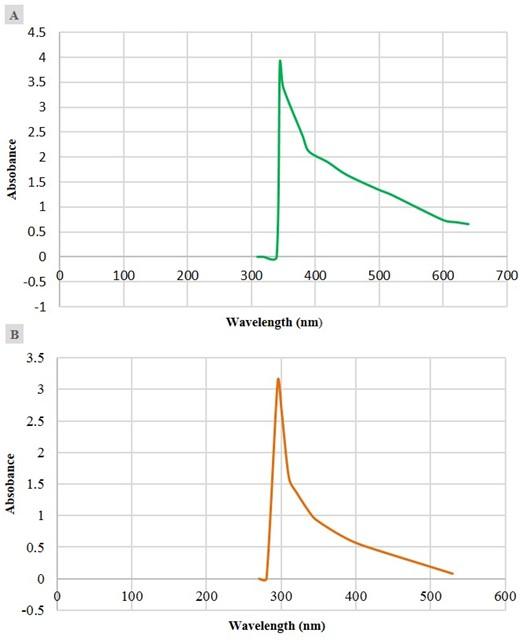
Antibacterial activity of crude extract of G. formosa
The crude extract of G. formosa leaves and flowers was applied on 5 distinct bacterial strains to detect their antibacterial activity. Before using, the plant crude extracts were filtered through a millipore filter to remove existing bacteria. Among the five pathogenic strains, three were gram-positive (S. aureus; GCA_000013425.1, S. epidermidis; GCA_006094375.1, and S. mutans; GCA_001558215.1) and two were gram-negative (K. pneumoniae; GCA_000240185.2 and P. aeruginosa; GCA_000006765.1). However, both of these crude extracts hardly showed considerable antibacterial activity against the bacterial strains.
Higher antibacterial activity of synthesized Ag-NPs than crude extracts
The antibacterial activity of the synthesized Ag-NPs from G. formosa was increased despite the limited activity of crude extract. In this experiment, it was observed that silver nanoparticles showed better performance than crude extracts.
Flower extract showed higher antibacterial activity than leaf extract
The Ag-NPs synthesized from G. formosa flower extract showed more growth inhibitory response against the tested bacteria. The maximum 13.33±0.58 mm zone of inhibition was recorded for flower extract against P. aeruginosa, whereas Ag-NPs from G. formosa leaf extract showed an 11.33±0.58 mm zone of inhibition. The synthesized Ag-NPs were more sensitive against the aforementioned two bacterial strains, where it is clear that Ag-NPs from flower extract were more potent than leaf extract. The detailed result is depicted in Figure 3.
Antimicrobial activity of Ag-NPs was not affected by silver nitrate solutions
Two different concentrations of silver nitrate solutions (10 mM (A) and 5 mM (B)) were used to synthesize Ag-NPs from G. formosa plant extract. No remarkable differences were observed in the two different concentrations in terms of their activity (Figure 3). In most of the cases, they pointed very similar results. So, the concentration of silver nitrate solution made no major changes in antibacterial activity by synthesizing particular Ag-NPs.
