HMG-CoA reductase inhibitor, rosuvastatin averted carbon tetrachloride-induced oxidative stress, inflammation and fibrosis in the liver of rats
Abstract
The aim of this study was to examine the effect of rosuvastatin in experimentally-induced hepatic inflammation and fibrosis in rats. Carbon tetra chloride (CCl4) was administered orally to induce liver damage in female Long Evans rats. Rats were treated with CCl4 alone twice a week over two weeks. Rosuvastatin (10 mg/kg) was also given daily to CCl4 treated rats concurrently by nasogastric gavage. After two weeks, various oxidative stress markers as well as liver markers enzymes were investigated in different animal groups tested in this study. Moreover, histological assessments were also done for inflammatory cell infiltration and fibrosis in the liver of all test groups. Plasma aspartate aminotransferase (AST), alanine aminotransferase (ALT) and alkaline phosphatase (ALP) activities were increased in the CCl4 group compared with the control group. Increased liver enzyme activities were significantly decreased by rosuvastatin treatment. Moreover, rosuvastatin treatment inhibited the formation of lipid peroxidation products in CCl4 administered rats. Rosuvastatin treatment also restored the decreased superoxide dismutase (SOD) activities as well as elevated the reduced glutathione concentration in CCl4 administered rats. Liver tissues from rats of control group also revealed no significant pathological changes, while CCl4 administered rats showed significant infiltration of inflammatory cells and liver fibrosis, which was further, normalized or significantly decreased by rosuvastatin treatment. This study revealed that, rosuvastatin treatment may ameliorate all necro-inflammatory and fibrotic changes in liver tissues of CCl4 induced rats and could be used as an alternative therapy for chemical or drug-induced liver fibrosis.
INTRODUCTION
Nowadays, an upsurge of persistent liver diseases, associated with metabolic syndrome, non-alcoholic fatty liver disease, steatosis, and primary biliary cirrhosis has become one of the major concerns in health care society. A statistics showed that annual deaths due to liver cancer and cirrhosis increased by 1.25 to 1.75 million from 1990 to 2010 worldwide. In most cases, advanced cirrhosis and hepatocellular carcinoma (HCC) are the main complications behind most of the liver-related mortality [1]. Chronic liver injury leading to cirrhosis is occurred due to various insults like viral hepatitis, consumption of alcohol, irrational use of drugs, metabolic disease associated with iron or copper overload, autoimmune reaction of the hepatocyte, congenital abnormalities [2] or due to the exposure of some chemical toxin e.g. ethanol, carbon tetrachloride etc. [3, 4]. Liver malfunction is primarily associated with oxidative stress. Irreversible liver cirrhosis occurs due to persistent existence of inflammation. There are mainly two types of hepatic cells, including tissue macrophages (Kupffer cells), and a perivascular mesenchymal stellate cells, whose activation occurs following any liver injury of any etiology. Hepatocyte and kupffer cell is the primary source of ROS. In this study halo-alkane like carbon tetrachloride was used for the study of hepatotoxicity in animals. Trichloromethyl (•CCl3) and peroxy trichloromethyl (•OOCCl3) species are produced from carbon tetrachloride via the influence of phase-I cytochrome P450 enzyme [5]. These excess ROSs are the main culprit for causing oxidative stress in the liver by raising the level of different oxidative stress markers (lipid peroxidation, nitric oxide, advanced oxidative protein products, myeloperoxidase).
At present, lipid-lowering drugs have gained much more attention as an effective treatment approaches as these drugs lower cholesterol synthesis, reduce different reactive oxygen species and oxidative stress [6]. Increasing investigational studies have revealed that statins have a beneficial effect on atherosclerosis by diminishing the function of NF-κB and by up-regulating the expression of peroxisome proliferator receptors (PPAR-α), both these activity of statins reduce inflammatory responses [7]. Competitive inhibition of HMG-CoA reductase (rate limiting step for cholesterol synthesis), one of the major properties of rosuvastatin results in expression of hepatic low-density lipoprotein (LDL) receptors, reduction of oxidative stress by changing in oxidized LDL and reduction of pro-atherogenic circulating LDL cholesterol [8]. Prominent characteristics like, enhanced binding of HMG-CoA reductase, relative hydrophilicity and minimal hepatic cytochrome P450 (CYP) metabolism aid rosuvastatin to become one of the most used drug worldwide compared to other statins [9]. Besides that, several studies recommended that, rosuvastatin has some pleiotropic effects including refinement of endothelial dysfunction by upregulating endothelial synthesis (eNOS), reduction of vascular smooth muscle cell and macrophage proliferation, augmentation of nitric oxide systemic availability, antioxidant effects and immunomodulatory and anti-inflammatory properties which causes to altered phosphorylation of pro-inflammatory proteins, thus reduce the release of pro-inflammatory cytokines [10]. Hepatoprotective activity of rosuvastatin was also reported in previous study. In a cholestasis-induced hepatic injury model in rats, rosuvastatin protected the liver injury by reducing lipid peroxides and nitric oxide level [11]. However, conflicting results were also reported previously, stated that rosuvastatin treatment was not effective in preventing hepatic damage in rats induced by thioacetamide [12]. Since there is less scrutiny of hepatoprotective effect of rosuvastatin, this study was conducted to appraise the effectiveness of rosuvastatin for preventing oxidative stress, hepatitis, and liver fibrosis actuated by CCl4 administered rats.
