Biochemical and in silico study of leaf extract from Rumex dentatus against Staphylococcus aureus
Abstract
Rumex dentatus is a medicinal plant with a variety of bioactive components that can be used to treat infections and multidrug-resistant (MDR) microbes. Infections caused by MDR microbes may pose a real threat and the available antibiotics can no longer be used to control or kill those bacteria. Thus, the study aimed to ascertain the antibacterial activity of Rumex dentatus leaf extract and identify a potent compound that can be used as a drug developmental agent may against Staphylococcus aureus. Disc diffusion method, mortality assay of brine shrimp, and DPPH free radical scavenging assay were carried out for in vitro analysis. Standard computational tools and servers such as Pymol, PyRx, and Discovery Studio were used for carrying out the in-silico studies. Staphylococcus aureus was isolated from the infected area of eczema sufferers and identified through morphological, biochemical, and 16SrRNA sequence analysis. Rumex dentatus leaf extract was diluted with methanol and it showed the maximum zone of inhibition (14.33 ± 0.68 mm) after applying it against Staphylococcus aureus at the dose of 150µg/disc. Furthermore, the extract revealed remarkable antioxidant activity and the death rate of brine shrimp and leaf extract concentration were positively connected. Finding pharmacological targets with a high affinity for the FosB (4nb2) protein, which controls antibiotic resistance in Staphylococcus aureus, was done via molecular docking. 1-alpha-18O-1,25-dihydroxycholecalciferol (CID-5280453), androstan-3-ol, 9-methyl-(3 beta,5 alpha) (CID-22215820), phenylacetaldehyde (CID-998), and benzenepropanoic acid, 3,5-bis(1,1-dimethylethyl)-4-hydroxy- methyl ester (CID-62603) substances had binding energies of -6.8 Kcal/mol, -6.6 Kcal/mol, -5.6 kcal/mol, and -5.5 kcal/mol, respectively. As an organic source of medicines, Rumex dentatus leaf extract could be used to combat the antimicrobial-resistant pathogen.
Keywords
INTRODUCTION
Eczema, often known as dermatitis, is a group of diseases that are irritating, inflammatory, and cause skin rashes [1]. Atopic dermatitis (AD) is the most common type of eczema, characterized by dry, inflamed, and itchy skin, and it refers to a group of disorders caused by allergic reactions, including asthma and hay fever. AD has been more common in recent decades, with 10-30% of children in affluent nations suffering [2]. Atopic eczema can be caused by a variety of infectious agents and microorganisms such as Staphylococcus aureus, Candida albicans. Although candida, a yeast infection spread on weepy and heated skin, can cause eczema, the common bacterium Staphylococcus aureus is primarily blamed for the condition. The isolated strain Staphylococcus aureus, which thrives on moist and damaged skin, causes atopic eczema. As the infection originates in the bloodstream, Staphylococcus aureus infection can spread by contact with fluids into the blisters located on the affected skin [3]. Bacteria can genetically change themselves to become antibiotic-resistant. The main issue has been identified as people’s overuse of antibiotics, which causes bacteria to become resistant to antibiotics, resulting in an unclear future. Multidrug-resistant (MDR) organisms that are resistant to multiple antibiotics or antifungals are difficult to treat, and therefore can causes serious illness or even death. It is a better strategy to control the usage of antibiotics by producing natural drugs that aim to provide patients with the appropriate antimicrobial agents [4]. Plant extracts were used to obtain antimicrobial compounds, as there are around 120 plant species belonging to 28 distinct families [5] that contain various phytochemicals and are excellent sources of natural antioxidants for public health. Infectious bacteria species pose a multitude of contagious diseases [6] as a consequence of antibiotic and drug resistance [7]. S. aureus bacteria are the most common microbes that cause a variety of diseases in humans. It is critical in the treatment of bacteremia and necrotizing enterocolitis, as well as osteoarthritis, respiratory infections, pleuropulmonary infections, and device-related diseases [8]. Different medicinal plant extracts offer effective antibacterial properties against bacteria and fungi that cause a variety of skin diseases. Flavonoids and anthraquinones have been identified as important chemical components of this species in several studies [9]. It includes several pharmaceutically important substances that can help with a variety of conditions, including infection [10]. Rumex dentatus leaves were shown to have refrigerant characteristics [11]. Rumex dentatus extracts have potential antibacterial, antifungal, cytotoxic, anticancer, and allopathic properties [12]. These leaves also contain several important bioactive chemicals, including palmitic acid, hexestrol, phenylacetaldehyde, and vitamin C. According to World Health Organization (WHO), a wide range of plant extracts might produce a variety of pharmaceuticals., including medicines with effective healing properties [13]. Secondary metabolites, such as phenolic compounds found in essential oils and tannins [14], are plant extracts containing diverse types of phytochemicals that resist microorganisms. According to the documentary, Staphylococcus aureus was susceptible to 81 extracts found in 58 plants [15]. Due to its numerous uses in the analysis of molecular recognition events such binding energetics, molecular interactions, and induced conformational changes, molecular docking is one of the most widely utilized structure-based drug design methodologies. Using bioactive small-molecule libraries is a cutting-edge approach to drug development. The specific chemical space occupied by ligands known to interact with a particular target is represented by these libraries. he goal of ligand-protein docking is to predict the predominant binding modes of a ligand with a protein of known three-dimensional structure.
The purpose of this research is to investigate the antimicrobial property of Rumex dentatus leaf extracts in the laboratory and in silico approach to determine the potential compound of the Rumex dentatus leaf extract, which can be used in the development of drugs against Staphylococcus aureus. One of the causes of atopic dermatitis is infectious microorganisms. Antibiotics are urgently needed to combat such microorganisms since antibiotic resistance is making the situation worse. Therefore, if we can stop the protein that causes atopic dermatitis, it will aid in the fight against the condition. Rumex dentatus plant extracts’ antagonistic properties against the bacterium were also investigated.
MATERIALS AND METHODS
The following materials and methods were used for the completion of this research work.
Bacterial isolation and identification
The sample was taken from blisters that had formed in the infected area of eczema sufferers. The procedure was carried out using the swabbing technique, which involved rubbing a sterile dry cotton-tip swab on the blister of an infected skin location. The inoculated cotton tip was then placed in a conical flask or beaker containing saline water. After collecting the sample, it was serially diluted. Due to growing bacteria overnight in the mineral salt (MS) medium at 37 °C the diluted solution was mixed with the media. The colony was selected depending on the shape, and size of the single colony, and the streaking method was used for producing pure culture due to further use. For studying the characteristics of the bacteria morphological and biochemical tests were carried out such as gram staining test, mobility test, methyl red test, hemolysis, pigment test, eosin methylene blue agar test, urease test, catalase test, methyl red test, mannitol test, starch hydrolysis test, triple sugar iron test, and citrate test.