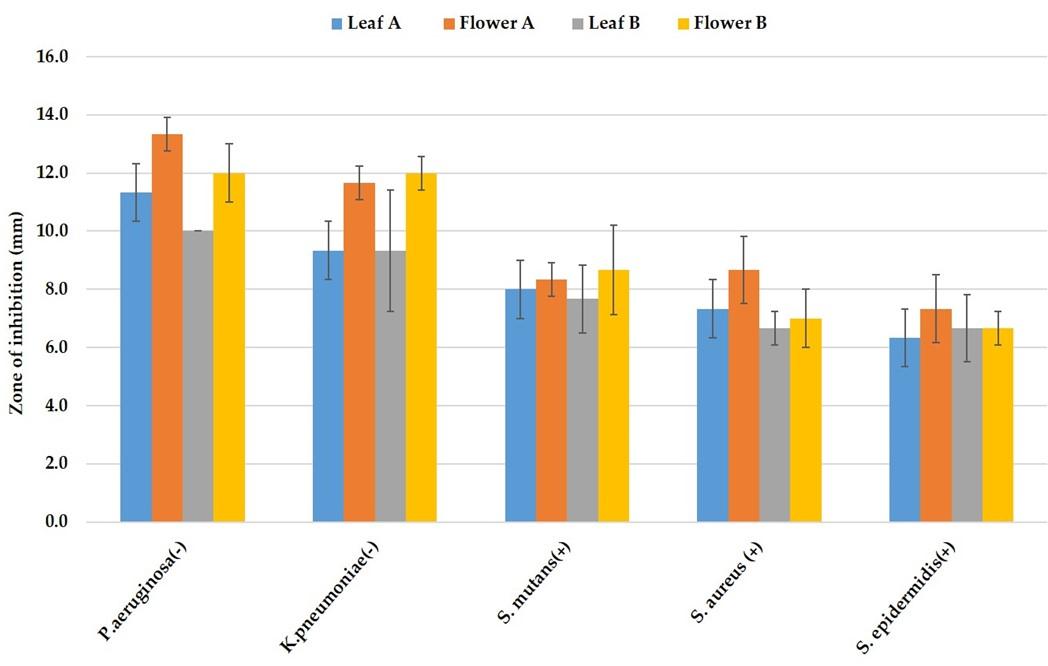
Gram-negative bacteria are more sensitive than gram-positive bacteria to synthesized nanoparticles
The synthesized Ag-NPs were investigated for their antibacterial activity assay against both gram-positive (S. aureus, S. epidermidis, and S. mutans) and gram-negative (K. pneumoniae and P. aeruginosa) bacteria by disc diffusion method. In this study, a maximal 13.33±0.58 mm zone of inhibition was recorded for G. formosa flower extract and 11.33±0.58 mm for G. formosa leaf extract against P. aeruginosa (Figure 4a; P-5 and Figure 4b: P-6; Table 1). On the contrary, the lowest zone of inhibition was observed against S. epidermidis both for G. formosa leaf and flower extract (Figure 4a; P-4 and Figure 4b: P-9; Table 1). Among the five tested pathogenic bacteria, the synthesized Ag-NPs from G. formosa was more effective towards gram-negative bacteria as compared to gram-positive bacteria (Figure 4a and 4b; Table 1). The comparison of such differences between gram-negative and gram-positive bacteria was disclosed in Figure 3.
The synthesized nanoparticles from G. formosa leaf and flower extract exhibited minimal growth inhibitory effects against S. epidermidis (Figure 4a: P-4 and Figure 4b: P-9) and S. aureus (Figure 4a: P-1 and Figure 4b: P-7) as well as S. mutans (Figure 4a: P-3 and Figure 4b: P-8) but showed maximal growth inhibitory effects against K. pneumonia (Figure 4a; P-2 and Figure 4b: P-10) and P. aeruginosa (Figure 4a: P-5 and Figure 4b: P-6). Surprisingly, no zone of inhibition was found for ampicillin (25 µg/mL) against those strains, but azithromycin (30 µg/mL) showed a broad-spectrum antibacterial activity (Figure 4a and 4b).