MATERIALS AND METHODS
Animals
Twenty four Long Evans female rats (180–210 g, ten to twelve weeks old) were used for this project, collected from Animal production unit of Animal House at Department of Pharmaceutical Sciences, North South University. According to the standard protocol these rats were kept with a 12 h dark/light cycles at room temperature in individual cages and provided with standard laboratory feed and water.
Rats were grouped into four groups (I, II, III, IV; six rats in each group) to study the hepatoprotective effects of rosuvastatin. Animal group I and II were administered saline (0.85%, 1 ml/kg) and olive oil (3 ml/kg) orally twice a week for two weeks. Animals of group II also received rosuvastatin (10 mg/Kg) every day. Animals of group III and IV were administered CCl4 (1:3 in olive oil) at a dose of 1 ml/kg in the same route, time interval and duration as of group I and II. In addition to CCl4 treatment, animals of group IV received rosuvastatin (10 mg/kg) every day for two weeks. Body weight, food and water intake were monitored regularly for all the animals. All experimental protocols were approved by the Ethical Committee of North South University, for animal care and experimentation.
Animal sacrifice and tissue collection
At the end of two weeks of treatment, weight of all rats were measured and sacrificed using high dose (65 mg/kg) of pentobarbitone-Na (anesthetic agent). Seven mL blood was withdrawn from abdominal aorta from each rat and preserved in citrate buffer containing tube until centrifugation to collect the plasma at 4º C. All internal organs (heart, kidney, spleen and liver) were weighed and preserved in neutral buffered formalin (pH 7.4) immediately after collection for histological analysis and refrigerated at −20°C for further biochemical analysis. Plasma of collected blood samples were obtained by centrifugation (8000 rpm) and refrigerated at -20°C for future analysis.
Evaluation of hepatotoxicity
Hepatotoxicity was assessed by estimating various liver marker enzymes such as alanine aminotransferase (ALT), aspartate aminotransferase (AST), and alkaline phosphatase (ALP) in plasma by using DCI diagnostic kits (Hungary) according to the manufacturer’s protocol.
Preparation of tissue sample -assessment of oxidative stress markers
Liver tissue, previously collected was homogenized in 10 volumes of Phosphate buffer (pH 7.4) and centrifuged at 10000 rpm for 30 min at controlled temperature (4°C). The supernatant from the centrifugation was collected and used for the assessment of protein and enzymatic studies as described below.
Estimation of lipid peroxidation
Lipid peroxidation in liver was assessed by following previously described mehtod of (author name,) [13]. Thiobarbituric acid reactive substances were developed with the reaction of MDA which was measured by UV-spectrophotometer. The absorbance of the clear supernatant was measured against reference blank at 535 nm.
Assay of nitric oxide (NO)
Determination of Nitric oxide (NO) (as nitrate) was carried out according to the method described by Tracy et al. [14]. In this study, Griess-Illosvoy reagent was modified by using naphthyl ethylene diamine dihydrochloride (0.1% w/v) instead of 1-naphthylamine (5%). A standard curve was prepared and NO level was measured from that standard curve and expressed as nmol/mL.
Advanced oxidation protein products (APOP) assay
APOP levels was assayed adopting the method of Witko-Sarsat [15] and Tiwari [16] with slight modification and AOPP concentrations were expressed as nmol·mL−1 chloramine-T equivalents.
Catalase Assay (CAT)
Catalase activities were evaluated followed by previously described method by Chance and Maehly [17]. A change in absorbance of 0.01 unit/min considered as one unit of CAT activity.
Reduced glutathione assay (GSH)
Reduced glutathione was evaluated by the method of Jollow et al. [18] and the concentration of GSH was expressed as ng/mg protein.
Histopathological determination
Immobility of the liver tissues were achieved by using neutral buffered formalin and embedded in paraffin for the microscopic evaluation. These bloked tissues were sectioned at 5 μm with a microtome. To observe the architecture of hepatic tissue and infiltration of inflammatory cell tissue sections were stained with hematoxylin/eosin. Furthermore, fibrosis of liver tissue was evaluated by sirius red staining of liver sections. Sections were then studied and photographed under a light microscope (Zeiss Axioscope) at X40 magnifications.
Statistical analysis
Statistical analysis of the results was carried out using One-way ANOVA followed by Newman-Keuls post hoc test using Graph Pad Prism Software, version 6. All values are expressed as a mean ± standard error of the mean (SEM), n=6. Statistical significance was considered p < 0.05 in all cases.