Evaluation of 16S rRNA gene sequencing
The Genomic DNA of the pure bacterial isolate was extracted. Here, universal reverse and forward primers, namely, 1492R (5’- GGTTACCTTGTTACGACTT-3’) and 27F (- 5’-AGAGTTTGATCCTGGCTCAG-3’-) were used. After the completion of PCR, the amplified PCR products were sent for 16S rRNA sequencing through a commercial sequencing service of Invent Technologies Ltd. Dhaka, Bangladesh. The 16S rRNA genes of the isolate were sequenced for checking the homology matched to other sequence data in the gene database server utilizing the Basic Local Alignment Search Tool (BLAST)(www.ncbi.nlm.nih.gov/Blast) [16].
Plant sample collection
Rumex dentatus leaves were collected from various locations in the Rajshahi University (Bangladesh) area, and the individuals placed in a sterilized zipper paunch were microbes free, and then the zipper paunch was taken to the Microbiology Lab in the Department of Genetic Engineering and Biotechnology University of Rajshahi. The plant was identified (Voucher no. 47) by Dr. A.H.M. Mahbubur Rahman, Professor, Department of Botany, University of Rajshahi, Bangladesh
Preparation of extracts
The sample of plant leaves was obtained and dried with the help of air at room temperature in the research lab for one week. A blender was used to grind the leaves. Mariswamy et al. [17] described a technique for preparing methanolic extracts in which 50 g of the specimen was diluted with 250 mL of methanolic solvent extract and positioned in an orbital shaker for shaking for five days. For clearing the unwanted particle from the sample filtered paper was used before being accumulated in an open jar for drying and the extract was stored at 4 ºC for further use.
Antibacterial activity test
The antibacterial activity of the isolate the test was carried out through the protocol explained by Nostro et al. [18]. For performing the test 6 mm discs of Whatman paper were used. 1 ml methanol was added to 0.05 gm extract for preparing the stock solution. The disinfected discs were then immersed in diluted extracts at 50, l00, 150, and 200 μg/ml concentrations. Finally, each agar plate was seeded with 3.5×108 CFU/mL of bacteria, and the medicated discs were positioned with a sterile loop on each plate. As a regulation, gentamicin was used. The plates were then managed to keep at 37 °C overnight for observation. The presence of distinct zones indicated that the growth of microorganisms was not rapid. The zones in the vicinity of the discs were measured and recorded.
Cytotoxicity assay
A cytotoxic test determines whether a substance is hazardous to cells. It is a frequently used method for determining the hazardous level of a naturally occurring plant extract or any other compound. Meyer et al. [19] described a cytotoxicity test that was done on brine shrimp (Artemia salina) nauplii.1 ml methanol was added to 0.05 gm extract for preparing the stock solution. In a tank, brine shrimp were generated at 25 °C temperature. In test tubes, extract concentration levels of 50, 100, 150, and 200 μg/mL were managed to make. After that, 10 live Artemia salina nauplii were placed in each test tube and left at room temperature for 24 h. Finally, the LC50 values of methanolic leaf extracts were calculated and recorded.
Antioxidant activity test
The antioxidant potential of the leaf extracts was determined using the DPPH (2,2-Diphenyl-1-Pi cryl-Hydrazyl-Hydrate) free radical scavenging assay with BHT (Butylated Hydroxy Toluene) as a control [20]. BHT concentration levels varied from 50, 100, and 150 μg/ml. Each test tube obtained 1.5 mL of 0.1 mM DPPH solution. Finally, by adding methanol to all the test tubes, the total volume was increased to 2.5ml. The test tubes were then retained in the dark room for 30 minutes time duration to complete the interaction. Finally, using a spectrophotometer and BHT as a control, absorbance at 517 nm was measured [21]. The scavenging portion was sorted out using the equation narrated by Mahmud et al. [22] and the IC50 was determined by calculating by projecting the results on a linear scatter graph in a graph pad prism.
Antibiotic sensitivity test
The Kirby-Bauer disc diffusion model was used to calculate the antibacterial drug responsiveness and resistance pattern of the bacterial isolate [23]. The isolated bacterial strain was cultivated and incubated at 37 °C for the antibiotic’s susceptibility test. The LB agar medium was prepared and commercially available, and antibiotics that were regularly prescribed (gentamycin, fosfomycillin, amoxicillin, ciprofloxacin, cefuroxime) were inserted in the center of the plates and incubated at 37 °C. The zones on the plate were noticed and measured using an mm scale after such a nighttime incubation period.
Molecular docking study
Ligand preparation
For docking analysis ligands were determined according to Rumex dentatus GC-MS compounds, a total of 17 Rumex dentatus GC-MS compounds were chosen as docking ligands [24]. The literature following a conscientious examination of the literature, the chemical substances of Rumex dentatus [25, 26] were reprocessed from the PubChem database of chemical molecules and their activities against biological assays [27]. Employing a Merck molecular force field (MMFF94), the substances were extracted in three dimensions as well as confined to minimize the objective function.
Protein preparation
The protein data library provided the crystalline structure of the S. aureus protein (PDB ID: 4nb2). Using Pymol software (version 2.4.0) and Discovery Studio software, the protein structure was washed, and heteroatoms were eliminated (version 4.5.0). In Swiss PDB Viewer software (version 4.1), the washed protein was energy reduced and streamlined using the Groningen molecular Simulation (GROMOS) 431B force field. The Ramachandran plot analysis was utilized to determine the quality geometry of the protein structure.
Molecular docking
With the help of AutoDock Vina software package (version 1.1.2) molecular docking analysis was carried out for appreciating the binding kinetics of substances from Rumex dentatus and the target protein [28, 29]. For converting the protein structure into molecules, the software had been used and after applying it ligand molecules became converted into PDBQT layout. Ligand-protein interactions can be defined with the help of Lamarckian genetic algorithms. Binding free energy and docking directions within a 2.0 Root-Mean-Square Deviation (RMSD) range were approved to alternate the molecules, which is considered a key advantage. A range of 10, 20 modes, and an exhaustiveness of 8 was used to complete molecular docking. In Pymol [30] and Discovery Studio [31], the autodock structures were eventually examined for non-bonded contacts.
RESULTS
Morphological and biochemical tests of isolates
The sample was collected from eczema-affected skin and then plated onto Mannitol Salt agar media which is selective for pathogenic bacteria. In the staining test, the isolated bacteria showed purple color indicating gram-positive spherical-shaped bacteria and motile. Biochemical tests indicated that the isolate was negative in the MacConkey test, TSI test, Bismuth sulfate agar (BSA) test, EMB agar test, and positive for Methyl red test, Catalase test, Mannitol test, Starch hydrolysis test, Urea hydrolysis test, and citrate test .In hemolysis and pigment tests on blood agar, it showed often-creamy gold pigment by producing various types of hemolysins (alpha, beta, gamma, and delta) (data are not shown).
Species identification of the isolate
Figure 1A illustrated that the length of 16S rRNA of the isolate was approximately 1500bp. After sequencing and editing the gene, the sequence was compared to other organism 16S rRNA gene sequences which had been uploaded to the Gene dataset of NCBI. Relating to substance numerous sequences were manifested for the isolate. Staphylococcus aureus formed a 99 percent identity with the 16S rRNA gene sequencing of the isolate bacteria (Figure 1B).