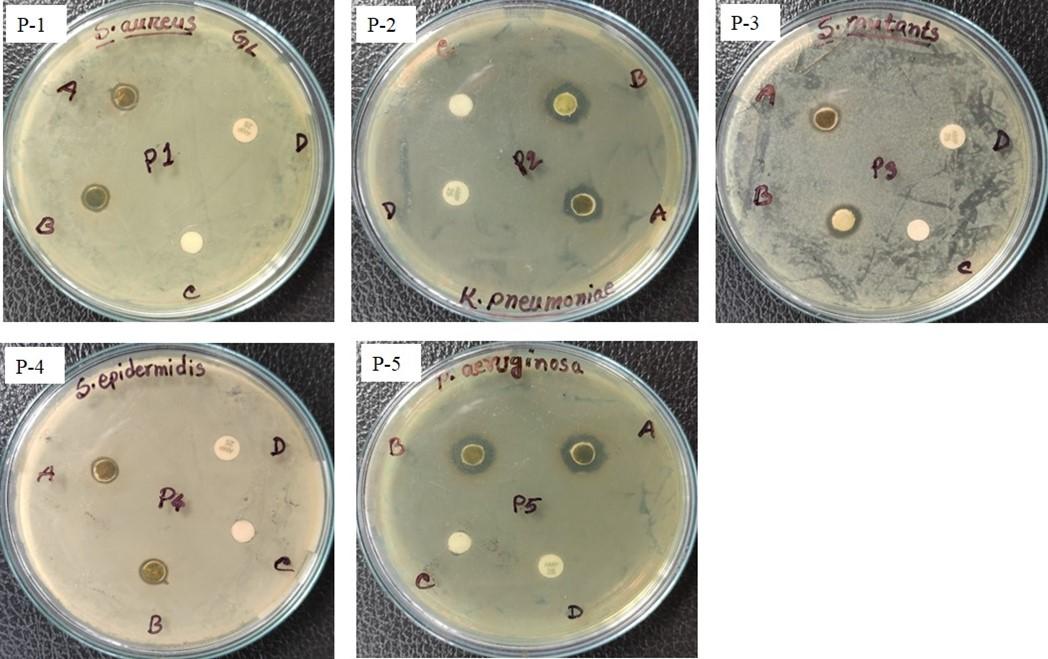
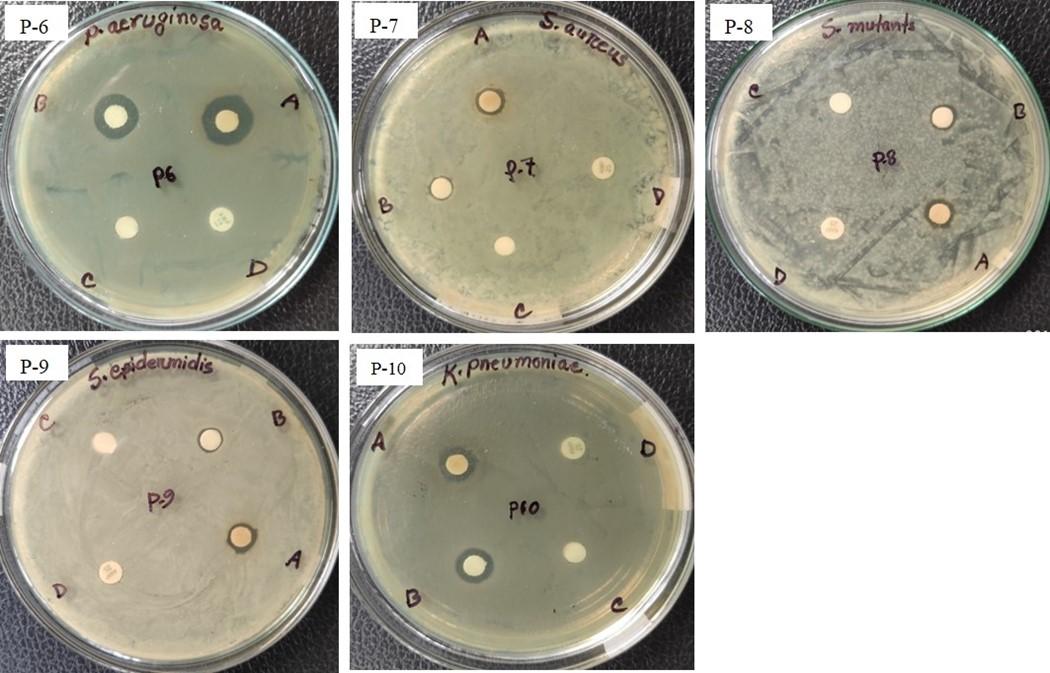
Table 1. Antibacterial activity of synthesized Ag-NPs.