RESULTS
Effect of rosuvastatin on liver marker enzymes (AST, ALT and ALP) activities in CCl4 administered rats
The outcome of prevention of hepatic injury produced by CCl4 is shown in figure 1. CCl4 usually increases the level of various liver marker enzyme including AST, ALT and ALP. In this experiment animal group treated with CCl4 increased the level of AST, ALT and ALP compared to control group. On the other hand animal group treated with both CCl4 and rosuvastatin significantly (p<0.05) reduces the level of AST, ALT and ALP (Figure 1 A-C).
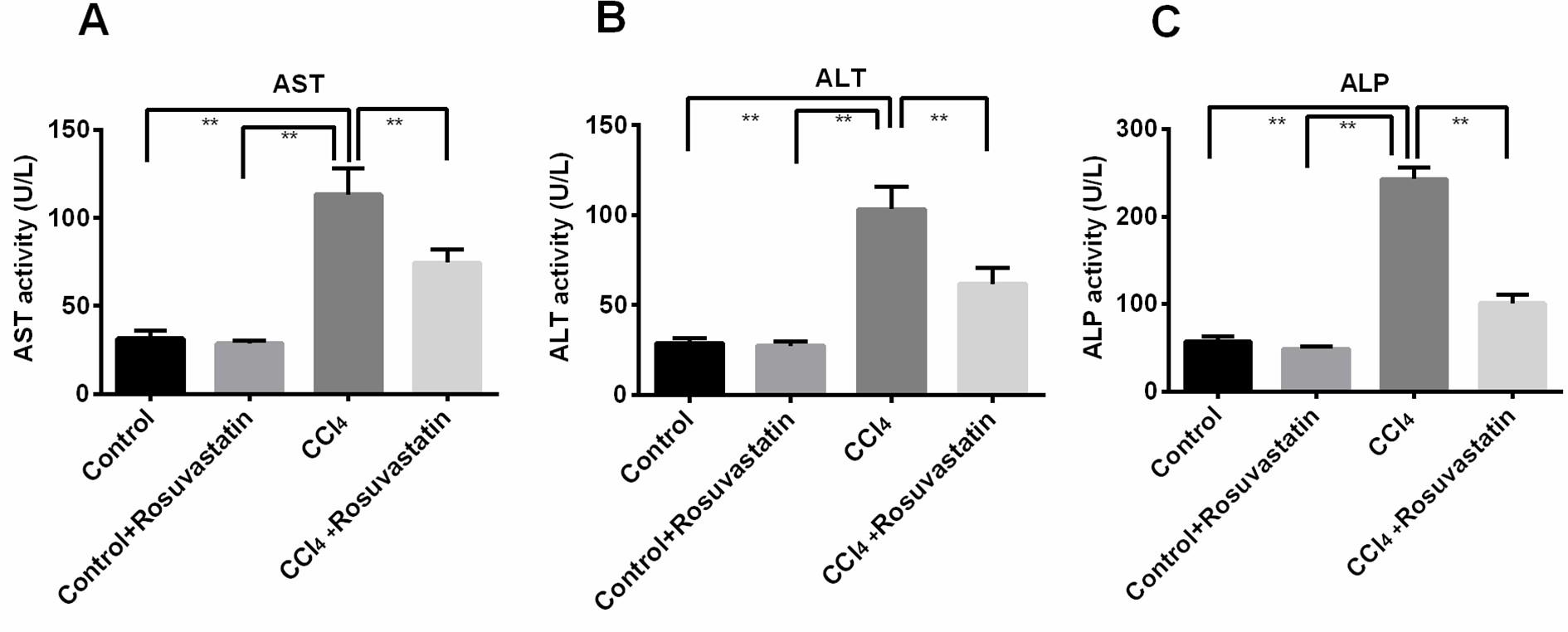
Effect of rosuvastatin on oxidative stress markers in CCl4 administered rats
Prevention of CCl4 induced oxidative stress by rosuvastatin is shown in figure 2. Typically CCl4 increases several stress marker such as MDA (Malondialdehyde), NO (Nitric oxide) and APOP (Advanced protein oxidation product) in both liver and plasma. Here, animal group treated with CCl4 showed significant (p<0.05) elevation of above mentioned stress markers in both liver and plasma compared to control group. However, treatment with rosuvastatin significantly (p<0.05) reduced the level of all the stress markers in both plasma and liver (Figure 2; A-F).