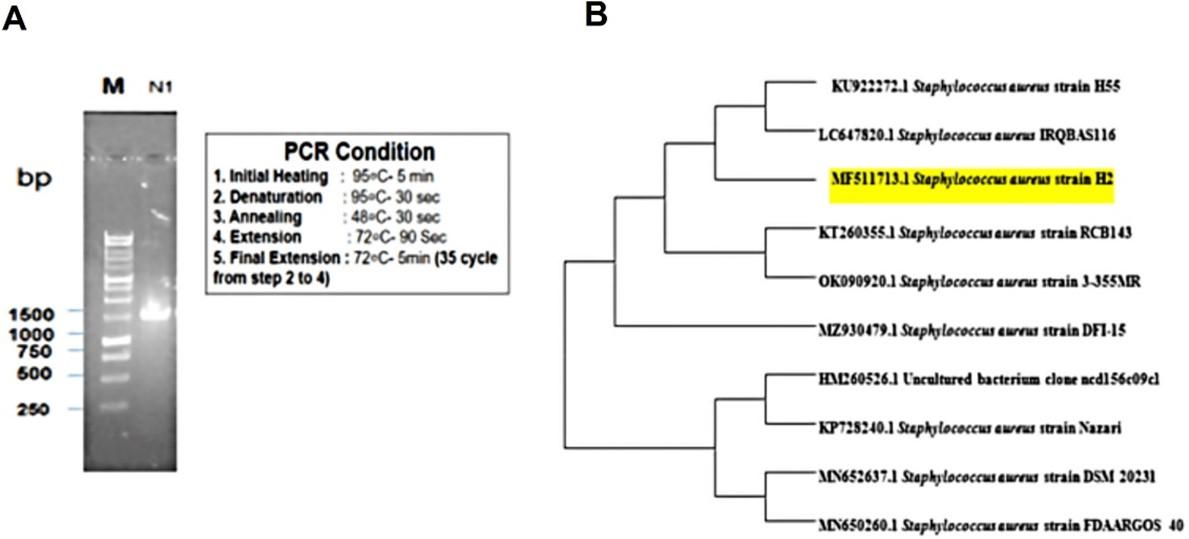
Effect of Rumex dentatus leaf extract against activity of Staphylococcus aureus
Figure 2A and 2B illustrates Rumex dentatus antibacterial activity. Methanolic extraction of Rumex dentatus leaf showed 12.52 ± 0.48 mm, 13.12 ± 0.53 mm, 14.33 ± 0.68 mm, 14.18 ± 0.54 mm inhibition zones against Staphylococcus aureus at a concentration of 50, 100, 150 and 200µg/disc, respectively.
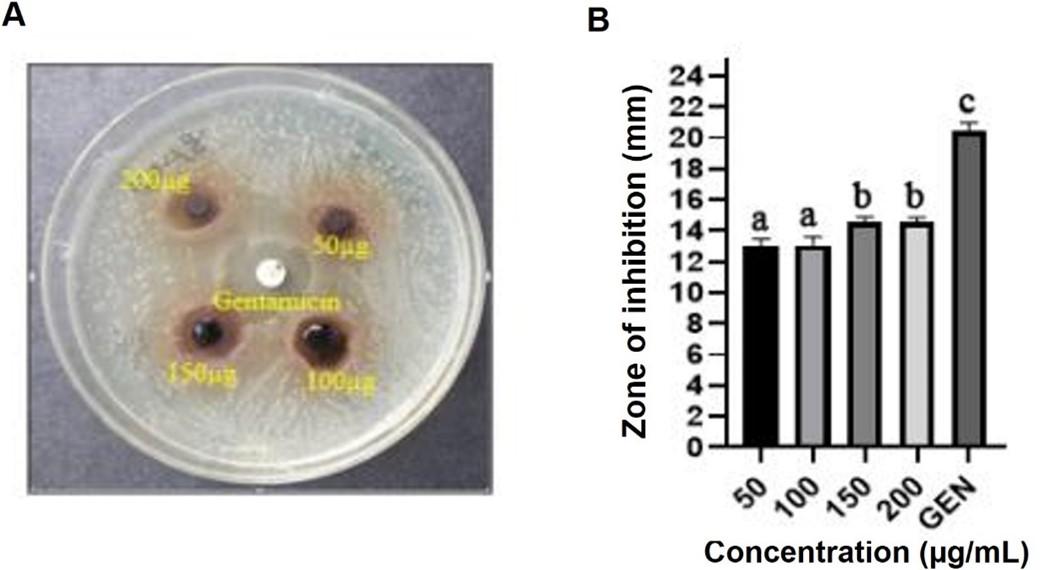
Effect of methanolic extract of Rumex dentatus leaf on cytotoxic activity
The LC50 values of methanolic Rumex dentatus leaf extracts were 296.57 ± 4.08 µg/mL. These findings suggest a strong positive correlation between the density of leaf extract and the fatal rate of brine shrimp, with a maximum mortality percentage at 200 µg/mL dose compared to other dosages and on the other hand a lower or minimum mortality percentage at 100 µg/mL dose (Figure 3).
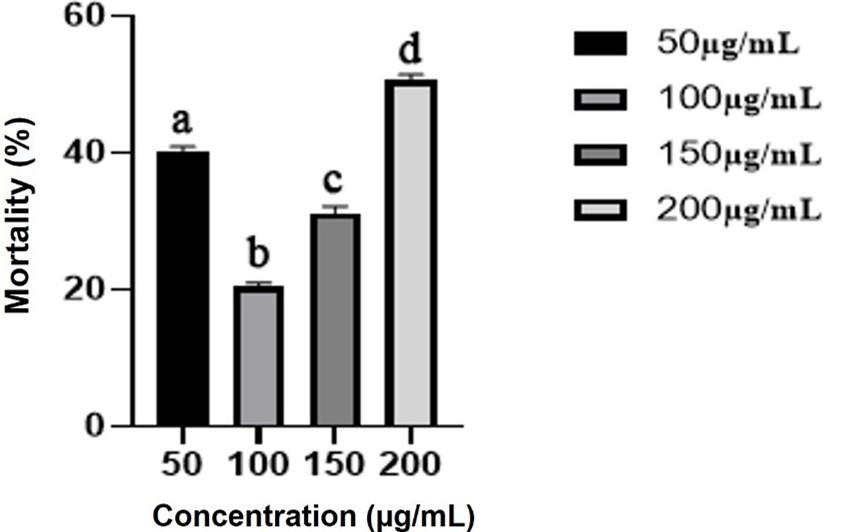
Effect of Rumex dentatus leaf extract on antioxidant activity
At 150 µg/mL concentration, Rumex dentatus leaf extract had 56.21 % DPPH free radical scavenging activity, whereas BHT control activity was 83.46 % (Figure 4A). Leaf extract and BHT had IC50 values of 139 ± 3.14 µg/mL and 54 ± 2.01 µg/mL, respectively (Figure 4B). These outcomes show that perhaps the leaf extract had modestly potent antioxidant activity, especially in comparison to the BHT control.