DISCUSSION
Bacterial resistance is a burning issue in the medical sector now-a-days, where Ag-NPs might be a solution due to their bactericidal properties. The scientists focus on a variation in the synthesis of nanoparticles for the development of antibiotics against microorganisms as Ag-NPs can improve the bioactivity of natural compounds, but the activity depends on the size of the nanoparticles [31,32]. The biologically synthesized Ag-NPs are eco-friendly, safe, and easy to use [33]. To synthesize Ag-NPs, G. formosa leaf and flower crude extract were treating with AgNO3 solution and initially confirmed by a visual color change to brown [34,35]. This is the primary indication for the formation of Ag-NPs, which is further confirmed by a UV-visible spectrophotometer. After confirmation, the synthesized nanoparticles from G. formosa leaf and flower extract were applied on five bacterial strains and exhibited growth-inhibitory response against all the strains, but showed prominent growth inhibitory effects against two strains namely, K. pneumonia and P. aeruginosa. According to the results, it was observed that the gram-negative bacteria showed more sensitivity to synthesized Ag-NPs than gram-positive bacteria, where flower extract was more potent than leaf extract. Alfuraydi et al. (2019) reported that synthesized Ag-NPs using sesame oil cake inhibited the growth of gram-negative bacteria such as P. aeruginosa, K. pneumonia, and E. coli, while Bacillus subtilis and S. aureus were not sensitive [36]. The outcomes of this study are also in agreement with the previous findings reported on the Datura stramunium leaf extract-assisted Ag-NPs and assessment of their antibacterial activity [37]. Senthil et al. (2017) observed that Ag-NPs enhanced protein leakage from bacterial cells, where they were more susceptible to gram-negative bacteria than gram-positive bacteria [38]. Such findings indicated that the nanoparticles might inhibit the growth of gram-negative bacteria more specifically than gram-positive bacteria.
However, the exact mechanism remains unclear; the adherence of Ag-NPs to bacterial cells, cell disruption, generation of reactive oxygen species, and free radical production, as well as the regulation of bacterial signal transduction pathways, have been recognized as the most important modes of antimicrobial action [39,40]. The discriminate bactericidal performance of Ag-NPs might depend on distinct interaction patterns between the bacterial cell wall and Ag-NPs. On the contrary, the charge of Ag-NPs is correlated with bacterial cell damage as gram-negative bacteria are more susceptible to Ag+ ions invasion than gram-positive bacteria [41,42]. Due to the difference in peptidoglycan structure, and denser outer membrane, some researchers described that Ag-NPs are more effective in gram-negative bacteria [34,42,43]. The gram-positive bacteria are resistant to the action of the nanoparticles because of their variations in the cell wall structures, and the activity of the nanoparticles towards the pathogens might be interrelated with the diverse alterations in the morphological features between gram-negative and gram-positive bacteria [36]. But several studies showed that Ag-NPs are effective in both gram-positive and gram-negative bacteria [44–47]. Although the antibacterial activity of Ag-NPs depends on particle charge, size, shape, and interaction of phytochemicals, the bacterial cell wall structures might be another important factor. Limitations of this study were smaller sample size, and unavailability of advanced characterization of the synthesized Ag-NPs. While the fresh G. formosa is commonly used as an antimicrobial as well as an anti-inflammatory agent, this experiment could not find such strong behavior of fresh aqueous crude extract. It is assumed that there are active ingredients in the plant that need to be identified in further study.
CONCLUSION
The antibacterial activity of bioderived silver nanoparticles from G. formosa flower and leaf extract was assessed against five pathogenic bacteria. The findings of this research summarized that the synthesized Ag-NPs inhibited the growth of gram-negative bacteria more specifically as compared to gram-positive bacteria. The synthesized Ag-NPs from flower extract has more antibacterial activity than leaf extract. This might be due to the existence of unique natural compounds or different concentrations of the same compounds in the different parts of the plants. The plant extract might contain novel ingredients that should be identified in further research. Such bioactive natural compounds could be served as supporting materials for the formulation of new medication against various bacterial infections.