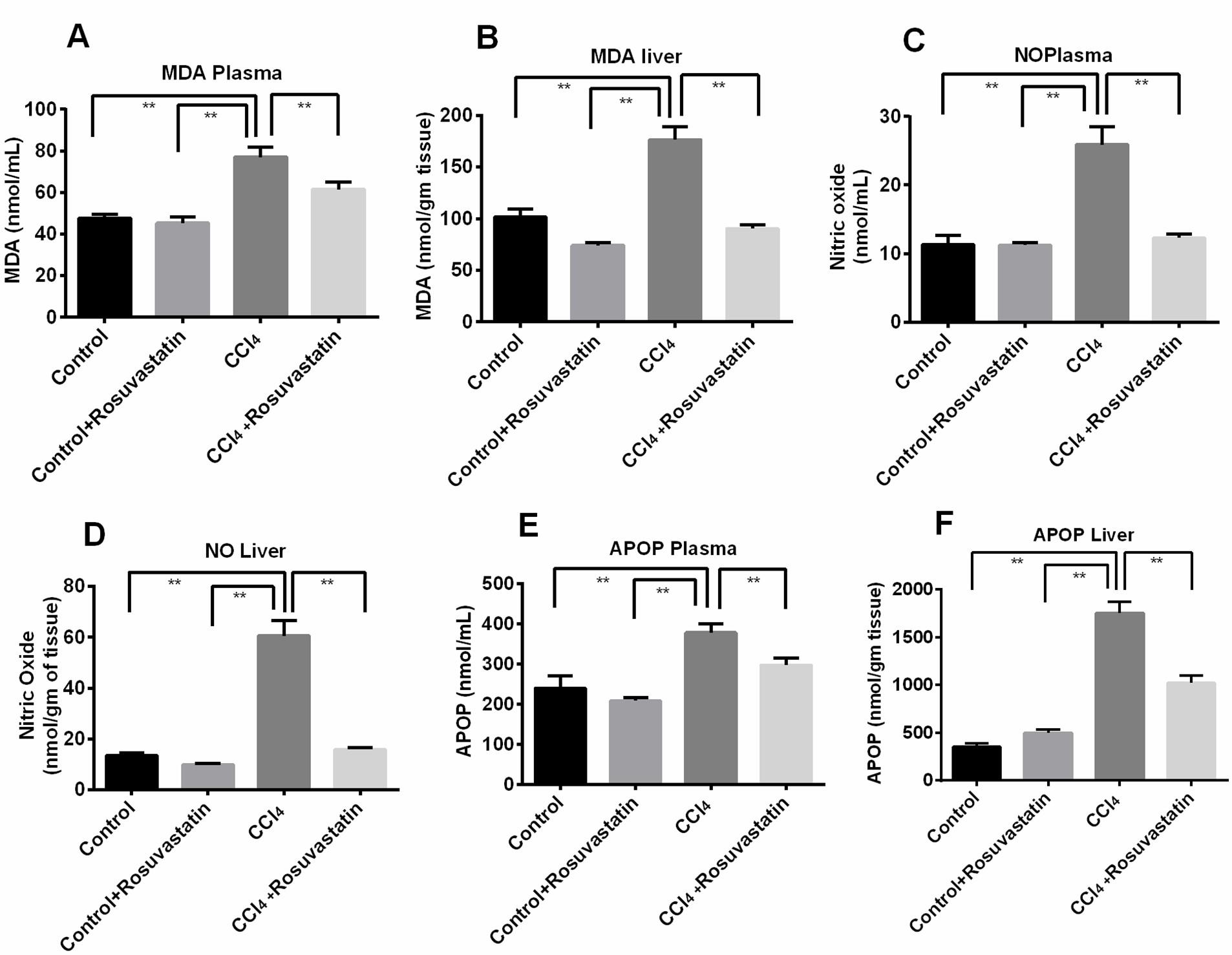
Effect of rosuvastatin on antioxidant enzyme function and inflammatory markers in CCl4 administered rats
Prevention of oxidative stress and lipid peroxidation is generally done by cellular antioxidant mechanism namely superoxide dismutase (SOD) and reduced glutathione (GSH). During oxidative stress level of both the enzymes in liver and plasma reduced. In this study CCl4 significantly (p<0.05) reduced the level of SOD and reduced GSH in treated animals (Figure 3, A-D). But, treatment with rosuvastatin regained the enzyme activity by increasing the level of SOD and reduced glutathione level (p<0.05) compared to CCl4 treated animal group (Figure 3, A-D). Furthermore, the activity of myeloperoxidase (MPO) in stressed and injured tissue is increased. In this experiment liver MPO activity significantly (p<0.05) increased in CCl4 treated animal group (Figure 3, E). Rosuvastatin significantly reduced the MPO activity in the treated animal group compared
to CCl4 treated animal group (Figure 3, E)
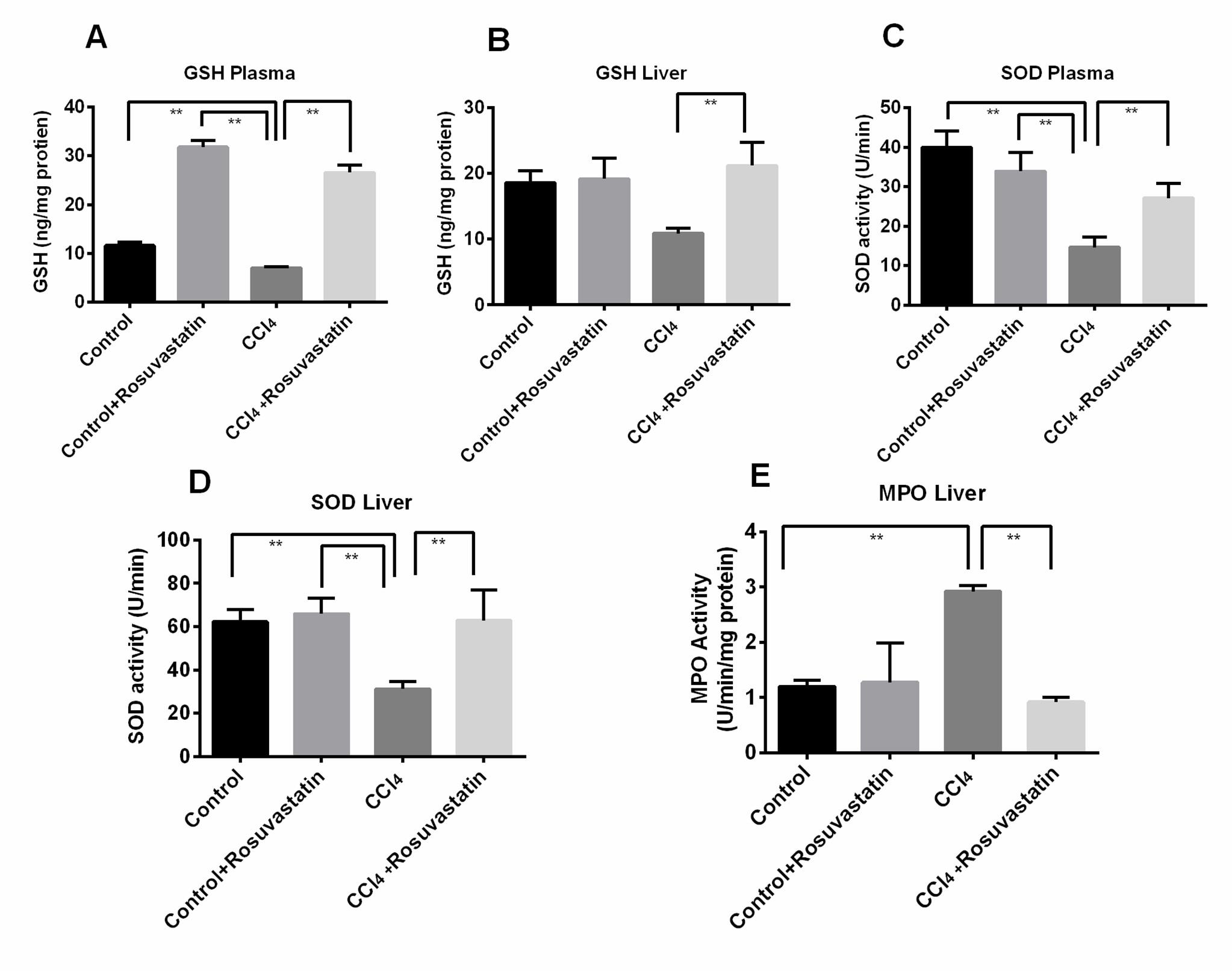
Effect of rosuvastatin on hepatic inflammation and fibrosis in CCl4 administered rats
Histological staining of liver sections was presented in Figure 4. Control rats showed normal structural orientation in the liver section in H and E staining (Figure 4 A). Control rats treated with rosuvastatin also showed normal structural orientation in liver section (Figure 4B). CCl4 administration induces hepatic damage and necrosis followed by infiltrating cells in the scar region (Figure 4C). Rosuvastatin treatment in CCl4 administered rats prevented the inflammatory cells infiltration and normalized the liver structure as shown in control rats (Figure 4 D).
Furthermore, Sirius red staining was also conducted to evaluate the fibrosis in the liver section of CCl4 administered rats. Control rats showed base line collagen deposition in the hepatic arterial and bile duct region (Figure 4 E). Control rats treated with rosuvastatin also showed limited collagen deposition around blood vessel and bile duct region (Figure 4 F). However, CCl4 administered rats showed increased collagen deposition and fibrosis in liver (Figure 4 G), which were further ameliorated by the rosuvastatin treatment (Figure 4 H).