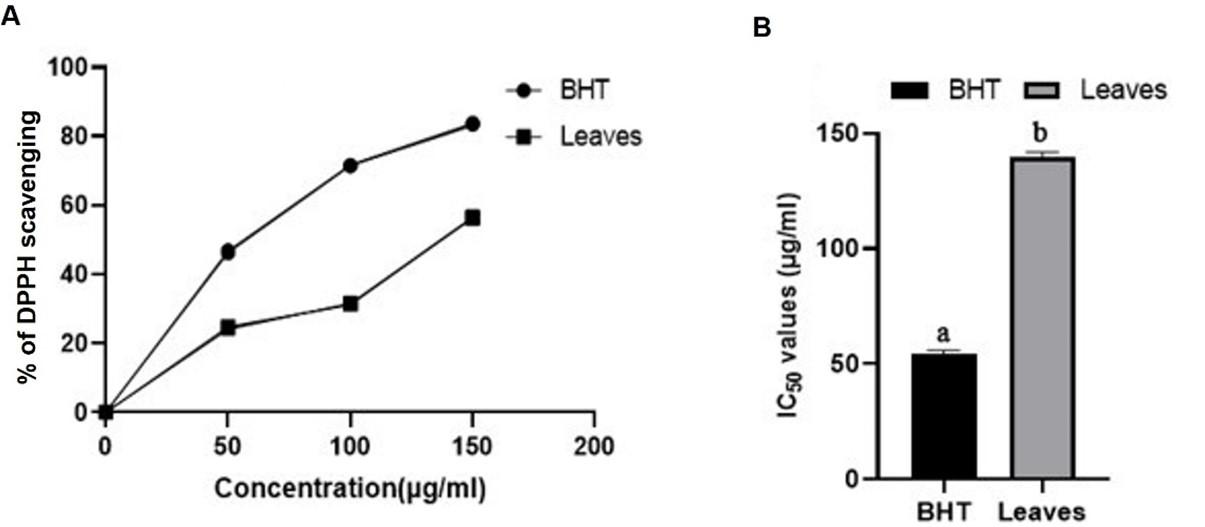
Resistance pattern of the isolate
Staphylococcus aureus antibiotic responsiveness was evaluated utilizing five antibiotic disks: amoxicillin, gentamycin, ciprofloxacin, cefuroxime, and fosfomycin. Utilizing nutrient agar media, the standard disc diffusion model was used to narrate the trends of responsiveness and resistance of isolated bacterial cultures to five different antibiotics. Findings showed that Staphylococcus aureus was resistant to ampicillin, cefuroxime, and fosfomycin but responsive to gentamicin and ciprofloxacin (Figure 5A). Figure 5B illustrates the results of antibiotic sensitivity assessment and antibiotic inhibition against the bacterium.
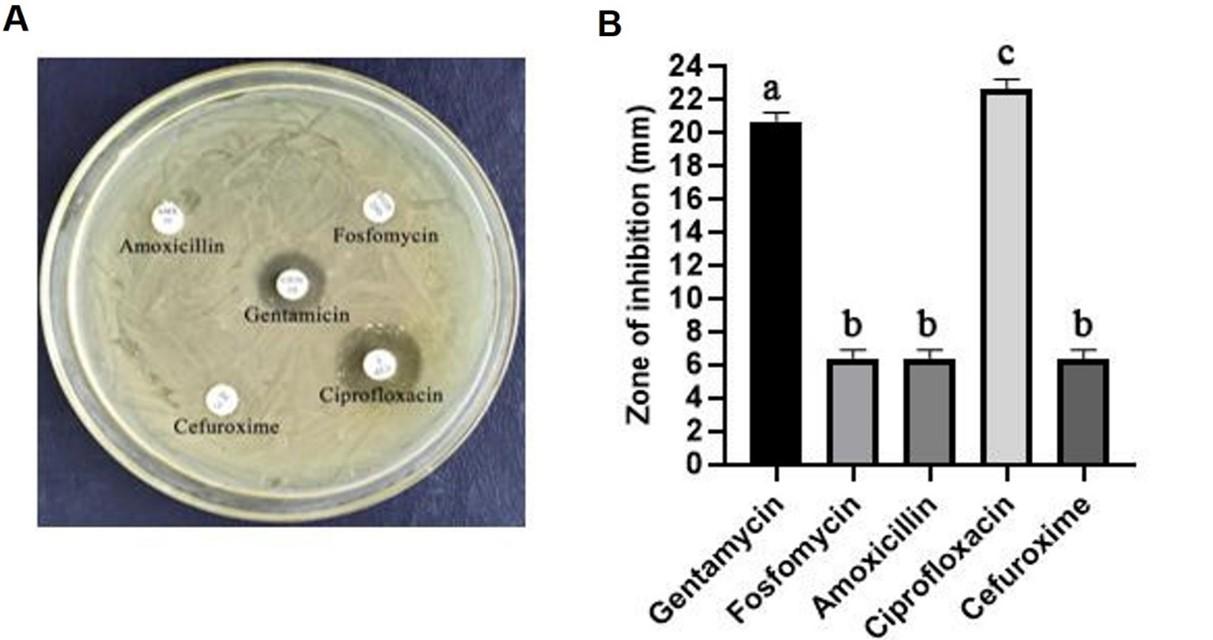
Ligand-protein interaction analysis
Molecular docking analyses were carried out for determining the interconnection among the bond and identifying the major molecules with a stronger relationship with the FosB (4nb2) protein complex. All 17 compounds from GC-MS analysis of methanolic leaf extracts of Rumex dentatus were used for molecular docking. Among them, only four phytochemicals from Rumex dentatus were scanned for the lesser binding score in this study. CID- 5280453, CID- 22215820, CID- 998, and CID- 62603 were assigned as D1, D2, D3, and D4, respectively. D1 and D2 compounds had a stronger affinity than other Rumex dentatus chemicals. -6.8 Kcal/mol and -6.6 Kcal/mol were the binding affinity score levels of D1 and D2 substances respectively (Table 1).
The D1 from Rumex dentatus interacted with the FosB protein and formed two hydrogen bonds (Figure 6 and Table 2) at Phe62, Thr65, alkyl bonds at Ile8, Ile55, Ile67, and pi-alkyl bonds at His112, His112, Phe10, and Tyr64, Phe62(3) positions. The D2 and 4nb2 protein complex formed one pi sigma bond at Trp46, alkyl bonds at Leu31 (2), Leu32(2), and pi-alkyl bond at Tyr39(2), and Trp46(2) (Figure 6). However, D3 had a binding score of -5.6 kcal/mol and had four pi-alkyl bonds at Ala43, Leu26, Leu42, and Leu116 residues (Figure 7). The D4 and 4nb2 protein complex was formed one pi sigma bond at Ile8, two pi-alkyl bonds at His112, Phe10 and one alkyl bond at Cys9 (Figure 7).