ACKNOWLEDGMENT
The authors would like to thank the Department of Biochemistry and Molecular Biology, Mawlana Bhashani Science and Technology University, Tangail-1902, Bangladesh for financial and laboratory support to carry out this research work.
AUTHORS CONTRIBUTION
MRM, AJ, and MAS planned and designed the research. MAS supervised the whole research. MRM, AJ, CB, SAS, TS, and TA conducted the entire laboratory works. MAS, MAZ, and MK arranged the entire facilities for the research. MRM and RM interpreted the results and performed the statistical analysis. MRM drafted the manuscript. MAS, MZA, and MAI have thoroughly edited and revised the manuscript. All authors read and approved the manuscript.
CONFLICTS OF INTEREST
There is no conflict of interest among the authors.
References
- [1]Manandhar S, Luitel S, Dahal RK. In Vitro Antimicrobial Activity of Some Medicinal Plants against Human Pathogenic Bacteria. J Trop Med 2019;2019.
- [2]Giamarellou H. Multidrug-resistant Gram-negative bacteria: How to treat and for how long. Int J Antimicrob Agents 2010;36.
- [3]Center for Disease Control. Biggest Threats and Data | Antibiotic/Antimicrobial Resistance | CDC. 2019 2019:1.
- [4]Superbugs linked to 80% of deaths in Bangladeshi ICUs | Dhaka Tribune n.d. https://www.dhakatribune.com/health/2019/04/24/superbugs-linked-to-80-of-deaths-in-bangladeshi-icus (accessed February 28, 2021).
- [5]Cushnie TPT, Cushnie B, Lamb AJ. Alkaloids: An overview of their antibacterial, antibiotic-enhancing and antivirulence activities. Int J Antimicrob Agents 2014;44:377–386.
- [6]Sakkas H, Papadopoulou C. Antimicrobial activity of basil, oregano, and thyme essential oils. J Microbiol Biotechnol 2017;27:429–438.
- [7]Koyuncu I, Gönel A, Akdağ A, Yilmaz MA. Identification of phenolic compounds, antioxidant activity and anti-cancer effects of the extract obtained from the shoots of Ornithogalum narbonense L. Cell Mol Biol 2018;64:75–83.
- [8]Mohan L. Plant-Based Drugs as an Adjuvant to Cancer Chemotherapy. Altern. Med. 2020.
- [9]Gorlenko CL, Kiselev HY, Budanova E V., Zamyatnin AA, Ikryannikova LN. Plant secondary metabolites in the battle of drugs and drug-resistant bacteria: New heroes or worse clones of antibiotics? Antibiotics 2020;9.
- [10]Bazylko A, Boruc K, Borzym J, Kiss AK. Aqueous and ethanolic extracts of Galinsoga parviflora and Galinsoga ciliata. Investigations of caffeic acid derivatives and flavonoids by HPTLC and HPLC-DAD-MS methods. Phytochem Lett 2015;11:394–398.
- [11]Visht S, Rathi V, Saini A, Anjum N. Antibacterial activity of volatile oil from areal plant Galinsoga formosa. Int J Curr Adv Res 2018;7:11446–11448.
- [12]Farokhzad OC, Langer R. Impact of nanotechnology on drug delivery. ACS Nano 2009;3:16–20.
- [13]Shi J, Xiao Z, Kamaly N, Farokhzad OC. Self-assembled targeted nanoparticles: Evolution of technologies and bench to bedside translation. Acc Chem Res 2011;44:1123–1134.
- [14]Shi J, Votruba AR, Farokhzad OC, Langer R. Nanotechnology in drug delivery and tissue engineering: From discovery to applications. Nano Lett 2010;10:3223–3230.
- [15]Wagner V, Dullaart A, Bock AK, Zweck A. The emerging nanomedicine landscape. Nat Biotechnol 2006;24:1211–7.
- [16]Rai MK, Deshmukh SD, Ingle AP, Gade AK. Silver nanoparticles: The powerful nanoweapon against multidrug-resistant bacteria. J Appl Microbiol 2012;112:841–852.