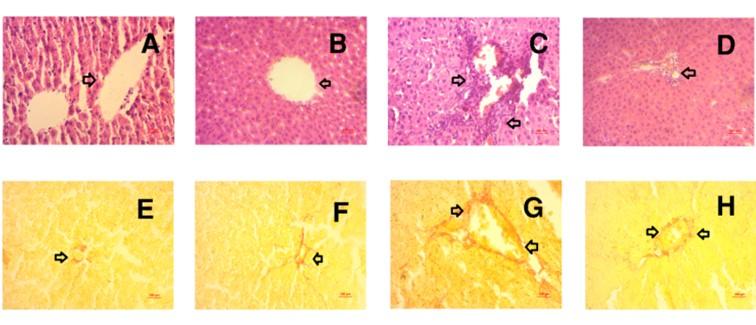
DISCUSSION
Cellular life emerges in an exceedingly belligerent chemical environment with profuse electrophilic stress rendered by reactive oxygen species (ROS) and reactive nitrogen species (RNS) in the primitive biosphere [19]. Oxidative stress, a common phenomenon in our body can result from an overabundance of reactive oxygen species (ROS) and lack of antioxidant potential [20]. CCl4, an extremely toxic chemical is widely used for the study of investigational hepatic abnormalities. Several studies have proposed some of the fundamental mechanism involving tissue damage induced by CCl4, like lipid peroxidation, reactive free radical metabolites, metabolic activation, covalent binding and disturbance of calcium homeostasis [21]. The present study suggests that rosuvastatin protected against hepatocyte injury evoked by administration of CCl4 in the rat. Moreover, rosuvastatin restored the antioxidant enzymes function and prevented fibrosis in the liver of CCl4 administered rats.
CCl4 has been used as a hepatotoxin in the experimental animal model. The characteristic features of this noxious agent in the liver are the elevation of various liver maker activities such as AST, ALT and ALP [22]. These enzymes are present in the hepatocyte and when any hepatocyte injury takes place in the liver, these enzymes will come to plasma. The present study also indicates that rosuvastatin protected against hepatocyte injury significantly by lowering plasma AST, ALT and ALP activities which were evoked by administration of CCl4 in the rat.
Oxidative stress, a common phenomenon in our body can result from an overabundance of oxygen free radical (ROS) and lack of antioxidant capability [20]. Oxidation of CCl4 by cytochrome P450 produces tri-chloromethyl free radicles which are the crucial factor of tissue damage induced by CCl4. In this study, CCl4 administration increased the free radical generation and amplified lipid peroxidation significantly (p<0.05), which is comparable to the control. Rosuvastatin, one of the most well-known lipids lowering agent have some pleiotropic effects like restoring endothelial functions, diminishing oxidative stress and vascular inflammation, stabilizing atherosclerotic plaques etc. [23]. Moreover, rosuvastatin increases PPAR expression and reduce oxidative stress, inflammation and atherosclerosis [24]. Our current study exhibited the hepatoprotective effect of rosuvastatin by preventing oxidative stress by lowering MDA concentration in plasma and tissues of CCl4 administered animals.
Nitric oxide is a crucial physiological molecule, acting as a signaling molecule in biological system. However, in association with other ROS (superoxide anion [–O·2]), nitric oxide immediately reacts with superoxide to produce more reactive peroxynitrite (–ONOO·) and may cause nitrosative stress in tissues. Increased nitric oxide level was observed in plasma and liver tissue of CCl4 administered rats significantly (p<0.05) compared to the control rats. Rosuvastatin treatment prevented the rise of nitric oxide in CCl4 administered rats significantly (p<0.05).
Another stress marker is known as advanced protein oxidation product (APOP). APOP concentration was also increased both in plasma and liver tissues in CCl4 administered rats significantly (p<0.05) compared to the control rats, which were also reduced by rosuvastatin treatment in CCl4 administered rats.
Protection against oxidative stress and lipid peroxidation can be exerted by cellular antioxidant defense present as superoxide dismutase (SOD) and reduced glutathione (GSH). CCl4 administration in rats lowered the SOD activities both in plasma as well as liver tissues significantly (p<0.05), which is comparable to the control rats (Figure 3). Rosuvastatin restored the activity of antioxidant function of enzymes such as SOD activities in the liver of CCl4 administered rats. Moreover, reduced GSH concentration was also decreased significantly (p<0.05) in CCl4 administered rats, which was also restored by rosuvastatin treatment.
Recent studies revealed that liver plays an important role in inflammatory responses because of involvement of dietary components, and even, nutrition diets may increase inflammatory components in atherogenesis [25]. Oxidative stress and tissue injury further attract enormous amount of inflammatory cells in to the injured site. Staining of liver section for microscopic study also indicated inflammatory cells infiltration alongside the portal vein in CCl4 administered rats compared to the control rats. Moreover, tissue MPO activities were also found increased in CCl4 administered rats significantly (p<0.05) compared to the control rats, which is a sign of inflammation in the tissue. Rosuvastatin prohibited infiltration of inflammatory cells and reduce the activity of MPO in CCl4 administered rats.