Table 1. Molecular docking score between Rumex dentatus plant compounds and selected protein.
Table 2. Non-covalent connections of compounds with specific proteins as well as their binding connections.
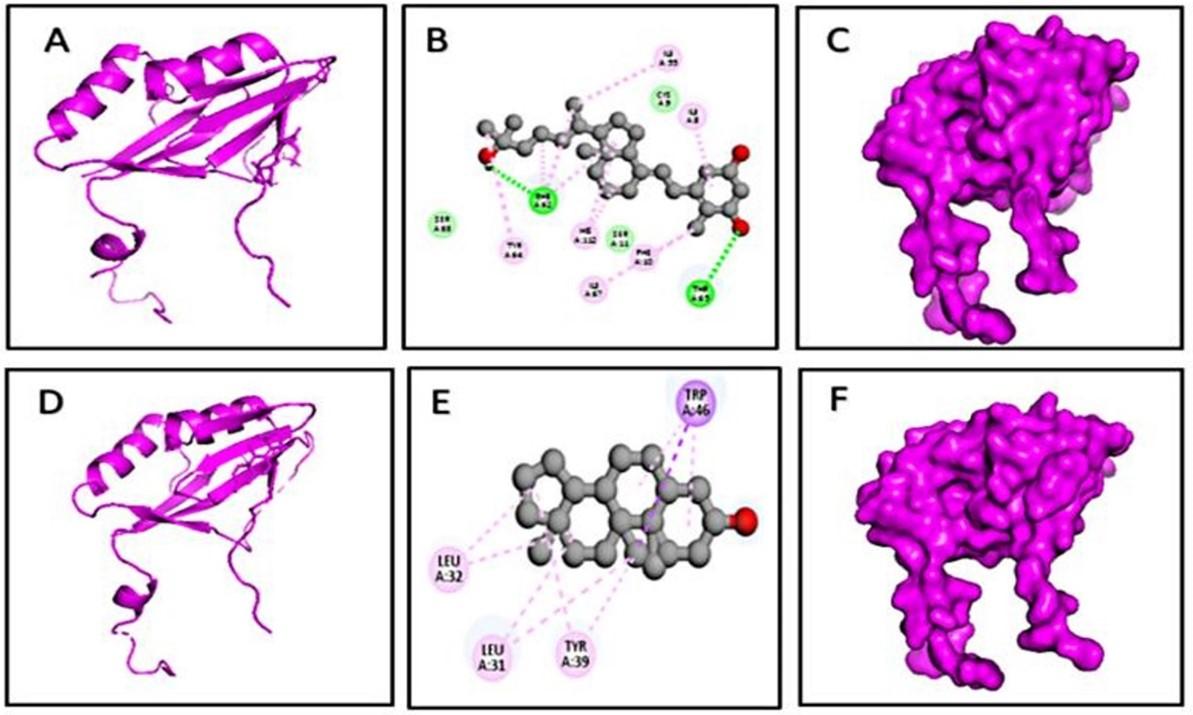
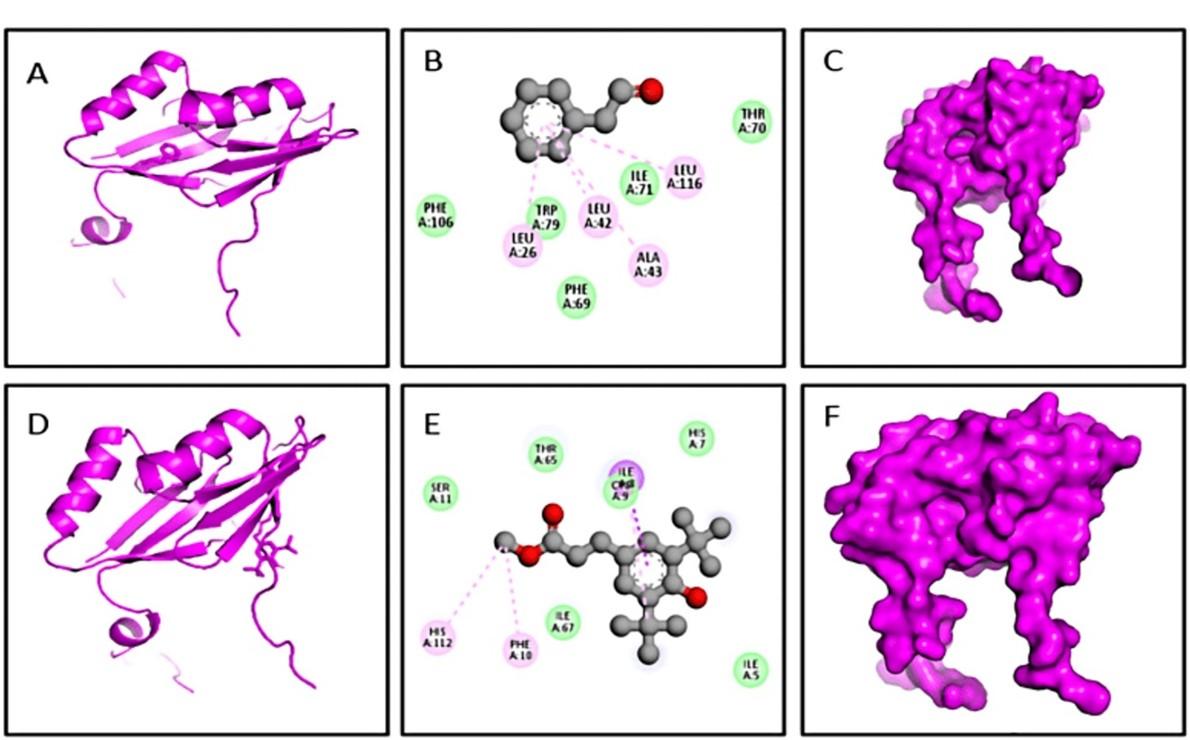
DISCUSSION
To serve as a defense against insects, fungi, and diseases as well as a treatment for human illnesses, medicinal plants manufacture hundreds of different chemical substances or compounds [32, 33]. The WHO has identified resistance to antibiotics as a national healthcare ultimatum to security which necessitates action from all levels of the government and community [34]. Plant-based phytochemicals have newly sparked considerable attention as a possible cause of novel pharmacological and therapeutic applications against bacterial infections [35,36]. Phytochemical analysis of plant extracts revealed the presence of parts known to illustrate herbal and biological activities [37]. Phytochemicals such as phenolic acids, tannins, flavonoids, saponins, glycosides, steroids, terpenoids, as well as alkaloids were discovered in leaf extracts. The phenolic compounds are among the most numerous and widespread groups of phytochemical constituents [38]. They have bioactivity such as anticarcinogen, anti-apoptosis, antiatherosclerosis, antiaging, anti-inflammation, as well as angiogenic [39]. Chromosomal DNA and 16S rRNA gene sequencing of the isolate was carried out for molecular identification and the isolated strains showed 99% similarities with Staphylococcus aureus. In this study, the leaf extracts of Rumex dentatus were found effective against Staphylococcus aureus and showed significant inhibition zone ranging between 11-14.5 mm. The antimicrobial activity, fungicidal, cytotoxic, antimutagenic, and medicinal prospects of Rumex dentatus leave extracts were evaluated. Rumex dentatus extracts were proven to be effective against a variety of infectious strains, with zones of inhibition ranging from 10 to 13 mm [12]. Rumex dentatus leaves extracts were screened on Staphylococcus aureus bacterial strains and indicated significant antibacterial activity [40]. The brine shrimp analysis can be used as a distinct probe for the pharmacological consequences of plant extracts [19]. The effective relationship between leaf extract density and the fatal rate of brine shrimp mortality was determined through the LC50 values of methanolic Rumex dentatus leaf extracts. According to Elzaawely et al., Rumex dentatus holds potential antioxidant activities that are correlated with its significant levels of phenolic compounds [41, 36]. Hence, they could be exploited as a natural source of antioxidants in the food industry. Numerous research has been done to determine the antioxidant effects of medicinal herbs that are rich in phenolics [42, 43].