- [17]Panáček A, Smékalová M, Večeřová R, Bogdanová K, Röderová M, Kolář M, et al. Silver nanoparticles strongly enhance and restore bactericidal activity of inactive antibiotics against multiresistant Enterobacteriaceae. Colloids Surfaces B Biointerfaces 2016;142:392–399.
- [18]Garibo D, Borbón-Nuñez HA, de León JND, García Mendoza E, Estrada I, Toledano-Magaña Y, et al. Green synthesis of silver nanoparticles using Lysiloma acapulcensis exhibit high-antimicrobial activity. Sci Rep 2020;10:12805.
- [19]Islam A, Mandal C, Habib A. Antibacterial potential of synthesized silver nanoparticles from leaf extract of Moringa oleifera. J Adv Biotechnol Exp Ther 2021;4:67.
- [20]Zhang XF, Liu ZG, Shen W, Gurunathan S. Silver nanoparticles: Synthesis, characterization, properties, applications, and therapeutic approaches. Int J Mol Sci 2016;17.
- [21]Liao C, Li Y, Tjong SC. Bactericidal and cytotoxic properties of silver nanoparticles. Int J Mol Sci 2019;20.
- [22]Gurunathan S, Park JH, Han JW, Kim JH. Comparative assessment of the apoptotic potential of silver nanoparticles synthesized by Bacillus tequilensis and Calocybe indica in MDA-MB-231 human breast cancer cells: Targeting p53 for anticancer therapy. Int J Nanomedicine 2015;10:4203–23.
- [23]Gurunathan S, Kalishwaralal K, Vaidyanathan R, Venkataraman D, Pandian SRK, Muniyandi J, et al. Biosynthesis, purification and characterization of silver nanoparticles using Escherichia coli. Colloids Surfaces B Biointerfaces 2009;74:328–335.
- [24]Hamelian M, Zangeneh MM, Amisama A, Varmira K, Veisi H. Green synthesis of silver nanoparticles using Thymus kotschyanus extract and evaluation of their antioxidant, antibacterial and cytotoxic effects. Appl Organomet Chem 2018;32.
- [25]Khan M, Khan M, Adil SF, Tahir MN, Tremel W, Alkhathlan HZ, et al. Green synthesis of silver nanoparticles mediated by Pulicaria glutinosa extract. Int J Nanomedicine 2013;8:1507–1516.
- [26]Franci G, Falanga A, Galdiero S, Palomba L, Rai M, Morelli G, et al. Silver nanoparticles as potential antibacterial agents. Molecules 2015;20:8856–8874.
- [27]Bindhu MR, Umadevi M. Antibacterial and catalytic activities of green synthesized silver nanoparticles. Spectrochim Acta – Part A Mol Biomol Spectrosc 2015;135:373–378.
- [28]Abdel-Aziz MS, Shaheen MS, El-Nekeety AA, Abdel-Wahhab MA. Antioxidant and antibacterial activity of silver nanoparticles biosynthesized using Chenopodium murale leaf extract. J Saudi Chem Soc 2014;18:356–363.
- [29]Sarkar S, Kotteeswaran V. Green synthesis of silver nanoparticles from aqueous leaf extract of Pomegranate (Punica granatum) and their anticancer activity on human cervical cancer cells. Adv Nat Sci Nanosci Nanotechnol 2018;9.
- [30]Bakht J, Islam A, Ali H, Tayyab M , Shafi M. Antimicrobial potentials of Eclipta alba by disc diffusion method. African J Biotechnol 2011;10:7658–7667.
- [31]Morones JR, Elechiguerra JL, Camacho A, Holt K, Kouri JB, Ramírez JT, et al. The bactericidal effect of silver nanoparticles. Nanotechnology 2005;16:2346–2353.
- [32]Espinosa-Cristóbal LF, Martínez-Castañón GA, Martínez-Martínez RE, Loyola-Rodríguez JP, N. Patiño-Marín, Reyes-Macías JF, et al. Antibacterial effect of silver nanoparticles against Streptococcus mutans. Mater Lett 2009;63:2603–2606.