Oxidative stress and inflammation may also trigger extracellular matrix (ECM) deposition in the tissue. Oxidative stress also initiates dormant hepatic stellate cell activation and altered them into myofibroblast via the influence of transforming growth factor beta 1 (TGF-β1). Myofibroblasts responsible for the elevation of oxidative stress on hepatocyte by the NADPH oxidase pathway can also be activated by reactive oxygen species (ROS), cytokines, and chemokines released by an active kupffer cell that also increases production of nitric oxide (NO) by increasing inducible nitric oxide synthase (iNOS) which ultimately results in promoting nuclear factor Kappa B (NF-κB) [26]. Pro-inflammatory cytokines that result in the development of hepatic inflammation, fibrosis and cirrhosis are also released by oxidative stress [27]. When the liver becomes fibrotic a drastic change like 3-5 fold increase of collagens and non-collagenous components are found in hepatic ECM’s composition [28]. Thus, development of hepatic cirrhosis is a consequence of progressive hepatic fibrosis and prolonged hepatic inflammation induced by oxidative stress [29]. In liver, Kuffer cells and hepatic stellate cells (HSCs) are the responsible cell types to release ECM in the tissues and causes fibrosis [30]. ROS and cytokines mediated signal may promote the HSCs to become activated and increases the collagen deposition in the scar site [31]. In this study, CCl4 mediated oxidative stress and inflammation also increased collagen deposition in the liver. Furthermore, rosuvastatin treatment decreased the collagen deposition in CCl4 administered rats.
CONCLUSIONS
In conclusion, this study exhibits the hepatoprotective effect of rosuvastatin by reducing the liver enzyme activities, elevating the antioxidant enzyme activities and decreasing oxidative stress markers of CCl4 administered animal models. This investigation revealed the beneficial role of rosuvastatin in hepatic dysfunction; this information can be utilized for the treatment of liver disorders in human such as fatty liver and non-alcoholic steatohepatitis. Further study is warranted to establish the efficacy in clinical trials.
ACKNOWLEDGEMENT
Authors acknowledge the authority of Department of Pharmaceutical Sciences, North South University, Bangladesh for providing the logistic support to carry out the project.
FUNDING
This research did not receive any grants/funds from any commercial, non- Government and/or Government organizations.
CONFLICTS OF INTEREST
The authors declare no conflict of interest.
References
- [1]Younossi ZM, Stepanova M, Afendy M, Fang Y, Younossi Y, Mir H, et al. Changes in the prevalence of the most common causes of chronic liver diseases in the United States from 1988 to 2008. Clinical Gastroenterology and Hepatology. 2011;9:524-30.
- [2]Bataller R, Brenner DA. Liver fibrosis. Journal of clinical investigation. 2005;115:209.
- [3]Sawant SP, Dnyanmote AV, Shankar K, Limaye PB, Latendresse JR, Mehendale HM. Potentiation of Carbon Tetrachloride Hepatotoxicity and Lethality in Type 2 Diabetic Rats. Journal of Pharmacology and Experimental Therapeutics. 2004;308:694-704.
- [4]Wang AL, Wang JP, Wang H, Chen YH, Zhao L, Wang LS, et al. A dual effect of N-acetylcysteine on acute ethanol-induced liver damage in mice. Hepatology research : the official journal of the Japan Society of Hepatology. 2006;34:199-206.
- [5]Weber LWD, Boll M, Stampfl A. Hepatotoxicity and Mechanism of Action of Haloalkanes: Carbon Tetrachloride as a Toxicological Model. Critical Reviews in Toxicology. 2003;33:105-36.
- [6]Habibi J, Whaley-Connell A, Qazi MA, Hayden MR, Cooper SA, Tramontano A, et al. Rosuvastatin, a 3-hydroxy-3-methylglutaryl coenzyme a reductase inhibitor, decreases cardiac oxidative stress and remodeling in Ren2 transgenic rats. Endocrinology. 2007;148:2181-8.
- [7]Seo M, Inoue I, Ikeda M, Nakano T, Takahashi S, Katayama S, et al. Statins Activate Human PPAR PPAR research. 2008;2008.
- [8]Argo CK, Loria P, Caldwell SH, Lonardo A. Statins in liver disease: a molehill, an iceberg, or neither? Hepatology. 2008;48:662-9.
- [9]Olsson AG, McTaggart F, Raza A. Rosuvastatin: A Highly Effective New HMG‐CoA Reductase Inhibitor. Cardiovascular drug reviews. 2002;20:303-28.
- [10]Jasiñska M, Owczarek J, Orszulak-Michalak D. Statins: a new insight into their mechanisms of action and consequent pleiotropic effects. Pharmacological Reports. 2007;59:483.
- [11]Awad AS, Kamel R. Effect of rosuvastatin on cholestasis-induced hepatic injury in rat livers. Journal of biochemical and molecular toxicology. 2010;24:89-94.