These substances were compared to butylated hydroxyl toluene mostly based on how well they were able to neutralize DPPH free radicals (BHT), which was done to assess how effective an antioxidant they were. This plant’s extracts scavenged free radicals in a dose-dependent process, correlating with the findings of Siddhuraju et al. [44] and the antioxidant activity also contain in the other plant metabolites such as flavonoids, tannins, catechins, and other phenolics. The highest superoxide anion radical scavenging activity of Rumex dentatus methanol extract agrees with the findings of Jayasri et al., who found methanol to be the best superoxide radical scavenger at higher doses of plant extract [45].
Docking study evaluation is a reputable method for drug development [46]. By using molecular docking, we may clarify crucial metabolic processes while analyzing the behavior of small substances at the binding sites of target proteins [47,48,49]. Numerous studies can be undertaken as a result of the effort to find an inhibitor that is effective for the target proteins, and these studies are crucial for the development of computer modeling as well as for figuring out the dynamics of the ligand-protein complexes [50, 51]. Additionally, according to Ying et al., docking calculations can be utilized to separate successful applicant composites from a range of ligand studies [52] since targeted proteins can be repressed by arresting the active site of proteins [53]. In order to find effective inhibitors from a variety of datasets, molecular docking and molecular dynamics analysis might be quite helpful. To investigate the binding interaction and find lead molecules Rumex dentatus with a greater affinity for the crystalline structure of the S. aureus protein’s receptor-binding domain (PDB ID: 4nb2), molecular docking analysis was conducted in this case. Additionally, this study improves our comprehension of the relationship between ligand-protein and target binding affinity. Staphylococcus aureus, according to Bearman et al., is a leading cause of chronic mortality throughout the world [54, 55]. Antibiotic-adjusting enzymes in S. aureus naturally attain resistance to a wide range of antimicrobial properties, resulting in a multi-drug-resistant bacterium [56]. The fosfomycin-inactivating enzyme which is known as FosB and depends on Mn2+ mainly found in S. aureus, and it play important role in adding either L-cysteine (L-Cys) or bacillithiol (BSH) to the antibiotic, and that’s why the new compound is free of any bacteriostatic materials [57]. According to Kumar et al., Rumex dentatus is an affluent source of polyphenols and amino acids [58, 59]. The complexes of 1-Alpha-18O-1,25-dihydroxycholecalciferol and benzenepropanoic acid, 3,5-bis(1,1-dimethylethyl)-4-hydroxy-methyl ester had non-bonded connections with the active points SER 11 and multiple connections all around effective points of the protein target, that could be liable for the suppression of the target molecule. These chemicals were found to form hydrogen bonds, alkyl bonds, pi-alkyl bonds, and pi sigma bonds. The active site of the target receptor established numerous linkages, implying that they could inhibit and disrupt the actions of these amino acids [60, 61]. Here, 1-Alpha-18O-1,25-dihydroxycholecalciferol; Androstan-3-ol, 9-methyl-(3 beta,5 alpha); phenylacetaldehyde; and benzenepropanoic acid, 3,5-bis(1,1-dimethylethyl)-4-hydroxy-methyl ester compounds from Rumex dentatus showed the most effective result against FosB (4nb2) protein. As a result, compounds derived from Rumex dentatus plants may block the FosB protein’s receptor domain. However, for ensuring the stabilization properties MD simulation is needed. Moreover, Wet-lab synthesis and more in vivo studies are needed to corroborate these findings.
CONCLUSION
MDR microbes seem to be a greater threat to human health and social assistance. Healthcare providers struggle to treat pathogen infections, along with those caused by Staphylococcus aureus. Plants have largely replaced synthetic drugs as an organic resource of health aid alleviation for their less reaction, and higher particularity. In the treatment of bacterial infections that are resistant to antibiotics, Rumex dentatus extracts may suppress some strains. In this research, Staphylococcus aureus was isolated from eczema sufferers and confirmed through morphological, biochemical, and molecular techniques. The strain was resistant to Penicillin, Ampicillin, Amoxicillin, and Cefuroxime. Rumex dentatus leaves extract in methanolic concentrations was used to control the identified strain and it demonstrated potent antibacterial activity. Interconnection between extract concentration and brine shrimp fatality is dependent on the cytotoxicity, indicating that at 200 µg/mL, brine shrimp mortality was greater than at other therapeutic doses, and the antioxidant test results showed that when it compared to the BHT control the leaf extract showed remarkable antioxidant properties. In molecular docking studies, the maximum target binding attractions for the strains were obtained for 1-Alpha-18O-1,25-dihydroxycholecalciferol, Androstan-3-ol, 9-methyl-(3 beta,5 alpha), Phenylacetaldehyde, Benzenepropanoic acid, 3,5-bis(1,1-dimethylethyl)-4-hydroxy-methyl ester, and this research could help future researchers to find an effective treatment for Staphylococcus aureus infections. More research on the safety characteristics of potential Rumex dentatus ingredients is required.
ACKNOWLEDGEMENT
None
AUTHOR CONTRIBUTIONS
MMZ: Experiment, conceptualizations, validations, data analysis; MMM: Experiment, conceptualizations, SI: Experiments, writing, editing, MMJ: Writing, data curation; JB: Writing, data curation; SB: validations, data analysis; AI: Data curation, data analysis; SZ: Review, editing; MAS: Review, editing, supervision; MSU: Conceptualization, editing, supervision, revision.
CONFLICTS OF INTEREST
There is no conflict of interest among the authors.
References
- [1]Nedorost ST. Generalized dermatitis in clinical practice. Springer Science & Business Media; 2012 Jul 6.