- [33]Patil MP, Singh RD, Koli PB, Patil KT, Jagdale BS, Tipare AR, et al. Antibacterial potential of silver nanoparticles synthesized using Madhuca longifolia flower extract as a green resource. Microb Pathog 2018;121:184–189.
- [34]Asaduzzaman AKM, Chun B-S, Kabir SR. Vitis vinifera Assisted Silver Nanoparticles with Antibacterial and Antiproliferative Activity against Ehrlich Ascites Carcinoma Cells . J Nanoparticles 2016;2016:1–9.
- [35]Singh H, Du J, Yi TH. Green and rapid synthesis of silver nanoparticles using Borago officinalis leaf extract: anticancer and antibacterial activities. Artif Cells, Nanomedicine Biotechnol 2017;45:1310–1316.
- [36]Alfuraydi AA, Devanesan S, Al-Ansari M, AlSalhi MS, Ranjitsingh AJ. Eco-friendly green synthesis of silver nanoparticles from the sesame oil cake and its potential anticancer and antimicrobial activities. J Photochem Photobiol B Biol 2019;192:83–89.
- [37]Gomathi M, Rajkumar PV, Prakasam A, Ravichandran K. Green synthesis of silver nanoparticles using Datura stramonium leaf extract and assessment of their antibacterial activity. Resour Technol 2017;3:280–284.
- [38]Senthil B, Devasena T, Prakash B, Rajasekar A. Non-cytotoxic effect of green synthesized silver nanoparticles and its antibacterial activity. J Photochem Photobiol B Biol 2017;177:1–7.
- [39]Dakal TC, Kumar A, Majumdar RS, Yadav V. Mechanistic basis of antimicrobial actions of silver nanoparticles. Front Microbiol 2016;7.
- [40]de Lacerda Coriolano D, de Souza JB, Bueno EV, Medeiros SM de FR dos S, Cavalcanti IDL, Cavalcanti IMF. Antibacterial and antibiofilm potential of silver nanoparticles against antibiotic-sensitive and multidrug-resistant Pseudomonas aeruginosa strains. Brazilian J Microbiol 2020, 52(1):267-278.
- [41]Malapermal V, Botha I, Krishna SBN, Mbatha JN. Enhancing antidiabetic and antimicrobial performance of Ocimum basilicum, and Ocimum sanctum (L.) using silver nanoparticles. Saudi J Biol Sci 2017, 24(6):1294-1305.
- [42]Liao C, Li Y, Tjong SC. Bactericidal and cytotoxic properties of silver nanoparticles. Int J Mol Sci 2019;20.
- [43]Singh G, Babele PK, Shahi SK, Sinha RP, Tyagi MB, Kumar A. Green synthesis of silver nanoparticles using cell extracts of Anabaena doliolum and screening of its antibacterial and antitumor activity. J Microbiol Biotechnol 2014;24:1354–1367.
- [44]Ameen F, Srinivasan P, Selvankumar T, Kamala-Kannan S, Al Nadhari S, Almansob A, et al. Phytosynthesis of silver nanoparticles using Mangifera indica flower extract as bioreductant and their broad-spectrum antibacterial activity. Bioorg Chem 2019;88.
- [45]Tang S, Zheng J. Antibacterial Activity of Silver Nanoparticles: Structural Effects. Adv Healthc Mater 2018;7.
- [46]Shehzad A, Qureshi M, Jabeen S, Ahmad R, Alabdalall AH, Aljafary MA, et al. Synthesis, characterization and antibacterial activity of silver nanoparticles using Rhazya stricta. PeerJ 2018;2018.
- [47]Brahmachari G, Sarkar S, Ghosh R, Barman S, Mandal NC, Jash SK, et al. Sunlight-induced rapid and efficient biogenic synthesis of silver nanoparticles using aqueous leaf extract of Ocimum sanctum Linn. with enhanced antibacterial activity. Org Med Chem Lett 2014, 4(1):18.