- [12]Shirin H, Sharvit E, Aeed H, Gavish D, Bruck R. Atorvastatin and rosuvastatin do not prevent thioacetamide induced liver cirrhosis in rats. World journal of gastroenterology. 2013;19:241-8.
- [13]Niehaus WG, Samuelsson B. Formation of malonaldehyde from phospholipid arachidonate during microsomal lipid peroxidation. European Journal of Biochemistry. 1968;6:126-30.
- [14]Tracey WR, Tse J, Carter G. Lipopolysaccharide-induced changes in plasma nitrite and nitrate concentrations in rats and mice: pharmacological evaluation of nitric oxide synthase inhibitors. Journal of Pharmacology and Experimental Therapeutics. 1995;272:1011-5.
- [15]Witko-Sarsat V, Friedlander M, Capeillère-Blandin C, Nguyen-Khoa T, Nguyen A, Zingraff J, et al. Advanced oxidation protein products as a novel marker of oxidative stress in uremia. Kidney Int. 1996;49:1304-13.
- [16]Tiwari BK, Kumar D, Abidi AB, Rizvi SI. Efficacy of Composite Extract from Leaves and Fruits of Medicinal Plants Used in Traditional Diabetic Therapy against Oxidative Stress in Alloxan-Induced Diabetic Rats. ISRN Pharmacology. 2014;2014:7.
- [17]Khan RA. Protective effects of Sonchus asper (L.) Hill, (Asteraceae) against CCl4-induced oxidative stress in the thyroid tissue of rats. BMC Complementary and Alternative Medicine. 2012;12:181.
- [18]Jollow D, Mitchell J, Zampaglione N, Gillette J. Bromobenzene-induced liver necrosis. Protective role of glutathione and evidence for 3,4-bromobenzene oxide as the hepatotoxic metabolite. Pharmacology 1974;11:151-69.
- [19]Sporn MB, Liby KT, Yore MM, Fu L, Lopchuk JM, Gribble GW. New synthetic triterpenoids: potent agents for prevention and treatment of tissue injury caused by inflammatory and oxidative stress. Journal of natural products. 2011;74:537-45.
- [20]Ha H-L, Shin H-J, Feitelson MA, Yu D-Y. Oxidative stress and antioxidants in hepatic pathogenesis. World journal of gastroenterology: WJG. 2010;16:6035.
- [21]Zhu W, Fung P. The roles played by crucial free radicals like lipid free radicals, nitric oxide, and enzymes NOS and NADPH in CCl 4-induced acute liver injury of mice. Free Radical Biology and Medicine. 2000;29:870-80.
- [22]Chowdhury MRH, Sagor MAT, Tabassum N, Potol MA, Hossain H, Alam MA. Supplementation of Citrus maxima peel powder prevented oxidative stress, fibrosis, and hepatic damage in carbon tetrachloride (CCl4) treated rats. Evid Based Complement Alternat Med. 2015;2015:10.
- [23]Takemoto M, Liao JK. Pleiotropic effects of 3-hydroxy-3-methylglutaryl coenzyme a reductase inhibitors. Arteriosclerosis, thrombosis, and vascular biology. 2001;21:1712-9.
- [24]Camps J, García-Heredia A, Rull A, Alonso-Villaverde C, Aragones G, Beltrán-Debón R, et al. PPARs in regulation of paraoxonases: control of oxidative stress and inflammation pathways. PPAR research. 2012;2012.
- [25]Kleemann R, Verschuren L, van Erk MJ, Nikolsky Y, Cnubben N, Verheij ER, et al. Atherosclerosis and liver inflammation induced by increased dietary cholesterol intake: a combined transcriptomics and metabolomics analysis. Genome Biol. 2007;8:R200.
- [26]Czaja AJ. Hepatic inflammation and progressive liver fibrosis in chronic liver disease. World journal of gastroenterology: WJG. 2014;20:2515.
- [27]Vuppalanchi R, Juluri R, Bell LN, Ghabril M, Kamendulis L, Klaunig JE, et al. Oxidative stress in chronic liver disease: relationship between peripheral and hepatic measurements. The American journal of the medical sciences. 2011;342:314-7.
- [28]Friedman SL. Molecular regulation of hepatic fibrosis, an integrated cellular response to tissue injury. Journal of Biological Chemistry. 2000;275:2247-50.
- [29]Czaja AJ. Hepatic inflammation and progressive liver fibrosis in chronic liver disease. World J Gastroenterol. 2014;20:2515-32.
- [30]Elpek GÖ. Cellular and molecular mechanisms in the pathogenesis of liver fibrosis: An update. World Journal of Gastroenterology : WJG. 2014;20:7260-76.
- [31]Zhang C-Y, Yuan W-G, He P, Lei J-H, Wang C-X. Liver fibrosis and hepatic stellate cells: Etiology, pathological hallmarks and therapeutic targets. World Journal of Gastroenterology. 2016;22:10512-22.