- [2]Laborel-Préneron E, Bianchi P, Boralevi F, Lehours P, Fraysse F, Morice-Picard F, et al. Effects of the Staphylococcus aureus and Staphylococcus epidermidis secretomes isolated from the skin microbiota of atopic children on CD4+ T cell activation. PLoS One. 2015 Oct 28;10(10):e0141067.
- [3]Rasmussen RV, Fowler Jr VG, Skov R, Bruun NE. Future challenges and treatment of Staphylococcus aureus bacteremia with emphasis on MRSA. Future microbiology. 2011 Jan;6(1):43-56.
- [4]Nascimento GG, Locatelli J, Freitas PC, Silva GL. Antibacterial activity of plant extracts and phytochemicals on antibiotic-resistant bacteria. Brazilian journal of microbiology. 2000;31:247-56.
- [5]Santos FD, Sarti SJ, Bastos JK, Leitão Filho HF, Machado JO, Araujo ML, et al. Atividade antibacteriana de extratos vegetais. Revista de Ciências Farmacêuticas, 1990; 12, 47-69.”.
- [6]Amenu D. Antimicrobial activity of medicinal plant extracts and their synergistic effect on some selected pathogens. American Journal of Ethnomedicine. 2014;1(1):18-29.
- [7]Bhavsar AP, Guttman JA, Finlay BB. Manipulation of host-cell pathways by bacterial pathogens. Nature. 2007 Oct;449(7164):827-34.
- [8]Tong SY, Davis JS, Eichenberger E, Holland TL, Fowler Jr VG. Staphylococcus aureus infections: epidemiology, pathophysiology, clinical manifestations, and management. Clinical microbiology reviews. 2015 Jul;28(3):603-61.
- [9]Batool R, Aziz E, Tan BK, Mahmood T. Rumex dentatus inhibits cell proliferation, arrests cell cycle, and induces apoptosis in MDA-MB-231 cells through suppression of the NF-κB pathway. Frontiers in pharmacology. 2017 Oct 12;8:731.
- [10]Orhan I, Deliorman-Orhan D, Özçelik B. Antiviral activity and cytotoxicity of the lipophilic extracts of various edible plants and their fatty acids. Food chemistry. 2009 Jul 15;115(2):701-5.
- [11]Hussain F, Islam M, Zaman A. Ethnobotanical profile of plants of Shawar valley, district Swat, Pakistan. Int J Biol Biotechnol. 2006;3(2):301-7.
- [12]Fatima N, Zia M, Rehman R, Rizvi ZF, Ahmad S, Mirza B, et al. Biological activities of Rumex dentatus L: Evaluation of methanol and hexane extracts. African Journal of Biotechnology. 2009;8(24).
- [13]Santos PR, Oliveira AC, Tomassini TC. Controle microbiológico de produtos fitoterápicos. Rev. farm. bioquim. Univ. Säo Paulo. 1995:35-8.
- [14]Janssen AM, Scheffer JJ, Svendsen AB. Antimicrobial activity of essential oils: a 1976-1986 literature review. Aspects of the test methods. Planta medica. 1987 Oct;53(05):395-8.
- [15]Lemos TL, Monte FJ, Matos FJ, Alencar JW, Craveiro AA, Barbosa RC, et al. Chemical composition and antimicrobial activity of essential oils from Brazilian plants. Fitoterapia. 1992;63(3):266-8.
- [16]Stach JE, Bathe S, Clapp JP, Burns RG. PCR-SSCP comparison of 16S rDNA sequence diversity in soil DNA obtained using different isolation and purification methods. FEMS Microbiology Ecology. 2001 Jul 1;36(2-3):139-51.
- [17]Mariswamy Y, Gnaraj WE, Johnson M. Chromatographic finger print analysis of steroids in Aerva lanata L by HPTLC technique. Asian Pacific journal of tropical biomedicine. 2011 Dec 1;1(6):428-33.
- [18]Nostro A, Germano MP, D’angelo V, Marino A, Cannatelli MA. Extraction methods and bioautography for evaluation of medicinal plant antimicrobial activity. Letters in applied microbiology. 2000 May;30(5):379-84.
- [19]Meyer BN, Ferrigni NR, Putnam JE, Jacobsen LB, Nichols DE, McLaughlin JL. Brine shrimp: a convenient general bioassay for active plant constituents. Planta medica. 1982 May;45(05):31-4.
- [20]Rahman M, Islam M, Biswas M, Khurshid Alam AH. In vitro antioxidant and free radical scavenging activity of different parts of Tabebuia pallida growing in Bangladesh. BMC research notes. 2015 Dec;8(1):1-9.
- [21]Jahan I, Tona MR, Sharmin S, Sayeed MA, Tania FZ, Paul A, et al. GC-MS phytochemical profiling, pharmacological properties, and in silico studies of Chukrasia velutina leaves: A novel source for bioactive agents. Molecules. 2020 Aug 2;25(15):3536.
- [22]Mahmud S, Paul GK, Afroze M, Islam S, Gupt SB, Razu MH, et al. Efficacy of phytochemicals derived from Avicennia officinalis for the management of COVID-19: a combined in silico and biochemical study. Molecules. 2021a Apr 12;26(8):2210.
- [23]Bauer AW, Kirby WMN, Sherris JC, “Antibiotic Susceptibility Testing by a Standardized Single Disc Method. Am J Clin Pathol. 1996; no. 45, pp. 493–49.
- [24]Kumar V, Sharma A, Thukral AK, Bhardwaj R. Phytochemical Profiling of Methanolic extracts of medicinal plants using GC-MS. International Journal of Research and Development in Pharmacy and Life Sciences. 2016;5(3):2153-8.
- [25]Rahman M, Ahad A, Saha SK, Hong J, Kim KH. Antibacterial and phytochemical properties of Aphanamixis polystachya essential oil. 분석과학. 2017;30(3).
- [26]Wang GW, Jin HZ, Zhang WD. Constituents from Aphanamixis species and their biological activities. Phytochemistry reviews. 2013 Dec;12(4):915-42.
- [27]Kim S, Thiessen PA, Bolton EE, Chen J, Fu G, Gindulyte A, et al. PubChem substance and compound databases. Nucleic acids research. 2016 Jan 4;44(D1):D1202-13.
- [28]Goodsell DS, Morris GM, Olson AJ. Automated docking of flexible ligands: applications of AutoDock. Journal of molecular recognition. 1996 Jan;9(1):1-5.
- [29]Trott O, Olson AJ. AutoDock Vina: improving the speed and accuracy of docking with a new scoring function, efficient optimization, and multithreading. Journal of computational chemistry. 2010 Jan 30;31(2):455-61.
- [30]DeLano WL. Pymol: An open-source molecular graphics tool. CCP4 Newsl. Protein Crystallogr. 2002 Mar;40(1):82-92.
- [31]Accelrys Software Inc., Discovery Studio Modeling Environment, Release 3.0, San Diego: Accelrys Software Inc.,” p. 2007, 2005, [Online]. http://www.accelrys.com.
- [32]Hosseinzadeh S, Jafarikukhdan A, Hosseini A, Armand R. The application of medicinal plants in traditional and modern medicine: a review of Thymus vulgaris. International Journal of Clinical Medicine. 2015;6(09):635.
- [33]Silva NC, Fernandes Júnior AJ. Biological properties of medicinal plants: a review of their antimicrobial activity. Journal of venomous animals and toxins including tropical diseases. 2010;16:402-13.
- [34]Kebede T, Gadisa E, Tufa A. Antimicrobial activities evaluation and phytochemical screening of some selected medicinal plants: A possible alternative in the treatment of multidrug-resistant microbes. PLoS One. 2021 Mar 26;16(3):e0249253.
- [35]Zhang A, Sun H, Wang X. Recent advances in natural products from plants for treatment of liver diseases. European journal of medicinal chemistry. 2013 May 1;63:570-7.
- [36]Paul GK, Mahmud S, Hasan MM, Zaman S, Uddin MS, Saleh MA. Biochemical and in silico study of leaf and bark extracts from Aphanamixis polystachya against common pathogenic bacteria. Saudi journal of biological sciences. 2021 Nov 1;28(11):6592-605.
- [37]Yadav RN, Agarwala M. Phytochemical analysis of some medicinal plants. Journal of phytology. 2011 Dec 14;3(12).
- [38]ingh R, Singh S, Kumar S, Arora S. Evaluation of antioxidant potential of ethyl acetate extract/fractions of Acacia auriculiformis A. Cunn. Food and chemical toxicology. 2007 Jul 1;45(7):1216-23.
- [39]Han X, Shen T, Lou H. Dietary polyphenols and their biological significance. International journal of molecular sciences. 2007 Sep 12;8(9):950-88.
- [40]Humeera N, Kamili AN, Bandh SA, Lone BA, Gousia N. Antimicrobial and antioxidant activities of alcoholic extracts of Rumex dentatus L. Microbial pathogenesis. 2013 Apr 1;57:17-20.
- [41]Elzaawely AA, Tawata S. Antioxidant capacity and phenolic content of Rumex dentatus L. grown in Egypt. Journal of Crop Science and Biotechnology. 2012 Mar;15(1):59-64.
- [42]Brown JE, Rice-Evans CA. Luteolin-rich artichoke extract protects low density lipoprotein from oxidation in vitro. Free radical research. 1998 Jan 1;29(3):247-55.
- [43]Krings U, Berger RG. Antioxidant activity of some roasted foods. Food chemistry. 2001 Feb 1;72(2):223-9.
- [44]Siddhuraju P, Mohan PS, Becker K. Studies on the antioxidant activity of Indian Laburnum (Cassia fistula L.): a preliminary assessment of crude extracts from stem bark, leaves, flowers and fruit pulp. Food chemistry. 2002 Oct 1;79(1):61-7.
- [45]Jayasri MA, Lazar M, Radha A. A report on the antioxidant activity of leaves and rhizomes of Costus pictus D. Don. International Journal of Integrative Biology. 2009;5(1):20-6.
- [46]Bonvin AM. Flexible protein–protein docking. Current opinion in structural biology. 2006 Apr 1;16(2):194-200.
- [47]Silakari O, Singh PK. Concepts and Experimental Protocols of Modelling and Informatics in Drug Design. Academic Press; 2020 Nov 5.
- [48]Naher K, Moniruzzaman M, Islam S, Hasan A, Paul GK, Jabin T, et al. Evaluation of biological activity and in silico molecular docking studies of Acanthus ilicifolius leaf extract against four multidrug-resistant bacteria. Informatics in Medicine Unlocked. 2022 Jan 1;33:101092. doi: 10.1016/j.imu.2022.101092.
- [49]Mahmud S, Uddin MA, Zaman M, Sujon KM, Rahman ME, Shehab MN, et al. Molecular docking and dynamics study of natural compound for potential inhibition of main protease of SARS-CoV-2. Journal of Biomolecular Structure and Dynamics. 2021b Nov 2;39(16):6281-9.
- [50]Eissa IH, Ibrahim MK, Metwaly AM, Belal A, Mehany AB, Abdelhady AA, et al. Design, molecular docking, in vitro, and in vivo studies of new quinazolin-4 (3H)-ones as VEGFR-2 inhibitors with potential activity against hepatocellular carcinoma. Bioorganic Chemistry. 2021 Feb 1;107:104532.
- [51]Rahman M, Browne JJ, Van Crugten J, Hasan MF, Liu L, Barkla BJ. In silico, molecular docking and in vitro antimicrobial activity of the major rapeseed seed storage proteins. Frontiers in pharmacology. 2020 Sep 8;11:1340.
- [52]Ying TC, Ibrahim Z, Abd Rahman MB, Tejo BA. Structure-based design of peptide inhibitors for protein arginine deiminase type iv (PAD4); 2019.
- [53]Prabhu S, Ignatova A, Park ST, Sun XH. Regulation of the expression of cyclin-dependent kinase inhibitor p21 by E2A and Id proteins. Molecular and cellular biology. 1997 Oct;17(10):5888-96.
- [54]Bearman GM, Wenzel RP. Bacteremias: a leading cause of death. Archives of medical research. 2005 Nov 1;36(6):646-59.
- [55]Moniruzzaman M, Jinnah MM, Islam S, Biswas J, Pramanik MJ, Uddin MS, et al. Biological activity of Cucurbita maxima and Momordica charantia seed extracts against the biofilm-associated protein of Staphylococcus aureus: An in vitro and in silico studies. Informatics in Medicine Unlocked. 2022 Jan 1;33:101089.
- [56]Jahantighi A, Kiani G, Moghaddam TT, Ghahari S. In vitro antibacterial activity of selected medicinal plants traditionally used in Iran against plant and human pathogenic bacteria. Jordan Journal of Biological Sciences. 2016 Sep;9(3):221-6.
- [57]Thompson MK, Keithly ME, Goodman MC, Hammer ND, Cook PD, Jagessar KL, et al. Structure and function of the genomically encoded fosfomycin resistance enzyme, FosB, from Staphylococcus aureus. Biochemistry. 2014 Feb 4;53(4):755-65.
- [58]Kumar V, Sharma A, Thukral AK, Bhardwaj R. Amino acid profiling of the leaves of plants in the vicinity of river Beas, India. Journal of Chemical and Pharmaceutical Research. 2015;7(11):504-10.
- [59]Elbermawi A, Darwish MS, El-Awady AA, Zaki AA, Qiu L, Samra RM. Isolation and biological activities of compounds from Rumex vesicarius L. and their use as a component of a synbiotic preparation. Food chemistry: X. 2022 Jun 30;14:100306.
- [60]Shen J, Cheng F, Xu Y, Li W, Tang Y. Estimation of ADME properties with substructure pattern recognition. Journal of chemical information and modeling. 2010 Jun 28;50(6):1034-41.
- [61]Sanguinetti MC, Tristani-Firouzi M. hERG potassium channels and cardiac arrhythmia. Nature. 2006 Mar;440(7083):463-9.